LncRNA OXCT1-AS1 promotes the proliferation of non-small cell lung cancer cells by targeting the miR-195/CCNE1 axis
Introduction
Lung cancer (LC) is a common malignant tumor with high rates of morbidity and mortality. In fact, it has the highest mortality among various types of malignant tumors, which poses a great threat to human life and health (1). Among the traditional LC treatments, surgical treatment has been widely used. However, most patients with LC have atypical clinical manifestations in the early stage of the disease and clinical diagnostic techniques are limited at present, based on the fact that 75% of patients with LC are diagnosed with regional metastasis at the first visit. These patients’ cancers spread to regional lymph nodes or directly infiltrate the surrounding normal tissues, resulting in a significant reduction in the 5-year survival rate (only 20.6%) (2,3). At present, the overall 5-year survival rate of LC patients is still at a low level (only about 15%), which is due to the fact that most patients are in the advanced stage when they are first diagnosed and have poor response to chemoradiotherapy (4).
Clinically, non-small cell lung cancer (NSCLC) accounts for 85% of all LC cases. The main causes of LC development are mutation or inactivation of tumor suppressor genes as well as activation of oncogenes (5). Other biological pathways may also have an important impact on the prognosis of NSCLC patients and the sensitivity of NSCLC therapeutics (6). Therefore, studying the incidence of NSCLC and its malignant mechanism contributes to a deeper understanding of the disease, which is of great importance for the formulation of individualized treatment, and also provides an effective criteria for prognosis.
Long non-coding RNAs (lncRNAs) are a series of transcripts with a length of more than 200 nucleotides (7). Recently, a large number of lncRNAs have been found to be aberrantly expressed in tumors (8). Although lncRNAs have been considered as “noise” in the genome, increasing evidence has suggested that they play an essential role in biological and pathological functions, such as cell proliferation, invasion, and apoptosis (9). A previous study has reported that lncRNAs act as competitive endogenous RNAs (ceRNAs) or microRNA (miRNA) “sponges” binding to miRNAs without degradation, thereby affecting downstream gene expression (10). It has been shown that lncRNA-OXCT1-AS1 is lowly expressed in various tumors and is involved in the proliferation and metastasis of tumor cells (11). In glioma, lncRNA-OXCT1-AS1 acts as a ceRNA against miR-195 by increasing the expression of CDC25A and promoting the growth of cancer cells (12). The lncRNA, LINC00355, increases the expression of CCNE1 by downregulating miR-195, and promotes the proliferation of lung adenocarcinoma (LUAD) cells (13). The lncRNA, SNHG12, affects the activity, apoptosis, and autophagy of prostate cancer cells by regulating miR-195/CCNE1 (14). A study has shown that lncRNAs/miRNAs/target genes play an important role in NSCLC (15). The lncRNA-OXCT1-AS1 promotes the metastasis of NSCLC in vitro and in vivo by stabilizing LEF1. Therefore, we constructed a presumptive lncRNA-OXCT1-AS1/miR-195/CCNE1 network to elucidate the underlying mechanisms of the pathogenesis and progression of NSCLC. This study found that lnRNA OXCT1-AS1 can regulate the proliferation ability of NSCLC through miR-195/CCNE1 signaling, which aiming to provide clues for the identification of new diagnostic markers or therapeutic targets for NSCLC. We present the following article in accordance with the MDAR reporting checklist (available at https://tcr.amegroups.com/article/view/10.21037/tcr-22-855/rc).
Methods
Research object
Tumor samples and adjacent tissues from eight NSCLC patients were collected for this study, none of whom had received radiotherapy or chemotherapy before surgery. The tissue specimens included primary NSCLC and adjacent tissues (>5 cm from the primary tumor, and pathologically confirmed no tumor cell infiltration). After resection, the specimens were flash frozen in liquid nitrogen and then transferred to a −80 ℃ freezer for further experimentation. The study was conducted in accordance with the Declaration of Helsinki (as revised in 2013). The study was approved by institutional ethics board of The Third Affiliated Hospital of Qiqihar Medical University (No. 2021LL-9) and informed consent was taken from all the patients.
Cell lines and cell culture
Human LC cell lines (A549, NCI-H1299, and H1650) and normal human lung epithelial cells (BEAS-2B) were purchased from the Cell Bank of the Chinese Academy of Sciences (Shanghai, China). The cells were cultured with RPMI-1640 (HyClone, USA), containing 10% (v/v) fetal bovine serum (FBS, Gibco, USA), 1% penicillin/streptomycin (Sigma, Guangzhou, China), and placed in a cell incubator with 5% CO2 at 37 ℃.
RNA extraction and quantitative reverse transcription polymerase chain reaction (qRT-PCR)
Total RNA was extracted from NSCLC tissues and cells using TRIzol reagent (Invitrogen, Carlsbad, CA, USA). RNA was amplified to complementary DNA (cDNA) using the TapMan-MiRNA Reverse Transcription Kit (Invitrogen, Carlsbad, USA) with reverse transcription at 16 ℃ for 30 min, 42 ℃ for 30 min, and 85 ℃ for 5 min under reaction conditions. qRT-PCR amplification was performed using SYBR Green (Qiagen, USA), under the following reaction conditions: 40 cycles of amplification at 95 ℃ for 5 min, 95 ℃ for 15 s, and 6 ℃ for 30 s. The resulting values were normalized to the internal control glyceraldehyde-3-phosphate dehydrogenase (GAPDH) and U6 for each replicate. The qRT-PCR primers are shown in Table 1.
Table 1
Gene name | Forward 5'-3' | Reverse 5'-3' |
---|---|---|
CCNE1 | 5'-TGACCTAAGGGACTCCCACAA-3' | 5'-TGATATGTGGAGAGGGCAGC-3' |
CCND1 | 5'-TGGTGAACAAGCTCAAGTGGA-3' | 5'-AGGGCGGTTGGAAATGAACT-3' |
MiR-195 | 5'-ACACTCCAGCTGGGTAGCAGCACAGAAAT-3' | 5'-TGGTGTCGTGGAGTCG-3' |
U6 | 5'-CTCGCTTCGGGCAGCACA-3' | 5'-AACGCTTCAGGAATTTGCGT-3' |
GAPDH | 5'-AAGTTCAACGGCACAGTCAA-3' | 5'-TACTCACCAGCATCACC-3' |
RT-PCR, reverse transcription polymerase chain reaction; GAPDH, glyceraldehyde-3-phosphate dehydrogenase.
For miRNA expression analysis, RNA (100 ng) was reverse transcribed using TaqMan Advanced MicroRNA Assay kit (Applied Biosystems, Thermo Fisher Scientific, China) and miRNA-specific primers. The primers were synthesized by Shanghai GenePharma Co., Ltd. (Shanghai, China). The cycle threshold (Ct) value was recorded, and the relative expression level was calculated using the 2−ΔΔCt. All experiments were repeated three times.
Western blot
Cells from each group were collected, lysed by using RIPA buffer, and then centrifuged at 12,000 rpm for 10 minutes at 4 ℃. The supernatant was collected and the protein concentration in each group was quantified using the bicinchoninic acid (BCA) assay, and then separated by 12% polyacrylamide sodium dodecyl sulfate (SDS) gel electrophoresis and transferred to polyvinylidene fluoride (PVDF) membranes. Blocking was then performed using 5% non-fat dry milk for 1 h, followed by subsequent incubation with the primary antibody, CCNE1 (1:1,000, CST, Boston, USA), overnight at 4 ℃. The horseradish peroxidase-conjugated secondary antibody (1:1,000) was then incubated for 1 h. Signals were visualized by using enhanced chemiluminescence detection (BeyoECL Plus, Catalog No. P0018M; Beyotime Institute of Biotechnology). The ImageLabTM software system (Bio-Rad, USA) used to conduct quantitative analysis of the proteins in each group.
Cell transfection
si-OXCT1-AS1, si-NC, and miR-195 mimic, NC-mimics, pcDNA3.1 (+) vector, and pcDNA3.1-CCNE1 vector were designed and synthesized by Gene Pharma (Shanghai, China). The cells were seeded in six-well plates. Lipofectamine 2000 (Invitrogen, Carlsbad, USA) was used for transfection at 70% condensity according to the manufacturer’s instructions. Forty-eight hours after transfection, the cells were harvested for further in vitro experiments.
Luciferase reporter assay
OXCT1-AS1 and CCNE1 3' untranslated regions (3' UTRs) fragments containing the miR-195 binding site were amplified and cloned into the psiCheck2 reporter vector (Promega, Shanghai, China). Next, the wild-type or mutant plasmid, pCheck2 plasmid, and an equal amount of negative control or miR-195 mimic plasmid were co-transfected into the H1650 cells. Luciferase assays were performed using a dual luciferase reporter assay system according to the manufacturer’s instructions (PROMEGA).
Cell proliferation assay
Cells at 10,000 cells/well were seeded in 96-well plates and placed in a cell incubator (Thermo Fisher Scientific, China) for culture. According to the manufacturer’s instructions, cell proliferation was detected using a Cell Counting Kit-8 (CCK-8) at 0, 24, 48, and 72 h, respectively. Ten µL of CCK-8 reagent was added to each well, and after incubation for 1 h, the optical density (OD) value at 490 nm was record using a microplate reader (Shenzhen Mepco Technology Co., Ltd., Shenzhen, China). All experiments were repeated three times.
Colony forming assay
H16550 cells (100 cells/well) were used for the colony forming assay. One hundred cells were seeded in six-well culture plates. Visible colonies were fixed after 10–14 days and stained with 0.1% crystal violet in 20% methanol. All experiments were repeated three times
Target prediction
Bioinformatics analysis was performed by using the following bioinformatics tools: LncBase Predicted V2.0 (https://diana.e-ce.uth.gr/lncbasev3/expression), miRDB (http://mirdb.org/), TargetScan (https://www.targetscan.org/vert_71/), and miRTarBase (https://mirtarbase.cuhk.edu.cn/~miRTarBase/miRTarBase_2022/php/index.php).
Survival analysis of CCNE1 in LUAD
In this study, high- and low-expression groups were cut off using the median expression value of CCNE1. Prognostic differences between different CCNE1 expression groups were compared using Kaplan-Meier curves with the log-rank test. A time-dependent receiver operating characteristic (ROC) curve was used to evaluate the accuracy of CCNE1 expression in predicting the prognosis of LUAD, which was performed by survival package in the R platform (https://cran.r-project.org/web/packages/ROCR/index.html) (12,16).
Statistical analysis
Data are presented as the mean ± standard error of the mean (SEM). Group comparisons were made using the Student’s t-test or analysis of variance (ANOVA). P<0.05 was considered statistically significant. Statistical analyses were performed using Graph Pad Prism 8.0 (http://graphpadchina.com/).
Results
Different expression patterns of lncRNA OXCT1-AS1 and miR-195 in NSCLC
First, qRT-PCR was used to detect the mRNA expression levels of OXCT1-AS1 and miR-195 in eight pairs of NSCLC tissues and adjacent tissue samples. The results showed that the expression levels of OXCT1-AS1 were significantly increased, whereas miR-195 was significantly decreased in cancer tissues (as shown in Figure 1A,1B; P<0.001).
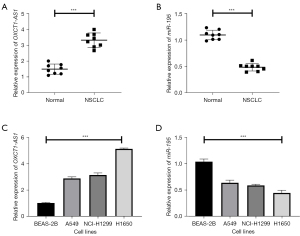
Furthermore, qRT-PCR was used to detect the mRNA expression levels of OXCT1-AS1 and miR-195 in BEAS-2B and NSCLC cell lines (A549, NCI-H1299, and H1650). Similarly, the results showed that OXCT1-AS1 were significantly increased, coupled with markedly decreased levels of miR-195 in NSCLC cell lines (Figure 1C,1D; P<0.001). The above results indicate that lncRNA OXCT1-AS1 is highly expressed and miR-195 is lowly expressed in NSCLC tissues and cell lines.
LncRNA OXCT1-AS1 targets and inhibits the expression of miR-195 in NSCLC cell lines
Next, we explored the regulatory relationship between OXCT1-AS1 and miR-195. As shown in Figure 2A, OXCT1-AS1 obtained a high-scoring binding site with miR-195 through the online database software, LncBaseV2.0 (https://starbase.sysu.edu.cn/starbase2/index.php). Given that OXTC1-AS1 was expressed at the highest levels in H1650 cells, we then selected H1650 cells as the research objects in subsequent experiments. After transfection (si-NC, si-OXTC1-AS1), changes in miR-195 were detected using qRT-PCR. As shown in Figure 2B, the si-OXCT1-AS1-transfected H1650 cells successfully knocked down the expression of OXCT1-AS1 (***P<0.001). Also, the detection of miR-195 expression by qRT-PCR revealed that knockdown of OXTC1-AS1 significantly increased the expression level of miR-195 compared with the si-NC group (Figure 2C; ***P<0.001).
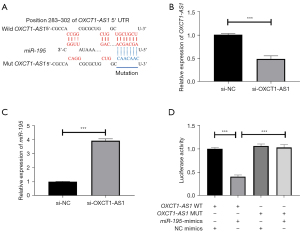
Furthermore, luciferase reporter analysis determined the regulatory relationship between OXTC1-AS1 and miR-195. The experimental groups, GLO-OXTC1-AS1-WT and GLO-OXTC1-AS1-MUT, were co-transfected with NC-mimics or miR-195 mimics, respectively, and the results showed that compared with NC mimics, luciferase activity was significantly reduced in the GLO-OXTC1-AS1-AS1-WT group after transfection with miR-195 mimics (Figure 2D). The above results indicated that lncRNA OXCT1-AS1 targeted and inhibited the expression of miR-195 in NSCLC cell lines.
LncRNA OXCT1-AS1 promotes the proliferation of NSCLC cell lines
To further explore the biological function of OXCT1-AS1 in NSCLC, we transfected si-NC and si-OXTC1-AS1, respectively, into H1650 cells. Changes in cell growth curves were analyzed using the CCK-8 assay. Knockdown of OXTC1-AS1 significantly inhibited the proliferative capacity of cells (Figure 3A). The results of colony forming assay and EdU assay showed that knockdown of OXTC1-AS1 markedly suppressed the colony forming ability and proliferation ability of cells (Figure 3B,3C).
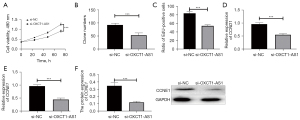
Cyclins also play an important role in the biological process of cell proliferation. Therefore, the effect of OXCT1-AS1 on the expression levels of cyclins (CCND1 and CCNE1) was examined by qRT-PCR. The results showed that knockdown of OXTC-AS1 considerably downregulated the expression of CCND1 and CCNE1 compared with si-NC group, as shown in Figure 3D,3E (P<0.001). Western blotting results indicated that knockdown of OXTC-AS1 significantly inhibited the protein expression of CCNE1 compared with the si-NC group (Figure 3F). The above results indicated that knockdown of OXCT1-AS1 inhibited cell proliferation by inhibiting the expression of cyclins (CCND1 and CCNE1) in NSCLC cells. In addition, a previous study found that knockdown of lncRNA OXCT1-AS1 inhibited tumor growth in mice (16). This result can further support our findings at the cellular level in vitro.
In NSCLC cell lines, knockdown of lncRNA OXCT1-AS1 inhibited cell proliferation by upregulating miR-195
Next, we transfected si-NC, si-OXCT1-AS1, si-OXCT1-AS1 + miR-195 mimics, respectively, into H1650 cells, and the changes in cell growth curves were analyzed using CCK-8. The si-OXCT1-AS1 + miR-195 mimics group more significantly inhibited the proliferation of cells compared with the si-OXCT1-AS1 group (Figure 4A). The results of the colony forming assay also showed that the clome numbers were more significantly inhibited in the si-OXCT1-AS1 + miR-195 mimics group compared with the si-OXCT1-AS1 group (Figure 4B). Additionally, qRT-PCR assay indicated that the expressions of CCND1 and CCNE1 were more significantly inhibited in the si-OXCT1-AS1 + miR-195 mimics group compared with the si-OXCT1-AS1 group (Figure 4C). Western blotting results indicated that CCNE1 protein expression was remarkably inhibited in the si-OXCT1-AS1 + miR-195 mimics group compared with the si-OXCT1-AS1 group (Figure 4D,4E). The above results indicated that knockdown of lncRNA OXCT1-AS1 inhibited cell proliferation by upregulating miR-195 in NSCLC cells.
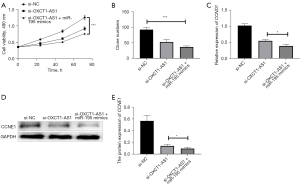
MiR-195 targets and regulates CCNE1 expression in NSCLC cell lines
Generally, the biological function of miRNAs is achieved through their downstream target genes. Thus, the downstream target genes of miR-195 were searched and analyzed. As shown in Figure 5A, miR-195 binds to the 3'-UTR of CCNE1. To verify this binding, miR-195 mimics was successfully transfected into H1650 cells to achieve its overexpression (Figure 5B; ***P<0.001). Detection of CCNE1 expression by qRT-PCR revealed that the miR-195 mimics group significantly inhibited the mRNA expression level of CCNE1 compared with the NC mimics group (Figure 5C; ***P<0.001).
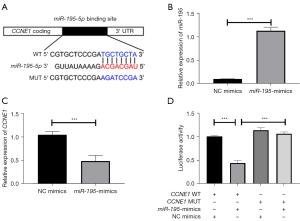
Also, luciferase reporter assay was performed to determine the regulatory relationship between miR-195 and CCNE1. The experimental groups, CCNE1-WT and CCNE1-MUT, were co-transfected with NC-mimics or miR-195 mimics, respectively. The results showed that in CCNE1-WT group, luciferase activity was significantly reduced after transfection with miR-195 mimics compared with NC (Figure 5D). The above findings indicated that miR-195 directly targeted the expression of CCNE1 in NSCLC cell lines.
Survival analysis of CCNE1 in LUAD
Survival analysis was performed in different CCNE1 expression groups for LUAD in The Cancer Genome Atlas (TCGA) datasets. Patients with high CCNE1 expression had a shorter median survival time compared with patients with low CCNE1 expression (high CCNE1 vs. low CCNE1 =580 vs. 645.5 days; Figure 6A). We observed that high CCNE1 expression was notably associated with an unfavorable prognosis in LUAD as well as an increased risk of cancer-related death of LUAD patients {P<0.01; hazard ratio (HR) =1.48 [95% confidence interval (CI): 1.1–1.99]; Figure 6B}. The time-dependent ROC curve indicated that the expression level of CCNE1 gene had a certain prognostic value in the long-term survival of patients with LUAD. The area under the ROC curve for the 1-, 3-, and 5-year survival were 0.592, 0.59, and 0.62, respectively (Figure 6C).
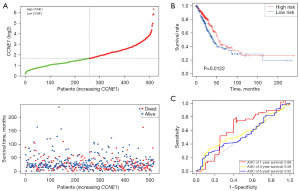
We then transfected pcDNA3.1 vector or pcDNA3.1-CCNE1, respectively, into H1650 cells, and the transfection efficiency was verified by qRT-PCR. The experimental results showed a successful overexpression of CCNE1 in H1650 cells (Figure 7A). Changes in cell growth curves and colony formation were analyzed, and were found to be significantly increased in the si-OXCT1-AS1 + pcDNA3.1-CCNE1 group compared with si-OXCT1-AS1 + pcDNA3.1 vector group (Figure 7B,7C). Overexpression of CCNE1 restored the cell proliferation against the knockdown of OXTC1-AS1. Indeed, the expression level of CCNE1 was significantly increased in si-OXCT1-AS1 + pcDNA3.1-CCNE1 group compared with si-OXCT1-AS1 group (Figure 7D-7F). Therefore, we surmised that overexpression of CCNE1 could reverse the proliferation against lncRNA OXCT1-AS1 knockdown in NSCLC cells.
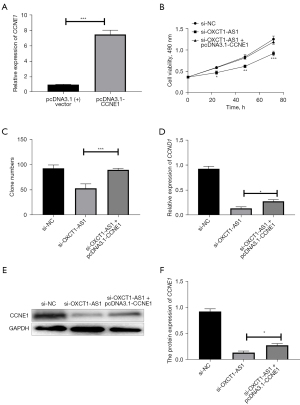
Discussion
LC is a common malignant tumor, with the highest rates of morbidity and mortality worldwide. In China, the peak morbidity and mortality has never declined. In 2012, there were about 326,600 new cases of LC and 56,400 deaths (17). A previous study has shown that NSCLC accounts for 85% of all LC cases. The biological characteristics of NSCLC are invasion and metastasis, which is also the leading cause of treatment failure and the high mortality rate (18). Due to the lack of effective therapeutic targets, the survival rate of cell LC is still decreasing year by year (19). Therefore, it is essential to find novel and effective therapeutic targets.
LncRNAs have been found to be aberrantly expressed during tumor development and progression, and are expected to be potential biomarkers and therapeutic targets. A recent study has found that aberrantly-expressed lncRNAs play important biological roles in various tumors, and are expected to be potential biomarkers and therapeutic targets (20). For example, lncRNA LINC00899 blocks the progression of breast cancer by inhibiting miR-425 (21), and lncRNA ZFAS1 regulates the development of esophageal squamous cell carcinoma via the miR124/STAT3 axis (22). Also, the lncRNA, MALAT1, promotes glioblastoma multiforme (GBM) proliferation and progression by targeting the miR-199a/ZHX1 axis (23). A recent study has shown that the lncRNA, AC079630, is associated with prognosis as a tumor suppressor in LC (24). Furthermore, m6A transferase METTL3-induced lncRNA ABHD11-AS1 promotes the Warburg effect in NSCLC (25). The above studies highlight the crucial role played by lncRNAs in the diagnosis and treatment of human cancers.
At present, it has been demonstrated that lncRNA OXCT1-AS1 (antisense RNA1 of OXCT1) is a ceRNA in human glioblastoma; it is upregulated in tumor tissues, and its high expression is associated with poor prognosis (11). Inhibition of OXCT1-AS1 expression significantly inhibits cell proliferation, migration, and invasion, and its mechanism of action potentially involves OXCT1-AS1 acting as a ceRNA of miR-195 to enhance the expression of CDC25A, thereby promoting the progression of glioma cells (11). It has also been shown that lncRNA OXCT1-AS1 expression is significantly increased in bladder cancer patients with lymph nodes metastasis, and the lncRNA, OXCT1-AS, reduces binding to the target gene, JAK1, by inhibiting miR-455-5p. This in turn upregulates the expression of JAK1, which promotes the proliferation and metastasis of bladder cancer (11). Thus, the lncRNA, OXCT1-AS1, can be used as a potential biomarker and therapeutic target in patients with human glioblastoma and bladder cancer.
A recent study has shown that the lncRNA, OXCT1-AS1, promotes the metastasis of NSCLC in vitro and in vivo by stabilizing LEF1 (26). In this study, we found that lncRNA OXCT1-AS1 expression was elevated and miR-195 expression was decreased in NSCLC tissues and cells. In NSCLC cell lines, knockdown of OXCT1-AS1 expression significantly inhibited cell viability and proliferation, together with the reduction of CCND1 and CCNE1. In contrast, overexpression of miR-195 significantly decreased cell viability and proliferation, and inhibited the expression of cyclins, CCND1 and CCNE1. Meanwhile, we found that knockdown of OXCT1-AS1 could significantly inhibit miR-195 expression, and the luciferase reporter assay further verified that OXCT1-AS1 targets and regulates miR-195 expression. It has been reported that reduced miR-195 expression in NSCLC promotes aspects of cancer progression, such as cancer cell proliferation, which is consistent with our findings. Taken together, our data suggested that OXCT1-AS1 acts as an oncogene in NSCLC.
A study has reported that miR-195 is abnormally expressed in a variety of malignancies, including NSCLC (25). As a tumor suppressor, miR-195 is involved in various cell functions such as cell cycle regulation, DNA damage and repair, gene transcription, migration, invasion, and tumor cell apoptosis (26,27). According to a previous study, the downstream target genes of miR-195 include CCNE1, BIRC5, IGF1R, and CCND3 (28). In addition, increased miR-195 expression levels were reportedly associated with decreased survival rates in NSCLC (29). In our study, we hypothesized that miR-195 inhibits the growth of NSCLC cells by targeting downstream genes. To further investigate the underlying mechanism, potential targets of miR-195 were explored and CCNE1 was selected due to its tight association with cell proliferation. We found that CCNE1 served as a direct target of miR-195.
Cyclins are involved in cell cycle regulation, and interact with cyclin-dependent kinases (CDKs) and cell cycle-dependent kinase inhibitors (CKIs) (30,31). CCNE1 is an isoform of cyclin E. The CCNE1-CKD2 complex induces hyperphosphorylated cyclin D-CDK4/6 to trigger Rb protein phosphorylation (32), releasing E2F, which allows cell cycle progression G1 to S phase and DNA synthesis (33). Some studies have illustrated the abnormal expression of CCNE1 in a variety of malignant cells (34,35). It has been shown that overexpression of CCNE1 is associated with poor prognosis in NSCLC (36). The lncRNA, SENP3-EIF4A1, acts as a sponge for miR-195-5p and drives the progression of triple-negative breast cancer by overexpressing CCNE1 (37). Also, the lncRNA, LINC00355, increases the expression of CCNE1 by downregulating miR-195, thereby promoting the proliferation of LUAD cells (12). Additionally, the lncRNA, SNHG12, affects the activity, apoptosis, and autophagy of prostate cancer cells by regulating miR-195/CCNE1 (13). An increasing number of studies have shown that lncRNAs/miRNAs/target genes play an important role in NSCLC (14,15). In our study, it was found that knockdown of lncRNA OXCT1-AS1 inhibited cell proliferation via miR-195/CCNE1 in NSCLC cell lines.
In future studies, we will continue to explore whether lncRNA OXCT1-AS1 can serve as a potential biomarker for patient risk stratification and loco regional metastasis in NSCLC. We are collecting more clinical information and gene expression data of NSCLC patients to provide a basis for future studies on the application of lncRNA OXCT1-AS1 as a clinical marker in patient risk stratification and loco regional metastasis of NSCLC. However, the clinical application of lnRNA OXCT1-AS1 still faces huge challenges. From the perspective of sensitivity, specificity, and reproducibility of lnRNA OXCT1-AS1, we still need more in-depth research.
In conclusion, there were significant differences in the expression of lncRNA OXCT1-AS1 and miR-195 in NSCLC tissues and cells. LncRNA OXCT1-AS1 was significantly upregulated and miR-195 was markedly downregulated in NSCLC tissues and cells. LncRNA OXCT1-AS1 can regulate the proliferative ability of NSCLC cells via the miR-195/CCNE1 signaling axis. Therefore, OXCT1-AS1 may be a prospective biomarker and therapeutic target for patients with NSCLC.
Acknowledgments
Funding: This study was funded by
Footnote
Reporting Checklist: The authors have completed the MDAR reporting checklist. Available at https://tcr.amegroups.com/article/view/10.21037/tcr-22-855/rc
Data Sharing Statement: Available at https://tcr.amegroups.com/article/view/10.21037/tcr-22-855/dss
Conflicts of Interest: All authors have completed the ICMJE uniform disclosure form (available at https://tcr.amegroups.com/article/view/10.21037/tcr-22-855/coif). All authors report that this study was funded by The Fundamental Research Fund Project of Higher Education of Heilongjiang, China (No. 2021-KYYWF-0361). The authors have no other conflicts of interest to declare.
Ethical Statement: The authors are accountable for all aspects of the work in ensuring that questions related to the accuracy or integrity of any part of the work are appropriately investigated and resolved. The study was conducted in accordance with the Declaration of Helsinki (as revised in 2013). The study was approved by institutional ethics board of The Third Affiliated Hospital of Qiqihar Medical University (No. 2021LL-9) and informed consent was taken from all the patients.
Open Access Statement: This is an Open Access article distributed in accordance with the Creative Commons Attribution-NonCommercial-NoDerivs 4.0 International License (CC BY-NC-ND 4.0), which permits the non-commercial replication and distribution of the article with the strict proviso that no changes or edits are made and the original work is properly cited (including links to both the formal publication through the relevant DOI and the license). See: https://creativecommons.org/licenses/by-nc-nd/4.0/.
References
- Rodriguez-Canales J, Parra-Cuentas E, Wistuba II. Diagnosis and Molecular Classification of Lung Cancer. Cancer Treat Res 2016;170:25-46. [Crossref] [PubMed]
- Nasim F, Sabath BF, Eapen GA. Lung Cancer. Med Clin North Am 2019;103:463-73. [Crossref] [PubMed]
- Bade BC, Dela Cruz CS. Lung Cancer 2020: Epidemiology, Etiology, and Prevention. Clin Chest Med 2020;41:1-24. [Crossref] [PubMed]
- Romaszko AM, Doboszyńska A. Multiple primary lung cancer: A literature review. Adv Clin Exp Med 2018;27:725-30. [Crossref] [PubMed]
- Jones GS, Baldwin DR. Recent advances in the management of lung cancer. Clin Med (Lond) 2018;18:s41-6. [Crossref] [PubMed]
- Jathar S, Kumar V, Srivastava J, et al. Technological Developments in lncRNA Biology. Adv Exp Med Biol 2017;1008:283-323. [Crossref] [PubMed]
- Bardhan A, Banerjee A, Basu K, et al. PRNCR1: a long non-coding RNA with a pivotal oncogenic role in cancer. Hum Genet 2022;141:15-29. [Crossref] [PubMed]
- Qian X, Zhao J, Yeung PY, et al. Revealing lncRNA Structures and Interactions by Sequencing-Based Approaches. Trends Biochem Sci 2019;44:33-52. [Crossref] [PubMed]
- Paraskevopoulou MD, Hatzigeorgiou AG. Analyzing MiRNA-LncRNA Interactions. Methods Mol Biol 2016;1402:271-86. [Crossref] [PubMed]
- Charles Richard JL, Eichhorn PJA. Platforms for Investigating LncRNA Functions. SLAS Technol 2018;23:493-506. [Crossref] [PubMed]
- Chen JB, Zhu YW, Guo X, et al. Microarray expression profiles analysis revealed lncRNA OXCT1-AS1 promoted bladder cancer cell aggressiveness via miR-455-5p/JAK1 signaling. J Cell Physiol 2019;234:13592-601. [Crossref] [PubMed]
- Zhong C, Yu Q, Peng Y, et al. Novel LncRNA OXCT1-AS1 indicates poor prognosis and contributes to tumorigenesis by regulating miR-195/CDC25A axis in glioblastoma. J Exp Clin Cancer Res 2021;40:123. [Crossref] [PubMed]
- Liang Y, Rong X, Luo Y, et al. A novel long non-coding RNA LINC00355 promotes proliferation of lung adenocarcinoma cells by down-regulating miR-195 and up-regulating the expression of CCNE1. Cell Signal 2020;66:109462. Erratum in: Cell Signal 2020;73:109715. [Crossref] [PubMed]
- Wang X, He C, Yang Z, et al. Dysregulation of long non-coding RNA SNHG12 alters the viability, apoptosis, and autophagy of prostate cancer cells by regulating miR-195/CCNE1 axis. Int J Clin Exp Pathol 2019;12:1272-83. [PubMed]
- Zhou Y, Shi H, Du Y, et al. lncRNA DLEU2 modulates cell proliferation and invasion of non-small cell lung cancer by regulating miR-30c-5p/SOX9 axis. Aging (Albany NY) 2019;11:7386-401. [Crossref] [PubMed]
- Li B, Zhu L, Li L, et al. lncRNA OXCT1-AS1 Promotes Metastasis in Non-Small-Cell Lung Cancer by Stabilizing LEF1, In Vitro and In Vivo. Biomed Res Int 2021;2021:4959381. [Crossref] [PubMed]
- Wang T, Tang X, Liu Y. LncRNA-ATB promotes apoptosis of non-small cell lung cancer cells through MiR-200a/β-Catenin. J BUON 2019;24:2280-6. [PubMed]
- Chen W, Zheng R, Zeng H, et al. The incidence and mortality of major cancers in China, 2012. Chin J Cancer 2016;35:73. [Crossref] [PubMed]
- Wei L, Sun JJ, Cui YC, et al. Twist may be associated with invasion and metastasis of hypoxic NSCLC cells. Tumour Biol 2016;37:9979-87. [Crossref] [PubMed]
- Jonna S, Subramaniam DS. Molecular diagnostics and targeted therapies in non-small cell lung cancer (NSCLC): an update. Discov Med 2019;27:167-70. [PubMed]
- Zhou W, Gong J, Chen Y, et al. Long noncoding RNA LINC00899 suppresses breast cancer progression by inhibiting miR-425. Aging (Albany NY) 2019;11:10144-53. [Crossref] [PubMed]
- Li Z, Qin X, Bian W, et al. Exosomal lncRNA ZFAS1 regulates esophageal squamous cell carcinoma cell proliferation, invasion, migration and apoptosis via microRNA-124/STAT3 axis. J Exp Clin Cancer Res 2019;38:477. [Crossref] [PubMed]
- Liao K, Lin Y, Gao W, et al. Blocking lncRNA MALAT1/miR-199a/ZHX1 Axis Inhibits Glioblastoma Proliferation and Progression. Mol Ther Nucleic Acids 2019;18:388-99. [Crossref] [PubMed]
- Wang LF, Wu LP, Wen JD. LncRNA AC079630.4 expression associated with the progression and prognosis in lung cancer. Aging (Albany NY) 2021;13:18658-68. [Crossref] [PubMed]
- Xue L, Li J, Lin Y, et al. m6 A transferase METTL3-induced lncRNA ABHD11-AS1 promotes the Warburg effect of non-small-cell lung cancer. J Cell Physiol 2021;236:2649-58. [Crossref] [PubMed]
- Luo J, Pan J, Jin Y, et al. MiR-195-5p Inhibits Proliferation and Induces Apoptosis of Non-Small Cell Lung Cancer Cells by Targeting CEP55. Onco Targets Ther 2019;12:11465-74. [Crossref] [PubMed]
- Long Z, Wang Y. miR-195-5p Suppresses Lung Cancer Cell Proliferation, Migration, and Invasion Via FOXK1. Technol Cancer Res Treat 2020;19:1533033820922587. [Crossref] [PubMed]
- Yu X, Zhang Y, Cavazos D, et al. miR-195 targets cyclin D3 and survivin to modulate the tumorigenesis of non-small cell lung cancer. Cell Death Dis 2018;9:193. [Crossref] [PubMed]
- Li L, Feng T, Zhang W, et al. MicroRNA Biomarker hsa-miR-195-5p for Detecting the Risk of Lung Cancer. Int J Genomics 2020;2020:7415909. [Crossref] [PubMed]
- Swaffer MP, Jones AW, Flynn HR, et al. CDK Substrate Phosphorylation and Ordering the Cell Cycle. Cell 2016;167:1750-61.e16. [Crossref] [PubMed]
- Wood DJ, Endicott JA. Structural insights into the functional diversity of the CDK-cyclin family. Open Biol 2018;8:180112. [Crossref] [PubMed]
- Macaluso E. Multisensory processing in sensory-specific cortical areas. Neuroscientist 2006;12:327-38. [Crossref] [PubMed]
- Arbour N, Ekandé S, Côté G, et al. Persistent infection of human oligodendrocytic and neuroglial cell lines by human coronavirus 229E. J Virol 1999;73:3326-37. [Crossref] [PubMed]
- Gorski JW, Ueland FR, Kolesar JM. CCNE1 Amplification as a Predictive Biomarker of Chemotherapy Resistance in Epithelial Ovarian Cancer. Diagnostics (Basel) 2020;10:279. [Crossref] [PubMed]
- Yang R, Xing L, Zheng X, et al. The circRNA circAGFG1 acts as a sponge of miR-195-5p to promote triple-negative breast cancer progression through regulating CCNE1 expression. Mol Cancer 2019;18:4. [Crossref] [PubMed]
- Wu Y, Zheng Q, Li Y, et al. Metformin targets a YAP1-TEAD4 complex via AMPKα to regulate CCNE1/2 in bladder cancer cells. J Exp Clin Cancer Res 2019;38:376. [Crossref] [PubMed]
- Chen L, Miao X, Si C, et al. Long Non-coding RNA SENP3-EIF4A1 Functions as a Sponge of miR-195-5p to Drive Triple-Negative Breast Cancer Progress by Overexpressing CCNE1. Front Cell Dev Biol 2021;9:647527. [Crossref] [PubMed]
(English Language Editor: A. Kassem)