Effect of IL-8 on hepatocellular carcinoma-associated metastasis by targeting MMP9 in mice
Introduction
Hepatocellular carcinoma (HCC) is the main histological subtype of liver cancer, accounting for 90% of primary liver cancer, and is the second leading cause of cancer-related deaths worldwide (1). The treatment methods for early HCC include surgical resection, percutaneous liver tumor ablation, and liver transplantation, but for advanced HCC, the curative effect of these methods is limited, so the 5-year survival rate of advanced HCC is extremely low (2). The poor prognosis of HCC is related to the high recurrence rate and metastasis rate of HCC (1). Therefore, it is of great significance to explore the mechanism of metastasis regulation and seek new treatment methods for improving the prognosis of patients.
Studies have found that the high expression of interleukin-8 (IL-8) in the serum of patients with HCC is related to the poor prognosis of the patients, which has caused more attention to the potential of IL-8 as a biomarker for patients with HCC (3,4). IL-8 is a chemotactic cytokine, which can activate several downstream signaling pathways after binding to its receptor C-X-C chemokine receptor 1 (CXCR1) or CXCR2, thus having a certain impact on the angiogenesis and metastasis of tumor (5). In HCC, studies have shown that IL-8 is related to tumor cell epithelial-mesenchymal transition and cancer cell invasion of blood vessels (6,7).
Of note, one of the mechanisms by which IL-8 regulates tumor metastasis is to regulate the expression of matrix metalloproteinase (MMP) (8). MMPs can destroy the extracellular matrix, reduce the connection between cells and promote tumor cell invasion and migration. MMP9, a gelatinase, is one of the most studied MMPs in the mechanism of HCC metastasis (9). It is overexpressed in HCC and is closely related to the local and remote metastasis of tumor cells, the reduction of apoptosis, and the increase of angiogenesis (9). A study by Zarogoulidis et al. has found that IL-8 can enhance the expression of MMP9 to promote tumor metastasis activity (8). Although both IL-8 and MMP9 are closely related to the poor prognosis of HCC, it is unclear whether IL-8 regulates the metastasis of HCC by regulating the expression of MMP9. Therefore, we sought to clarify the causal role of IL-8 and MMP9 in HCC metastasis. We present the following article in accordance with the ARRIVE reporting checklist (available at https://tcr.amegroups.com/article/view/10.21037/tcr-22-37/rc).
Methods
Animals
A total of 79 BALB/C nude mice (18–25 g) were purchased from Shanghai Slack Laboratory Animal Co., Ltd. [SCXK (Shanghai) 2007-0005, Shanghai, China]. All mice were housed in a specific pathogen-free (SPF) animal facility, 60–65% humidity, 12-hour light-dark cycle, at 25 ℃, with free access to water and food, and begin the experiment about 1 week after acclimatization. All the animal experiments were performed in strict accordance with the recommendations in the Guide for the Care and Use of Laboratory Animals by the National Institutes of Health and approved by the Ethics Committee of Experimental Animals of Zhejiang Chinese Medical University (approval No. ZSLL-2019-186).
Establishment of an orthotopic implantation mouse model of HCC
Human HCC cells HCCLM6 obtained from the Chinese Academy of Sciences Cell Bank were cultured in Dulbecco’s modified Eagle medium (DMEM) (10569010, Thermo Fisher, USA) containing 10% fetal bovine serum (FBS) (10091, Gibco, USA) and 1% penicillin-streptomycin (15140-122, Gibco, USA) in 37 ℃, 5% CO2 incubator (Forma Steri-Cycle, Thermo Fisher, USA). HCCLM6 cells stably expressing the green fluorescent protein (GFP) were constructed as previously described (10).
BALB/C nude mice were anesthetized with an intraperitoneal (i.p.) injection of 45 mg/kg pentobarbital sodium (P-010, Sigma Aldrich, USA) followed by subcutaneous inoculation of tumor cells. Briefly, HCCLM6 cells (1×107) were subcutaneously inoculated into the ventral forearm of BALB/c nude mice (n=2). Four weeks later, mice were put to death by cervical dislocation, the tumor tissue was separated and cut into tumor tissue blocks of approximately 2 mm × 2 mm × 1 mm with ophthalmological scissors for use.
After anesthesia, the mice were taken to the supine position, and the skin was cut layer by layer with tissue scissors to expose the liver. A 3-mm incision was made in the middle of the left lobe of the liver as the transplantation site. The prepared tumor tissue was carefully inserted into the mouse liver. Finally, the abdominal cavity was sewn layer by layer and the wound was disinfected.
To evaluate the role of IL-8 in the model, mice were randomly assigned to 2 groups, 7 mice in each group. One day after HCC transplantation in mice, mice in the control group were administered i.p. with normal saline every day, 0.1 mL/time, once a day; Mice in the IL-8 group were administered i.p. with exogenous IL-8 (1 µg/mouse) every day. All mice were given drugs continuously for 4 weeks. After 4 weeks, the mice were killed by cervical dislocation, and the liver and lung were isolated.
To evaluate the effects of protein kinase C (PKC) inhibitors (HY-12048, MedChemExpress, USA) and ERK1/2 inhibitors (HY-12028, MedChemExpress, USA) on tumor metastasis in an orthotopic implantation mouse model of HCC, the mice were divided into 3 groups with 7 mice in each group. One day after HCC transplantation in mice, mice in the IL-8 group were administered i.p. with exogenous IL-8 (1 µg/mouse) every day. Mice in IL-8 + PKC inhibitors group were administered i.p. with exogenous IL-8 (1 µg/mouse) and PKC inhibitors (5 mg/kg/mouse) every day. Mice in IL-8 + ERK1/2 inhibitors group were administered i.p. with exogenous IL-8 (1 µg/mouse) and ERK1/2 inhibitors (10 mg/kg/mouse) every day. All mice were given drugs continuously for 4 weeks. After 4 weeks, the mice were killed by cervical dislocation, and the liver and lung were isolated.
In vivo colonization assay
Liver metastasis model: after anesthesia, the left upper abdomen of mice was cut open, and the spleen was pulled out from the abdominal cavity with tweezers. Then HCCLM6 cells (5×106/well) were slowly injected into the spleen by syringe. After injection, the spleen was sent back to its original position, and a 75% alcohol cotton ball was used to compress the needle eye, and finally, the wound was sutured. Lung metastasis model: HCCLM6 cells (5×106) were resuspended with serum-free DMEM and then injected into mice through the tail vein.
The mice of the above two models were divided into two groups: the control group and the IL-8 group, with 7 mice in each group, a total of 28 mice. Mice in the control group were administered i.p. with normal saline every day, 0.1 mL/time, once a day. Mice in the IL-8 group were administered i.p. with exogenous IL-8 (1 µg/mouse) every day. All mice were given drugs continuously for 6 weeks. After 6 weeks, the mice were killed by cervical dislocation, and the liver and lung were isolated.
Liver metastasis model mice were divided into the IL-8 group and the IL-8 + MMP9 inhibitor group, with 7 mice in each group. Mice in the IL-8 group were administered i.p. with IL-8 (1 µg/mouse) every day, 0.1 mL/time, once a day. Mice in the IL-8 + MMP9 inhibitor group were administered i.p. with exogenous IL-8 (1 µg/mouse) and intravenously injected with MMP9 inhibitor (20 mg/kg, 6 days/week; 444293, Merck, German) every day. All mice were given drugs continuously for 6 weeks. After 6 weeks, the mice were killed by cervical dislocation, and the liver and lung were isolated.
Hematoxylin and eosin (H&E) staining
The collected liver and lung tissues were fixed in 4% paraformaldehyde (P0099, Beyotime, China), and then embedded in paraffin to make 5-µm sections. After the sections were deparaffinized and hydrated, they were stained with hematoxylin and eosin using an H&E staining kit (C0105, Beyotime, China) according to the manufacturer’s protocol. After the sections were dehydrated and transparent, they were sealed by neutral balsam (G8590, Solarbio, China). The metastasis foci were measured under a microscope (BX53M, Olympus, Japan).
Count of circulating tumor cells (CTCs)
When the mice were sacrificed, blood was taken from the heart and collected into the anticoagulant tube, and then the number of CTCs in the whole blood was measured by flow cytometry (DxFLEX, Beckman, USA).
Quantitative reverse transcription-polymerase chain reaction (qRT-PCR)
Total RNA was extracted using the RNA simple total RNA extraction kit (DP419, TIANGEN, China) following the manufacturer’s instructions. Purified RNA was reverse-transcribed by TIANScript Ⅱ RT Kit (KR107, TIANGEN, China) and detected by Fast Start Universal SYBR Green Master (4913850001, Roche, Germany) on the Real-Time PCR Detection system (CFX96, Bio-Rad, USA). The relative expression levels were calculated by the 2−ΔΔCT method, and glyceraldehyde-3-phosphate dehydrogenase (GAPDH) was used to normalize the gene expressions. The sequences of the primers are listed as follows: MMP9, forward: 5'-CAAGCTGGACTCGGTCTTTGA-3'; reverse: 5'-GCCTGTGTACACCCACACCT-3' and GAPDH, forward: 5'-CTCTCTGCTCCTCCTGTTCGACAG-3'; reverse: 5'-GTGTAATCATATTGGAACATGTAG-3'.
Immunohistochemistry (IHC)
IHC was performed with the help of the IHC Kit (E-IR-R221, Elabscience, China). Briefly, the sections needed to be placed in Dewaxing/Antigen Retrieval Buffer for heating. Then the sections were soaked in SP Reagent B Peroxidase Blocking Buffer to eliminate endogenous peroxidase activity. After the sections were blocked by 5% BSA (E661003, Sangon, China), they were incubated with the primary antibody anti-MMP9 (ab283575, Abcam, UK) at 4 ℃ overnight, followed by secondary antibody incubation (ab97051, Abcam, UK). Subsequently, the sections were reacted with DAB color solution (DA1010, Solarbio, China), hematoxylin buffer. After re-bluing treatment, the expression of MMP9 was observed under a microscope.
Cell grouping and treatment
Cell experiments were divided into three parts, there were three groups in the first part: blank group (HCCLM6 cells were normally cultured), IL-8 treated groups (HCCLM6 cells were treated with 15 or 30 ng/mL IL-8 for 24 hours). And in the second part, HCCLM6 cells were treated with 15 ng/mL IL-8 for 0, 15, 18 and 36 hours, respectively. In the third part, there were four groups as follows: blank group (HCCLM6 cells were normally cultured), IL-8 group (HCCLM6 cells were treated with 15 ng/mL IL-8 for 24 hours), IL-8 + PKC inhibitors group (HCCLM6 cells were treated with 15 ng/mL IL-8 and 20 µM PKC inhibitors for 24 hours) and IL-8 + ERK1/2 inhibitors group (HCCLM6 cells were treated with 15 ng/mL IL-8 and 10 µM ERK1/2 inhibitors for 24 hours).
Western blot
Total protein was extracted by RIPA lysis buffer (R0010, Solarbio, China), and qualified by BCA protein assay kit (BI-WB005, Sbjbio, China), separated by sodium dodecyl sulfate-polyacrylamide gel electrophoresis (SDS-PAGE) and then electrically transferred onto PVDF membranes (AR0136-02, Boster, China). After being blocked by 5% BSA, the membrane was cultured with primary antibodies overnight at 4 ℃. The primary antibodies were as follows: anti-MMP9, anti-p-PKC (ab109539, Abcam, UK), anti-PKC (ab179521, Abcam, UK), anti-ERK1/2 (ab184699, Abcam, UK), anti-p-ERK1/2 (ab278538, Abcam, UK), anti-β-actin (ab8226, Abcam, UK) and anti-α-tubulin (ab7291, Abcam, UK). After washing with TBST buffer, membranes were incubated with goat anti-rabbit (ab6721, Abcam, UK) or goat anti-mouse (ab205719, Abcam, UK). The relative intensity of the protein bands was visualized by the ECL luminescence kit (PE0010, Solarbio, China) on the iBright FL1500 Imaging System (A44115, Invitrogen, USA).
Statistical analysis
Statistical analysis experimented with GraphPad Prism 8.0. The measurement data were expressed by mean ± standard deviation. One-way ANOVA was used for multiple group comparisons. Differences with P<0.05 were considered statistically significant.
Results
IL-8 promotes tumor cell metastasis to the liver and lung in the HCCLM6 orthotopic HCC mouse model
To evaluate the effect of IL-8 on the metastasis of HCC cells in vivo, we established a mouse model of orthotopic HCC metastasis, and the successful mice were treated with normal saline or IL-8 respectively. As shown in Figure 1A-1C, the number of liver metastasis and lung metastasis in the IL-8 group was significantly higher than that in the control group (P<0.01). Moreover, flow cytometry analysis showed that IL-8 increased the population of CTCs in model mice (Figure 1D, P<0.01).
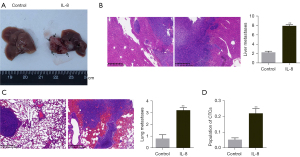
IL-8 promotes tumor cell metastasis to the liver and lung in vivo colonization mouse model
To further study the effect of IL-8 on metastatic colonization of HCC cells, we established a liver metastasis model and lung metastasis model respectively and treated mice with normal saline or IL-8 respectively. The liver metastases foci in liver metastasis model mice treated with IL-8 were significantly higher than that in liver metastasis model mice treated with normal saline (Figure 2A,2B, P<0.01). Similarly, the treatment of IL-8 can promote the increase of lung metastasis foci in lung metastasis model mice (Figure 2C, P<0.01).
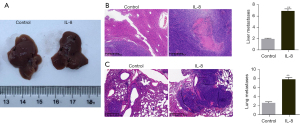
IL-8 promotes tumor cell metastasis to the liver and lung by increasing the expression of MMP9
We detected the expression of MMP9 in tumor tissues of mice with orthotopic HCC by qRT-PCR and found that the tumor tissues of mice in the IL-8 treatment group expressed more MMP9, compared with the control group (Figure 3A, P<0.01). The results of IHC also showed that the orthotopic HCC model mice treated with IL-8 expressed more MMP9 than the original HCC model mice treated with saline (Figure 3B, P<0.01). Based on these results, we administered IL-8 or IL-8 combined with MMP9 inhibitors to liver metastasis and lung metastasis model mice, respectively, to observe whether the metastasis-promoting effect of IL-8 is related to the expression of MMP9. As shown in Figure 3C-3E, MMP9 inhibitors significantly reversed IL-8’s promotion of tumor metastasis in liver metastasis model mice and lung metastasis model mice (P<0.01).
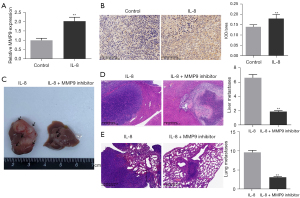
IL-8 promotes the expression of MMP9 in HCCLM6 cells through PKC/ERK1/2 signaling pathway
To explore the molecular mechanism of IL-8 regulating MMP9, we conducted cell experiments. Consistent with the results of animal experiments, IL-8 promoted the mRNA and protein levels of MMP9 in HCCLM6 cells, and the higher the concentration of IL-8, the higher the expression level of MMP9 (Figure 4A,4B, P<0.01). Then we selected 15 ng/mL IL-8 to treat HCCLM6 cells and found that with the increase of treatment time, the expression of p-PKC and p-ERK1/2 increased, while the expression of PKC and ERK1/2 decreased (Figure 4C). However, after using PKC inhibitor and ERK1/2 inhibitor, IL-8’s promotion of MMP9 mRNA and protein levels was reversed (Figure 4D,4E, P<0.05).
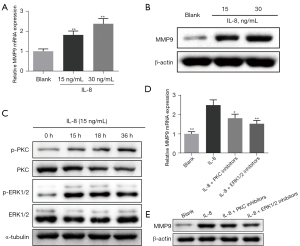
PKC inhibitor or ERK1/2 inhibitor reversed the effect of IL-8 on tumor metastasis in orthotopic HCC metastasis model mice
To verify that IL-8 promotes tumor metastasis through the PKC/ERK1/2 signaling pathway, we administered IL-8 combined with PKC inhibitors or ERK1/2 inhibitors to orthotopic HCC model mice. As shown in Figure 5A,5B, the number of liver and lung metastases in model mice injected with PKC Inhibitor and ERK1/2 inhibitor was significantly reduced (P<0.01). In addition, compared with the IL-8 group, the expression level of MMP9 in tumor tissues of the IL-8 + PKC inhibitor and IL-8 + EKR1/2 inhibitor group was also inhibited (Figure 5C,5D, P<0.05).
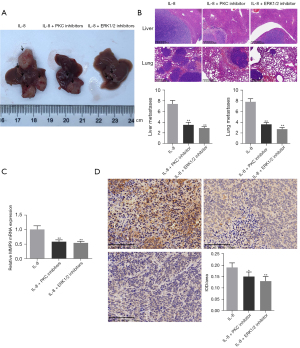
Discussion
Tumor metastasis is the main cause of death of various tumors. It can be divided into two major steps, namely, the spread of tumor cells from the site of cancer in situ to the remote tissues and the adaptation of the tumor cells to the microenvironment of the remote tissues and their successful colonization growth (11). Therefore, in this study, we constructed an orthotopic implantation model and in vivo colonization model, and conducted in vitro experiments to comprehensively explore the mechanism of IL-8 in the metastasis of HCC.
In our study, in the orthotopic implantation model, IL-8 not only significantly promoted the intrahepatic and lung metastasis of cancer cells but also increased the number of CTCs. CTCs refer to tumor cells that leave the primary tumor focus and enter the blood circulation and are also considered as precursors of various types of cancer metastasis (12). A large number of research results show that growth factors and chemokines [IL-8, vascular endothelial growth factor (VEGF) and basic fibroblast growth factor (bFGF)] secreted by tumor cells can induce the pre-metastatic organs of the primary tumor to form a pre-metastatic niche conducive to the spread and survival of tumor cells, thus promoting the colonization and proliferation of CTCs in the pre-metastatic organs, and finally forming metastatic lesions (13,14). On the other hand, CTCs can also be induced by IL-8 for “tumor self-seeding”, that is, they can be colonized in situ tumors and accelerate the growth of in situ tumors (15). In the colonization experiment, the liver metastasis and lung metastasis of mice injected with HCCLM6 cells through the spleen and tail vein were deepened after being treated with IL-8, which means that HCCLM6 cells escaped immune surveillance and completed colonization with the help of IL-8.
We found that IL-8 exerts a metastatic effect by up-regulating the expression of MMP9 in tumor tissues. MMP9 can cleave type IV collagen and gelatin, which facilitates the invasion of cancer cells (16). Therefore, Reis et al. used MMP9 and IL-8 as prognostic indicators for bladder cancer (17). The regulatory effect of IL-8 on MMP9 has been verified. Shiau et al. found that human papillomavirus (HPV) up-regulates MMP9 by inducing the expression of IL-8 in lung cancer cells, thereby promoting the metastasis of lung cancer cells (18). Jovanović et al. reported that IL-8 stimulated the migration and invasion of trophoblast cells by increasing the levels of MMP9 and integrins alpha5 and beta1 (19). In addition, Yu et al. showed that the activation of IL-8 in HCC can promote the formation of an inflammatory microenvironment, and stimulate the expression of downstream VEGF and MMP9, increasing the invasion potential of tumor cells (7). This meant that the regulation of MMP9 by IL-8 in HCC is likely to be related to the inflammatory tumor microenvironment.
IL-8 is a pro-inflammatory factor and a chemotactic factor of leukocytes, while neutrophils are the largest number of leukocytes in the blood. In addition, neutrophils are also the most abundant source of MMP9 in vivo. It is found that neutrophils recruited by IL-8 infiltrate into tumor tissues play a role in promoting tumor by enhancing the expression of MMP9, and recruiting new pro-inflammatory cells into the tumor microenvironment by releasing CXCL1 and CXCL6, thus forming an inflammatory tumor microenvironment (20,21). We noticed that Chakrabarti et al. found that IL-8 mediated MMP9 release from neutrophils through binding to CXCR2, which involved PKC/ERK1/2 signaling pathway (22). Therefore, we speculate that the up-regulation of MMP9 by IL-8 in HCC may also involve the activation of the PKC/ERK1/2 signaling pathway. PKC is an important kinase in cells, which can activate its downstream ERK signaling pathway by phosphorylation (23). MMP9 has also been proved to be a downstream effector protein of the ERK signaling pathway (24). Hah et al. reported that PKC-dependent ERK activation promoted the expression of MMP9 in HCC cells (25). In our study, the promoting effect of IL-8 on MMP9 expression was reversed by PKC inhibitor and ERK1/2 inhibitor, indicating that IL-8 regulates MMP9 expression via PKC/ERK1/2 signaling pathway.
Conclusions
In summary, this article showed that IL-8 upregulates the expression of MMP9 by activating PKC/ERK1/2 signaling pathway, thus promoting the metastasis and colonization of HCC cells. The results of this study may provide a new perspective on the pathogenesis of HCC metastasis.
Acknowledgments
Funding: None.
Footnote
Reporting Checklist: The authors have completed the ARRIVE reporting checklist. Available at https://tcr.amegroups.com/article/view/10.21037/tcr-22-37/rc
Data Sharing Statement: Available at https://tcr.amegroups.com/article/view/10.21037/tcr-22-37/dss
Conflicts of Interest: All authors have completed the ICMJE uniform disclosure form (available at https://tcr.amegroups.com/article/view/10.21037/tcr-22-37/coif). The authors have no conflicts of interest to declare.
Ethical Statement: The authors are accountable for all aspects of the work in ensuring that questions related to the accuracy or integrity of any part of the work are appropriately investigated and resolved. All the animal experiments were performed in strict accordance with the recommendations in the Guide for the Care and Use of Laboratory Animals by the National Institutes of Health and approved by the Ethics Committee of Experimental Animals of Zhejiang Chinese Medical University (approval No. ZSLL-2019-186).
Open Access Statement: This is an Open Access article distributed in accordance with the Creative Commons Attribution-NonCommercial-NoDerivs 4.0 International License (CC BY-NC-ND 4.0), which permits the non-commercial replication and distribution of the article with the strict proviso that no changes or edits are made and the original work is properly cited (including links to both the formal publication through the relevant DOI and the license). See: https://creativecommons.org/licenses/by-nc-nd/4.0/.
References
- Forner A, Reig M, Bruix J. Hepatocellular carcinoma. Lancet 2018;391:1301-14. [Crossref] [PubMed]
- Anwanwan D, Singh SK, Singh S, et al. Challenges in liver cancer and possible treatment approaches. Biochim Biophys Acta Rev Cancer 2020;1873:188314. [Crossref] [PubMed]
- Welling TH, Fu S, Wan S, et al. Elevated serum IL-8 is associated with the presence of hepatocellular carcinoma and independently predicts survival. Cancer Invest 2012;30:689-97. [Crossref] [PubMed]
- Deng Y, Ning Z, Hu Z, et al. High interleukin-8 and/or extracellular signal-regulated kinase 2 expression predicts poor prognosis in patients with hepatocellular carcinoma. Oncol Lett 2019;18:5215-24. [Crossref] [PubMed]
- Alfaro C, Sanmamed MF, Rodríguez-Ruiz ME, et al. Interleukin-8 in cancer pathogenesis, treatment and follow-up. Cancer Treat Rev 2017;60:24-31. [Crossref] [PubMed]
- Kubo F, Ueno S, Hiwatashi K, et al. Interleukin 8 in human hepatocellular carcinoma correlates with cancer cell invasion of vessels but not with tumor angiogenesis. Ann Surg Oncol 2005;12:800-7. [Crossref] [PubMed]
- Yu J, Ren X, Chen Y, et al. Dysfunctional activation of neurotensin/IL-8 pathway in hepatocellular carcinoma is associated with increased inflammatory response in microenvironment, more epithelial mesenchymal transition in cancer and worse prognosis in patients. PLoS One 2013;8:e56069. [Crossref] [PubMed]
- Zarogoulidis P, Katsikogianni F, Tsiouda T, et al. Interleukin-8 and interleukin-17 for cancer. Cancer Invest 2014;32:197-205. [Crossref] [PubMed]
- Scheau C, Badarau IA, Costache R, et al. The Role of Matrix Metalloproteinases in the Epithelial-Mesenchymal Transition of Hepatocellular Carcinoma. Anal Cell Pathol (Amst) 2019;2019:9423907. [Crossref] [PubMed]
- Yamauchi K, Yang M, Hayashi K, et al. Induction of cancer metastasis by cyclophosphamide pretreatment of host mice: an opposite effect of chemotherapy. Cancer Res 2008;68:516-20. [Crossref] [PubMed]
- Valastyan S, Weinberg RA. Tumor metastasis: molecular insights and evolving paradigms. Cell 2011;147:275-92. [Crossref] [PubMed]
- Rossi E, Fabbri F. CTCs 2020: Great Expectations or Unreasonable Dreams. Cells 2019;8:989. [Crossref] [PubMed]
- Yang WW, Yang LQ, Zhao F, et al. Epiregulin Promotes Lung Metastasis of Salivary Adenoid Cystic Carcinoma. Theranostics 2017;7:3700-14. [Crossref] [PubMed]
- Ji Q, Zhou L, Sui H, et al. Primary tumors release ITGBL1-rich extracellular vesicles to promote distal metastatic tumor growth through fibroblast-niche formation. Nat Commun 2020;11:1211. [Crossref] [PubMed]
- Kim MY, Oskarsson T, Acharyya S, et al. Tumor self-seeding by circulating cancer cells. Cell 2009;139:1315-26. [Crossref] [PubMed]
- Wang Q, Yu W, Huang T, et al. RUNX2 promotes hepatocellular carcinoma cell migration and invasion by upregulating MMP9 expression. Oncol Rep 2016;36:2777-84. [Crossref] [PubMed]
- Reis ST, Leite KR, Piovesan LF, et al. Increased expression of MMP-9 and IL-8 are correlated with poor prognosis of Bladder Cancer. BMC Urol 2012;12:18. [Crossref] [PubMed]
- Shiau MY, Fan LC, Yang SC, et al. Human papillomavirus up-regulates MMP-2 and MMP-9 expression and activity by inducing interleukin-8 in lung adenocarcinomas. PLoS One 2013;8:e54423. [Crossref] [PubMed]
- Jovanović M, Stefanoska I, Radojcić L, et al. Interleukin-8 (CXCL8) stimulates trophoblast cell migration and invasion by increasing levels of matrix metalloproteinase (MMP)2 and MMP9 and integrins alpha5 and beta1. Reproduction 2010;139:789-98. [Crossref] [PubMed]
- Giese MA, Hind LE, Huttenlocher A. Neutrophil plasticity in the tumor microenvironment. Blood 2019;133:2159-67. [Crossref] [PubMed]
- Dymicka-Piekarska V, Koper-Lenkiewicz OM, Zińczuk J, et al. Inflammatory cell-associated tumors. Not only macrophages (TAMs), fibroblasts (TAFs) and neutrophils (TANs) can infiltrate the tumor microenvironment. The unique role of tumor associated platelets (TAPs). Cancer Immunol Immunother 2021;70:1497-510. [Crossref] [PubMed]
- Chakrabarti S, Patel KD. Regulation of matrix metalloproteinase-9 release from IL-8-stimulated human neutrophils. J Leukoc Biol 2005;78:279-88. [Crossref] [PubMed]
- Tsai JY, Rédei D, Hohmann J, et al. 12-Deoxyphorbol Esters Induce Growth Arrest and Apoptosis in Human Lung Cancer A549 Cells Via Activation of PKC-δ/PKD/ERK Signaling Pathway. Int J Mol Sci 2020;21:7579. [Crossref] [PubMed]
- Marchese V, Juarez J, Patel P, et al. Density-dependent ERK MAPK expression regulates MMP-9 and influences growth. Mol Cell Biochem 2019;456:115-22. [Crossref] [PubMed]
- Hah N, Lee ST. An absolute role of the PKC-dependent NF-kappaB activation for induction of MMP-9 in hepatocellular carcinoma cells. Biochem Biophys Res Commun 2003;305:428-33. [Crossref] [PubMed]