Growth of T-cell lymphoma cells is inhibited by mPGES-1/PGE2 suppression via JAK/STAT, TGF-β/Smad3 and PI3K/AKT signal pathways
Introduction
T-cell lymphoma (TCL) comprises a highly heterogeneous group of invasive non-Hodgkin’s lymphomas with rapid disease progression, poor prognosis, and lack of effective treatment options (1). It is urgently needed to identify and develop novel therapeutic targets for TCL. Membrane-bound prostaglandin E2 synthase l (mPGES-1) is the specificity terminal rate-limiting enzyme of target compositing prostaglandin E2 (PGE2). Over-expression of mPGES-1 has been found in many types of solid tumors and hematologic malignancies (2-4). Our previous studies have shown that mPGES-1 was over-expressed in human leukemia primary cells and cell lines, and inhibiting mPGES-1 could suppress proliferation, induce apoptosis and arrest the cell cycle of leukemia cells in vitro and in vivo (5-7). However, the role and mechanism of mPGES-1/PGE2 in TCL has remained largely unknown.
Janus kinase/signal transduction and transcription (JAK/STAT) signal pathway, a downstream pathway of cytokine signaling, regulates the differentiation, proliferation and apoptosis of cells. It not only takes part in the regulation of normal physiological procedures, but also contributes to the development of tumors, especially in hematologic tumors (8). Multiple studies have also shown that the over-expression of STAT3 could activate oncogene expression and promote tumor progression (9-11). Transforming growth factor-β (TGF-β) signals transmit information through a variety of pathways and regulates downstream gene expressions, the most important of which is the Smad. TGF-β is highly expressed in multiple malignancies. Moreover, activation of the Smad signal by TGF-β enhances proliferation, metastasis, and invasive activities of tumor cells (12). Plenty of studies have confirmed that abnormal activation of the phosphatidylinositol 3-kinase/protein kinase B (PI3K/AKT) signaling pathway is present in a variety of tumors and PI3K inhibitor has been approved by the Food and Drug Administration (FDA) for the treatment of refractory or relapsed acute myelocytic leukemia (AML) most recently (13,14). However, the relationship between mPGES-1 and its potential downstream signals JAK/STAT, TGF-β/Smad and PI3K/AKT remains unknown in TCL. Whether mPGES-1could regulate these pathways and further participate in the occurrence of TCL has not been reported so far.
In the present study, we showed that TCL cell line Hut78 highly expressed mPGES-1. The proliferation of Hut78 cells was inhibited by CAY10526, a selective mPGES-1 inhibitor which also induced Hut78 cells apoptosis. This effect was partially attributed to the suppression of JAK/STAT, TGF-β/Smad and PI3K/AKT signal pathways. We present the following article in accordance with the MDAR reporting checklist (available at https://tcr.amegroups.com/article/view/10.21037/tcr-21-2834/rc).
Methods
Materials
The human TCL cell line Hut78 was obtained from Cell Bank of the Chinese Academy of Sciences (Shanghai, China). Normal mono-nuclear cells were separated from the peripheral blood of healthy volunteers. The anti-mPGES-1 antibody (#10004350) and mPGES-1 inhibitor CAY10526 (#10010088) were ordered from Cayman Chemical Company (Ann Arbor, MI, USA). Antibodies to JAK2 (#3230), STAT3 (#9139), p-STAT3 (#52075), Akt (#4691), p-Akt (#4060), TGF-β (#3709), Smad (#9523) and p-Smad (#9520) were obtained from Cell Signaling Technology (Danvers, MA, USA). Antibodies to JAK1 (ab133666), GAPDH (ab181602) and Caspase-3 (ab32351) were purchased from Abcam (Cambridge, MA, USA).
Cell culture
Hut78 Cells was cultured in in RPMI-1640 medium (Gibco, USA) supplemented by10% fetal bovine serum (FBS, Gibco, USA) at 37 ℃ in 5% CO2. Healthy peripheral blood mono-nuclear cells (PBMCs) donated by volunteers were separated by Ficoll-Hypaque gradient.
Cell viability assay
Hut78 cells were seeded at a density 5×104 cells/well in triplicate in 96-well plates and incubated for 72 hours with CAY10526 at various concentrations. Cell viability was measured using a cell counting kit-8 (CCK-8, DOJINDO, Japan) assay kit and the absorbance of wells at 450 nm was measured using a micro-plate reader. The experiments were performed repeatedly for three times.
Immunofluorescence analysis
Hut78 cells were plated onto glass cover-slips, fixed with 4% paraformaldehyde at room temperature for 30 minutes and blocked with 5% bovine serum albumin (BSA) in phosphate buffer solution (PBS) which contains 0.1% Triton X-100, then incubated with mPGES-1 antibody, followed by goat anti-rabbit IgG Cy3 (Abcam; ab6939) for 1 h. The cells were washed three times by PBS and stained with DAPI (Abcam; ab104139) for 30 min. Fluorescence images were acquired using a Zeiss LSM 800 Con-focal Imaging System. A Zeiss LSM 800 Con-focal Imaging System was used to acquire fluorescence images.
Apoptosis assay
A total of 1×106 of Hut78 cells were washed and re-suspended in binding buffer, followed by incubation with 5 µL of annexin-V-FITC (BD, USA) for 15 min in the dark at room temperature and subsequent incubation with 2.5 µL of propidium iodide (PI, Sigma, USA). Cells were analyzed by using FACScan flow cytometer (FACSCalibur, BD, USA). CellQuest software (v6.1x) was utilized for data acquisition and analysis.
PGE2 EIA analysis
Following the manufacturer’s protocol, PGE2 was measured by enzyme immunoassay (EIA) using a commercial kit (Cayman, Ann Arbor, USA). Negative, positive controls and standard curves were generated for PGE2 and analyzed with the samples. Results were calculated from the standard curve by cubic spline interpolation.
Western blot
Cells were lysed with appropriate volume of radioimmunoprecipitation buffer and determined by bicinchoninic acid assays. The lysates were resolved by sodium dodecyl sulfate-polyacrylamide gel electrophoresis (SDS-PAGE), transferred to polyvinylidene fluoride (PVDF) membrane, blocked Tris-buffered saline (TBS) containing 5% BSA diluted in TBS with Tween-20 for 1 h and incubated with primary antibodies overnight at 4 ℃. Membranes were probed with HRP-conjugated secondary antibody and developed using ECL reagents. Image J software (Bethesda, MD, USA) was used to calculate the density of bands.
Statistical analysis
Statistical analysis was done using SPSS 20.0 software. Results were provided as mean ± standard deviation (SD) from at least three biologically independent experiments. Statistical significance was conducted by using one-way analysis of variance (ANOVA). P<0.05 was considered to be statistically significant.
Ethical statement
The study was conducted in accordance with the Declaration of Helsinki (as revised in 2013) and approved by the Human Ethics Committee of Sun Yat-sen Memorial Hospital (SYSKY-2022-005-01) and informed consent were obtained from all healthy volunteers.
Results
mPGES-1 was highly expressed in Hut78 cells and suppression of mPGES-1/PGE2 by CAY10526 inhibited cell proliferation
To explore the potential role of mPGES-1 in TCL, the expression levels of mPGES-1 in normal human PBMC and Hut78 cell line were measured by using western blot and immunofluorescence. As shown in Figure 1A,1B, Hut78 cells expressed much higher level of mPGES-1 compared with normal PBMCs. Based on this preliminary finding, we chose CAY10526 (CAS:938069-71-7) to treat Hut78 cells. As several studies have shown that CAY10526 is an isoform-selective inhibitor of PGE2 production through the selective modulation of mPGES-1 expression (15-17), and was even selected as reference compound when newer compound was synthesized and tested (18). The dose of CAY10526 used in the studies ranged from 1.8 to 300 µM for different cells and experimental conditions, including our previous report showing CAY10526 [half inhibitory concentration (IC50): 16.7 µM at 24 h] reduced PGE2 synthesis in primary T-cell acute lymphoblastic leukemia (T-ALL) cells (7). Therefore, 10–80 µM of CAY10526 were used in the subsequent experiments. As demonstrated in Figure 1C, mPGES-1 was remarkably down-regulated compared with untreated cells. Meanwhile, the EIA data showed that the concentration of PGE2 in culture supernatant of Hut78 cells was decreased after treatment with CAY10526 for 24 h (Figure 1D). Furthermore, cell viability examined by CCK-8 showed that the growth of Hut78 cells was inhibited by CAY10526 in a dose-dependent manner. The IC50 of CAY10526 for Hut78 cell at 24 h was 27.64 µM (Figure 1E).
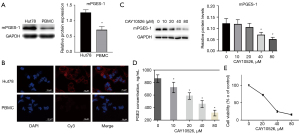
CAY10526 inhibited Hut78 cell proliferation via inducing apoptosis
Apoptosis is a common mode of cell death in hematological malignant cells treated with anticancer drugs. To investigate the possible mechanism of CAY10526 on the inhibition of cell proliferation, we examined the cell apoptosis rate of Hut78 cells via flow cytometer. After being treated with CAY10526 for 24 h, the apoptosis rate of Hut78 cells significantly increased (Figure 2A). Moreover, elevation of CAY10526 concentration led to a significantly increased expression of cleaved caspase-3 (Figure 2B). Taken together, these results suggested that CAY10526 induced cell death mainly through the apoptotic pathway.
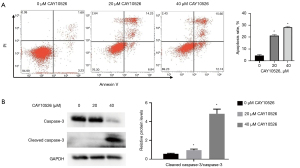
CAY10526 inhibited the JAK/STAT, TGF-β/Smad3 and PI3K/AKT signaling pathway
JAK/STAT, TGF-β/Smad3 and PI3K/AKT play a significant role in cell proliferation. To get insights into the effects of mPGES-1 on these signaling pathways, we used Western blotting to analyze the expression of JAK1, JAK2, STAT3, pSTAT3, TGF-β/Smad3 and PI3K/AKT in Hut78 cells after being treated by CAY10526 for 24 h. As shown in Figure 3A, there was a decrease in the expression of JAK1, JAK2 and pSTAT3 in Hut78 cells after treatment with CAY10526, while expression of total STAT3 did not decrease. For PI3K/AKT signaling pathway, the expression of PI3Kp110, PI3Kp85 and p-AKT protein declined as the increase of CAY10526 concentration (Figure 3B). We also examined the expression of TGF-β/Smad3 pathway, including TGF-β, Smad3 and p-Smad3 in Hut78 cells. The results revealed that no significant variation of Smad3 expression in Hut78 cells was detected. However, the level of phosphorylation of Smad3 was largely decreased after treatment with CAY10526. In addition, the level of TGF-β was slightly suppressed by CAY10526 (Figure 3C). Since CyclinD1 plays a role in cell apoptosis regulation, we tested whether CAY10526-induced apoptosis was associated with CyclinD1 expression. As shown in Figure 3D, there was a decrease in CyclinD1 in Hut78 cells after being exposed to CAY10526. Collectively, these results suggested CAY10526 might induce apoptosis of Hut78 cells via inhibition of CyclinD1 and JAK/STAT, TGF-β/Smad3 and PI3K/AKT signaling pathway.
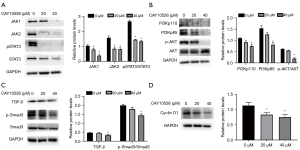
Discussion
TCL is a type of lymphoma with aggressive clinical behavior and adverse prognosis (19). The efficacy of current therapeutic strategies for TCL is unsatisfying, and new therapeutic targets are urgently needed. For the first time, our report elucidated the importance of mPGES-1 in proliferation of TCL and its possible mechanism, providing a potential therapeutic rationale for TCL.
In the present study, we found that the mPGES-1 expression was much higher in Hut78 cells compared with normal PBMC, in line with findings from other tumors such as prostate cancer, colon cancer and breast cancer (20,21), suggesting that mPGES-1 might play a potential therapeutic role in TCL. CAY10526, a selective inhibitor of mPGES-1, was chosen to treat Hut78 cells. As expected, the expression of mPGES-1 decreased together with the decrease of PGE2 in cell culture supernatant after treatment with CAY10526, indicating that inhibition of mPGES-1 could reduce PGE2 synthesis. Accordingly, the cell growth was inhibited by CAY10526 in a dose-dependent manner, indicating a key role of mPGES-1/PEG2 in the proliferation of TCL cells. Moreover, the apoptosis of Hut78 cells was increased, confirmed by both Annexin V/PI staining and western blot analysis of increase of cleavage of caspase-3, in line with research on melanoma cell (22). These results demonstrated that the inhibition of TCL cells growth by mPGES-1/PEG2 inhibition was at least partly due to the induction of apoptosis.
It has been found that down-regulation of mPGES-1/PGE2 expression in macrophages can simultaneously inhibit the JAK/STAT signaling pathway (23). Study have shown that the JAK/STAT pathway is the backbone of intracellular protein interactions and involved in various biological behaviors, including cell proliferation, apoptosis and differentiation via regulating downstream regulators such as cyclinD1, P21, P27, Bcl-XL and Bcl-2 (24). Abnormal activation of specific JAK/STAT signal results in occurrence of a variety of tumors, including hematological malignancies. JAK inhibitor, ruxolitinib, has been approved to use in patients with myeloproliferative neoplasms (25). In this study, we found that Hut78 cells displayed high level of phosphorylated STAT3, a marker of JAK/STAT pathway activation. The expression of JAK1, JAK2 and pSTAT3 decreased after treatment with CAY10526, indicating that suppression of mPGES-1/PGE2 could down-regulate the JAK/STAT pathway. Moreover, cyclinD1 expression was simultaneously inhibited. Since cyclinD1 is a downstream regulator of JAK/STAT signal, we infer that the induction of apoptosis by CAY10526 might be correlated with the down-regulation of CyclinD1. These results implied that the inhibition of mPGES-1/PGE2 by CAY10526 suppressed the activation of JAK/STAT and CyclinD1 expression, leading to the apoptosis of TCL cells.
TGF-β, a multifunctional cytokine, inhibits the cell growth, regulates diverse biological processes, and plays a dual role acting as either tumor suppressor or promoter. TGF-β transduces its signal through combining with TGF-βII cell surface receptors, so as to activate phosphorylation of Smad-2 and Smad-3 (26). Moreover, TGF-β stimulates other non-canonical effectors (e.g., PI3K/AKT, ERK1/2, p38MAPK, NF-κB and RhoA) which contributes to the complexity of its stimulation response. The alternate activation of pathways lead to neoplasm formation (27). However, the interaction between TGF-β and mPGES-1/PGE2 remains unclear. Study has found that PGE2 and prostaglandin I2 (PGI2) could stimulate cell proliferation and TGF-β secretion by activating protein kinase C (28). De Araújo showed that TGF-β dual regulation over COX-2/PGE2 tumor promotion depended on the activation status of H-Ras and Wnt/β-catenin pathways in intestinal tumor cells (29). In breast cancer, TGF-β-activated kinase-like protein (TAK1L), which was identified as a negative regulator for p38MAPK activation, was down-regulated after ablation of mPGES-1 in cancer-associated fibroblasts (CAFs) (30). In our study, the expression of TGF-β and p-Smad3 of Hut78 cells decreased after exposure to CAY10526, indicating that TGF-β/Smad pathway was inhibited via the down-regulation of mPGES-1/PGE2, leading to inhibition of cell proliferation and induction of apoptosis. However, the interplay between TGF-β/Smad pathway and mPGES-1/PGE2 in this context remains to be further elucidated.
PI3K/AKT signal transduction pathway is strongly associated with the occurrence and progression of various malignancies. PI3K phosphorylates AKT to be P-Akt, which is antagonized by PTEN. P-Akt is over-expressed in many human cancers (31,32). Our study showed that the expressions of PI3Kp110, PI3Kp85, P-Akt and AKT in Hut78 cells decreased after being treated with CAY10526, indicating that mPGES-1/PGE2 inhibition may also suppress the proliferation of TCL cells and promote cell apoptosis by suppressing the PI3K/AKT pathway. In breast cancer, Nandi P showed that PGE2 produced by tumor and macrophages could activate the PI3K/AKT signaling pathway (33). Our findings suggested that CAY10526 could decrease mPGES-1 expression and reduce PGE2 production, which would subsequently inhibit PI3K/AKT pathway, resulting in inhibition of TCL cells proliferation.
JAK/STAT, TGF-β/Smad and PI3K/AKT signal pathways could connect with each other and influence by each other. It has been found that TGF-β directly stimulated PI3K/AKT signaling pathway to regulate the nuclear translocation of Smad3 (34). TGF-β directly or indirectly activated the PI3K/AKT pathway was reported by another group (35). In addition, studies also have shown that TGF-β could positively or negatively regulate the JAK/STAT signaling pathway in different cell types (36,37). Therefore, we speculate that the effects of mPGES-1 inhibition on the JAK/STAT, TGF-β/Smad, and PI3K/AKT signal were extremely complex and there might be some key interaction effector among the three pathways (Figure 4). The precise mechanism of their mutual regulation definitely needs to be further explored in the future.
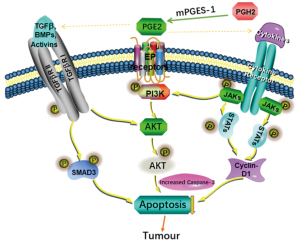
Other signaling pathways and/or mechanisms might also be involved in the modulation of tumor growth mediated by mPGES1/PGE2, as seen in a variety of malignancies. For lung cancer, it has been shown that CAY10526 restores T-cell immunity via inhibition of mPGES1, contributing to suppression of lung metastasis in Gprc5a-ko mouse model (17). In breast cancer, disruption of PGE2 signaling in CAFs represses carcinoma growth but promotes metastasis (30). Another study support the prognostic value of mPGES1, and suggest targeting this pathway may serve as novel therapeutic avenue for melanoma (22). The study even shed light on the mPGES1’s function and cross talk with inflammatory regulators, which may deepen our understanding of how inflammation promotes tumor progression. Skin carcinogenesis was also repressed by gene deletion of mPGES1 via suppression of IL-17 and other inflammatory cytokines (38). Other key molecules included Y-box binding protein 1 (YB-1) (22), NF-κB, etc. (39). In our group, we reported that c-Jun N-terminal kinase served a role in the mPGES-1/PGE2/EP4/MAPK positive feedback loops in T-ALL cells. Meanwhile, P38 and extracellular signal-regulated kinase 1/2 exerted negative feedback on mPGES-1 (5,30). More recently, we published that mPGES-1/PGE2 promoted proliferation of T-ALL cells by modulating MTDH via the EP3/cAMP/PKA/CREB pathway (7). In addition, we have found high expression of COX-2/mPGES-1 predicted a poor prognosis of diffuse large B cell lymphoma and affected cell proliferation by interacting with NF-κB p65 (manuscript in submission).
Our study has some limitations. Firstly, only one inhibitor of mPGES1, CAY10526, was used. However, its efficacy and selectivity on mPGES1 has been confirmed (15-17) including in our previous report (7), and was even employed as reference compound (18). Experiments are currently undergoing including knock down of mPGES-1 by RNA silencing and exogenous PGE2 supplement for rescue. Secondly, the precise mechanisms of mutual regulation of mPGES-1/PGE2, JAK/STAT, TGF-β/Smad3 and PI3K/AKT have not been investigated and undoubtedly require further exploration. Thirdly, the prognostic value of mPGES1 in TCL patients has not been determined. We are currently collecting these clinical samples and information to get new insights into the therapeutic implication of mPGES1 for TCL.
To the best of our knowledge, ours is the first report showing that mPGES-1 might be a novel therapeutic target for TCL. The suppression of mPGES-1/PGE2 lead to apoptosis induction and proliferation inhibition of TCL cells, probably via the JAK/STAT, TGF-β/Smad3 and PI3K/AKT signaling pathways. The precise interplay between these complex signaling network regulators remains to be further studied.
Acknowledgments
Funding: This work was supported by the National Natural Science Foundation of China (Nos. 82000153, 81900022, 81803783, 82000198), the Guangdong Medical Science and Technology Research Fund (No. A2019207), the Natural Science Foundation of Guangdong Province (No. 2018A030310091), the Science and Technology Foundation of Guangdong Province (Grant No. 2016A020215062, KTP2020324), the Key Laboratory of Malignant Tumor Molecular Mechanism and the Translational Medicine of Guangzhou Bureau of Science and Information Technology [Grant No. 163 (2013)], Key Laboratory of Malignant Tumor Gene Regulation and the Target Therapy of Guangdong Higher Education Institutes (Grant No. KLB09001).
Footnote
Reporting Checklist: The authors have completed the MDAR reporting checklist. Available at https://tcr.amegroups.com/article/view/10.21037/tcr-21-2834/rc
Data Sharing Statement: Available at https://tcr.amegroups.com/article/view/10.21037/tcr-21-2834/dss
Peer Review File: Available at https://tcr.amegroups.com/article/view/10.21037/tcr-21-2834/prf
Conflicts of Interest: All authors have completed the ICMJE uniform disclosure form (available at https://tcr.amegroups.com/article/view/10.21037/tcr-21-2834/coif). The authors have no conflicts of interest to declare.
Ethical Statement: The authors are accountable for all aspects of the work in ensuring that questions related to the accuracy or integrity of any part of the work are appropriately investigated and resolved. The study was conducted in accordance with the Declaration of Helsinki (as revised in 2013) and approved by the Human Ethics Committee of Sun Yat-sen Memorial Hospital (SYSKY-2022-005-01).
Open Access Statement: This is an Open Access article distributed in accordance with the Creative Commons Attribution-NonCommercial-NoDerivs 4.0 International License (CC BY-NC-ND 4.0), which permits the non-commercial replication and distribution of the article with the strict proviso that no changes or edits are made and the original work is properly cited (including links to both the formal publication through the relevant DOI and the license). See: https://creativecommons.org/licenses/by-nc-nd/4.0/.
References
- Ghione P, Moskowitz AJ, De Paola NEK, et al. Novel Immunotherapies for T Cell Lymphoma and Leukemia. Curr Hematol Malig Rep 2018;13:494-506. [Crossref] [PubMed]
- Psarra A, Nikolaou A, Kokotou MG, et al. Microsomal prostaglandin E2 synthase-1 inhibitors: a patent review. Expert Opin Ther Pat 2017;27:1047-59. [Crossref] [PubMed]
- Hara S. Prostaglandin terminal synthases as novel therapeutic targets. Proc Jpn Acad Ser B Phys Biol Sci 2017;93:703-23. [Crossref] [PubMed]
- Bergqvist F, Ossipova E, Idborg H, et al. Inhibition of mPGES-1 or COX-2 Results in Different Proteomic and Lipidomic Profiles in A549 Lung Cancer Cells. Front Pharmacol 2019;10:636. [Crossref] [PubMed]
- Li YQ, Chen JT, Yin SM, et al. Regulation of mPGES-1 composition and cell growth via the MAPK signaling pathway in jurkat cells. Exp Ther Med 2018;16:3211-9. [Crossref] [PubMed]
- Li Y, Yin S, Nie D, et al. MK886 inhibits the proliferation of HL-60 leukemia cells by suppressing the expression of mPGES-1 and reducing prostaglandin E2 synthesis. Int J Hematol 2011;94:472-8. [Crossref] [PubMed]
- Li Y, Chen J, Yang W, et al. mPGES-1/PGE2 promotes the growth of T-ALL cells in vitro and in vivo by regulating the expression of MTDH via the EP3/cAMP/PKA/CREB pathway. Cell Death Dis 2020;11:221. [Crossref] [PubMed]
- Xin P, Xu X, Deng C, et al. The role of JAK/STAT signaling pathway and its inhibitors in diseases. Int Immunopharmacol 2020;80:106210. [Crossref] [PubMed]
- Zhu F, Wang KB, Rui L. STAT3 Activation and Oncogenesis in Lymphoma. Cancers (Basel) 2019;12:19. [Crossref] [PubMed]
- Yang X, Tang Z, Zhang P, et al. Research Advances of JAK/STAT Signaling Pathway in Lung Cancer. Zhongguo Fei Ai Za Zhi 2019;22:45-51. [PubMed]
- Wu Q, Wu W, Fu B, et al. JNK signaling in cancer cell survival. Med Res Rev 2019;39:2082-104. [Crossref] [PubMed]
- Papageorgis P, Stylianopoulos T. Role of TGFβ in regulation of the tumor microenvironment and drug delivery Int J Oncol 2015;46:933-43. (review). [Crossref] [PubMed]
- Hoxhaj G, Manning BD. The PI3K-AKT network at the interface of oncogenic signalling and cancer metabolism. Nat Rev Cancer 2020;20:74-88. [Crossref] [PubMed]
- Li YJ, Li XF, Yang EH, et al. Reaserch Advances on the Role of PI3K/AKT Signaling Pathway and MiRNA in Acute T-Cell Lymphocytic Leukemia--Review. Zhongguo Shi Yan Xue Ye Xue Za Zhi 2019;27:1344-7. [PubMed]
- Guerrero MD, Aquino M, Bruno I, et al. Synthesis and pharmacological evaluation of a selected library of new potential anti-inflammatory agents bearing the gamma-hydroxybutenolide scaffold: a new class of inhibitors of prostanoid production through the selective modulation of microsomal prostaglandin E synthase-1 expression. J Med Chem 2007;50:2176-84. [Crossref] [PubMed]
- Yu R, Xiao L, Zhao G, et al. Competitive enzymatic interactions determine the relative amounts of prostaglandins E2 and D2. J Pharmacol Exp Ther 2011;339:716-25. [Crossref] [PubMed]
- Wang T, Jing B, Xu D, et al. PTGES/PGE2 signaling links immunosuppression and lung metastasis in Gprc5a-knockout mouse model. Oncogene 2020;39:3179-94. [Crossref] [PubMed]
- Di Micco S, Terracciano S, Ruggiero D, et al. Identification of 2-(thiophen-2-yl)acetic Acid-Based Lead Compound for mPGES-1 Inhibition. Front Chem 2021;9:676631. [Crossref] [PubMed]
- Elenitoba-Johnson KSJ. Functional profiling to improve therapy in TCL. Blood 2019;133:504-6. [Crossref] [PubMed]
- Finetti F, Terzuoli E, Giachetti A, et al. mPGES-1 in prostate cancer controls stemness and amplifies epidermal growth factor receptor-driven oncogenicity. Endocr Relat Cancer 2015;22:665-78. [Crossref] [PubMed]
- Banning A, Florian S, Deubel S, et al. GPx2 counteracts PGE2 production by dampening COX-2 and mPGES-1 expression in human colon cancer cells. Antioxid Redox Signal 2008;10:1491-500. [Crossref] [PubMed]
- Kim SH, Hashimoto Y, Cho SN, et al. Microsomal PGE2 synthase-1 regulates melanoma cell survival and associates with melanoma disease progression. Pigment Cell Melanoma Res 2016;29:297-308. [Crossref] [PubMed]
- Jo AR, Han HS, Seo S, et al. Inhibitory effect of moschamine isolated from Carthamus tinctorius on LPS-induced inflammatory mediators via AP-1 and STAT1/3 inactivation in RAW 264.7 macrophages. Bioorg Med Chem Lett 2017;27:5245-51. [Crossref] [PubMed]
- Tettweiler G, Blaquiere JA, Wray NB, et al. Hipk is required for JAK/STAT activity during development and tumorigenesis. PLoS One 2019;14:e0226856. [Crossref] [PubMed]
- Bose P, Verstovsek S. JAK2 inhibitors for myeloproliferative neoplasms: what is next? Blood 2017;130:115-25. [Crossref] [PubMed]
- Zhang Y, Alexander PB, Wang XF. TGF-β Family Signaling in the Control of Cell Proliferation and Survival. Cold Spring Harb Perspect Biol 2017;9:a022145. [Crossref] [PubMed]
- Che D, Zhang S, Jing Z, et al. Macrophages induce EMT to promote invasion of lung cancer cells through the IL-6-mediated COX-2/PGE2/β-catenin signalling pathway. Mol Immunol 2017;90:197-210. [Crossref] [PubMed]
- Sui G, Cheng G, Yuan J, et al. Interleukin (IL)-13, Prostaglandin E2 (PGE2), and Prostacyclin 2 (PGI2) Activate Hepatic Stellate Cells via Protein kinase C (PKC) Pathway in Hepatic Fibrosis. Med Sci Monit 2018;24:2134-41. [Crossref] [PubMed]
- de Araújo WM, Tanaka MN, Lima PHS, et al. TGF-β acts as a dual regulator of COX-2/PGE2 tumor promotion depending of its cross-interaction with H-Ras and Wnt/β-catenin pathways in colorectal cancer cells. Cell Biol Int 2021;45:662-73. [Crossref] [PubMed]
- Elwakeel E, Brüggemann M, Wagih J, et al. Disruption of Prostaglandin E2 Signaling in Cancer-Associated Fibroblasts Limits Mammary Carcinoma Growth but Promotes Metastasis. Cancer Res 2022;82:1380-95. [Crossref] [PubMed]
- Robbins HL, Hague A. The PI3K/Akt Pathway in Tumors of Endocrine Tissues. Front Endocrinol (Lausanne) 2016;6:188. [PubMed]
- Xu S, Li Y, Lu Y, et al. LZTS2 inhibits PI3K/AKT activation and radioresistance in nasopharyngeal carcinoma by interacting with p85. Cancer Lett 2018;420:38-48. [Crossref] [PubMed]
- Nandi P, Girish GV, Majumder M, et al. PGE2 promotes breast cancer-associated lymphangiogenesis by activation of EP4 receptor on lymphatic endothelial cells. BMC Cancer 2017;17:11. [Crossref] [PubMed]
- Zhang YE. Non-Smad pathways in TGF-beta signaling. Cell Res 2009;19:128-39. [Crossref] [PubMed]
- Song N, Zhong J, Hu Q, et al. FGF18 Enhances Migration and the Epithelial-Mesenchymal Transition in Breast Cancer by Regulating Akt/GSK3β/B-Catenin Signaling. Cell Physiol Biochem 2018;49:1019-32. [Crossref] [PubMed]
- Wang G, Yu Y, Sun C, et al. STAT3 selectively interacts with Smad3 to antagonize TGF-β signalling. Oncogene 2016;35:4388-98. [Crossref] [PubMed]
- Luo K. Signaling Cross Talk between TGF-β/Smad and Other Signaling Pathways. Cold Spring Harb Perspect Biol 2017;9:a022137. [Crossref] [PubMed]
- Sasaki Y, Kuwata H, Aida E, et al. Gene Deletion of Microsomal Prostaglandin E Synthase-1 Suppresses Chemically Induced Skin Carcinogenesis. Anticancer Res 2021;41:1307-14. [Crossref] [PubMed]
- Zhang YY, Yao YD, Luo JF, et al. Microsomal prostaglandin E2 synthase-1 and its inhibitors: Molecular mechanisms and therapeutic significance. Pharmacol Res 2022;175:105977. [Crossref] [PubMed]