MicroRNA expression profiles in extracellular vesicles and intracellular of AURKA inhibitor-induced senescent neuroblastoma cells
Introduction
Therapy induced senescence (TIS), a durable form of growth arrest, represents a primary response of tumor cells to numerous anticancer therapies. TIS is considered as one of the mechanisms by which chemotherapeutic agents or radiation control cancer progression in clinical practice (1). Nevertheless, after ceasing division and entering senescence, cells do not actually die. Some senescent tumor cells were reprogrammed under drug pressure, senescent escape occurred, cells re-entered the cell cycle and activated the expression of tumor metastasis infiltration-related genes, promoting tumor recurrence and metastasis (2). Senescent cells also develop distinctive metabolic and signaling features, referred to as the senescence-associated secretory phenotype (SASP). Through various proteins, such as inflammatory cytokines and chemokines, SASP can stimulate the immune system and limit tumor growth. On the other hand, SASP can also promote cancer development through the release of matrix metalloproteinases into the extra-cellular space to alter the cellular microenvironment (3). In addition to secretory proteins, senescent cells also secret small extracellular vehicles (EVs) (4). Increasing evidence suggests that EVs secreted from senescent cells have unique characteristics and contribute to modulating the phenotype of recipient cells similar with SASP factors. Thus, the EVs secreted from senescent cells, namely, senescence-associated EVs (SAEVs), appear to be a novel SASP factor (5). Through SASP and SAEVs, senescent cells communicate with the extracellular environment and change the clinical outcome of the tumor. And as a membranous pouch, EVs function as a mode of intercellular communication and molecular transfer to facilitate the direct extracellular transfer of proteins, lipids, DNAs, messenger RNAs (mRNAs), and microRNAs (miRNAs) between cells and extracellular environment (6).
MiRNAs are a class of short noncoding RNAs that play key roles in almost all biological pathways in mammalian and other organisms (7,8). Recently, miRNAs have even been regarded as hormones to regulate cell-to-cell communication (9). And as highly sensitive to environmental stress, miRNAs encapsulated in secreted EVs disseminate through the extracellular fluid to reach remote target cells to remodel local microenvironment by regulating mRNA and protein expression either as tumor suppressors or stimulators, depending on their targets (10).
MLN8237 (also named Alisertib), an orally administered selective small molecule inhibitor of aurora kinase A (AURKA), has been shown to reduce tumor growth in preclinical and clinical studies (11). On the mechanism, MLN8237 significantly impairs mitotic progression through activation of the mitotic checkpoint, causing abnormal spindle formation, mitotic defects, cell cycle arrest at G2/M phase, and cell senescence. In our previous study, we compared the effect of different chemotherapy agents on the senescence of neuroblastoma cells and found that 0.5 µmol/L of MLN8237 induced almost 90% of neuroblastoma IMR32 cells to enter the state of senescence (12).
Herein, we take advantage of MLN8237 to treat IMR32 as a senescent cell model to delineate the change of miRNA profile in MLN8237-induced cellular senescence. MiRNAs in senescent IMR32 cells and extracellular vesicles from cell supernatant were extracted for deep sequencing. The differentially expressed miRNAs were ranked according to their expression level. The expression level of several miRNAs was verified by quantitative reverse transcription polymerase chain reaction (qRT-PCR). The possible roles of up-regulated miRNAs in senescent cells and secreted EVs were evaluated according to the existing literature. The analysis of miRNA expression profile in senescent cells is beneficial to understand the mechanism of MLN8237-induced senescence. Uncovering the communication between senescent cells and the external environment from the perspective of miRNA provides some clues for understanding tumor recurrence and metastasis after chemotherapy. We present the following article in accordance with the MDAR reporting checklist (available at https://tcr.amegroups.com/article/view/10.21037/tcr-21-2438/rc).
Methods
Cell culture and reagents
Human neuroblastoma cell line of IMR32: RRID: CVCL_0346 were cultured in high glucose Dulbecco’s modified Eagle’s medium (Gibco, USA), supplemented with 10% (v/v) fetal bovine serum (Gibco), and 100 µg/mL penicillin/streptomycin (Invitrogen, USA) at 37 ℃ incubator plus 5% CO2. Chemotherapeutics of AURKA inhibitor, MLN8237, was purchased from MedChem Express (MCE, USA). Other reagents are commercially available.
Senescence-associated β-galactosidase (SA-β-gal) staining assay
An increased level of lysosomal β-galactosidase activity is a classic marker of cellular senescence. Under normal growth condition cells produce acid lysosomal β-galactosidase. Upon senescence, the lysosomal mass is increased, leading to production of a higher level of β-galactosidase, termed SA-β-gal. The enzymatic activity can be detected by using the chromogenic substrate 5-bromo-4-chloro-3-indolyl β D-galactopyranoside (X-gal). IMR32 cells were treated with 0.5 µmol/L of MLN8237 for 72 hours followed by staining using Cellular Senescence Detection Kit (Genmed Scientifics, China). The SA-β-gal positive cells were stained blue green, which can be observed under bright-field microscopy.
Senescence-associated heterochromatic foci (SAHF)
Senescence is also often accompanied by a global change in nuclear architecture, exemplified by the formation of SAHF. To clearly show SAHF, IMR32 cells were stained with 4',6'-diamidino-2-phenylindole (DAPI) and H2A.X antibody (GTX127340, GeneTex, USA) after treated with 0.5 µmol/L of MLN8237 for 72 hours. The results were observed by fluorescent microscopy.
Quantitative real-time RT-PCR
SASP is another important marker of cellular senescence. We detected the expression level of IL-6 and IL-8, two kinds of inflammatory factors of SASP. IMR32 cells were collected after treated with 0.5 µmol/L of MLN8237 for 72 hours. The total RNAs were extracted using TRIzol reagent (Invitrogen) and complementary DNAs (cDNA) were synthesized with random hexamers (Takara, Japan). Real-time quantitative PCR (qPCR) was carried out using SYBR Premix Ex Taq Kit (Takara) under the following conditions: denaturation at 94 ℃ for 1 min and amplification by cycling 40 times at 94 ℃ for 30 s, 60 ℃ for 30 s, and 72 ℃ for 45 s. A housekeeping gene encoding GAPDH was amplified to normalize target gene’s copy numbers. PCR primer sequences were shown in Table 1.
Table 1
Primer | Sequence (5' to 3') |
---|---|
IL-6 forward | GTCCAGCCTGAGGGCTCTTC |
IL-6 reverse | TCTGTGCCCAGTGGACAGGT |
IL-8 forward | GTGAAGGTGCAGTTTTGCCA |
IL-8 reverse | TCTCCACAACCCTCTGCAC |
GAPDH forward | TCCCTGAGCTGAACGGGAAG |
GAPDH reverse | GGAGGATGGGTGTCGCTGT |
RT-PCR, reverse transcription polymerase chain reaction.
As for miRNA verification, quantitative RT-PCR was also adopted. Firstly, reverse transcribed to cDNA using PrimeScriptTM RT Master Mix (Perfect Real Time) (Takara). The TB Green® Fast qPCR Mix (Takara) was used in qPCR to determine the expression of miRNA. U6 was chosen as reference gene. The primers of miRNAs were designed and synthesis by RIBOBIO Company. qPCR program was performed in Roche 480 II as follows: 95 ℃ for 2 min; 40 cycles of 95 ℃ for 10 s, 68 ℃ for 10 s, and then 72 ℃ for 30 s.
The relative expression level of mRNA or miRNA was calculated using the comparative 2−∆Ct (∆Ct = Cttarget gene − Ctreference gene). Fold change values were calculated using the comparative 2−∆∆Ct, in which ∆∆Ct = ∆Ct (MLN8237-treated group) − ∆Ct (untreated group). Real-time RT-PCR was performed in triplicate for each RNA sample and parallel experiments were carried out three times.
Cell samples preparation and EVs isolation and identification
IMR32 cells were routinely cultured in DMEM medium supplemented with 10% EVs-depleted FBS. When the cells grow to the logarithmic growth stage, the population of cells was divided into two distinct groups: the MLN8237-treated group and the no-treated group. The MLN8237-treated group was designated as MC, and the no-treated group as C. After 72 hours incubation, cell supernatants of both groups were collected for EVs isolation and cells were lysed with TRIzol for RNA extraction.
To isolate EVs, ultracentrifugation was performed. In detail, supernatants were centrifuged at 10,000 ×g for 30 min at 4 ℃ followed by filtered using a 0.22 µm pore filter to remove cells and large debris. Afterwards, EVs were pelleted at 100,000 ×g for 1 h at 4 ℃. Then, the pellets were washed with 10 mL of 1× phosphate-buffered saline (PBS) and pelleted again by centrifugation at 100,000 ×g for 1 h at 4 ℃. The resulting pellets were suspended in 1× PBS for EVs identification by western blot with primary antibodies of tumor susceptibility gene 101 (TSG101; ab8245448, Abcam, USA), glucose-regulated protein 78 (GRP78; ab229317, Abcam), and β-actin (ab8227, Abcam).
The size and concentration of EVs were tested by Nanosight (Nanosight LM10, Malvern). The group of EVs from treated-cell supernatant was designated as ME, and the notreatment control group as E. Samples were obtained from three separate experiments. Finally, 12 samples (3 MC, 3 C, 3 ME, and 3 E) were collected and stored at −80 ℃ for RNA extraction.
Small RNA library construction and deep sequencing
Total RNAs were extracted from IMR32 cells or EVs pellets and purified using the TRIzol reagent (Invitrogen/Life Technologies, USA) according to the manufacturer instructions. The quality and concentration of all twelve RNA samples were determined by 1.5% agarose gel electrophoresis and quantitated using the NanoDrop Spectrophotometer. Subsequently, 20 µg of total RNA were purified by denaturing 15% PAGE to enrich for 18–30 nt small RNAs, and then ligated with proprietary adapters. The ligated products were purified, and reverse transcribed into cDNA to produce libraries. Ultimately, the libraries were deep sequenced using BGISEQ-500 (BGI-Tech, Huada) at Huada Biotechnology Co., Ltd. (Shenzhen, China).
Analysis of sequence and differentially expressed miRNAs
Forty-nine nucleotide long sequence tags from sequencing will go through the data cleaning analysis, which includes getting rid of the low-quality tags, 5'-adaptor contaminants from the 50 nt tags, to get credible clean tags. Normally, the length of small RNA is between 18 and 30 nt. Then the length distribution of the clean tags and common and specific sequences among samples will be summarized. Then the standard analysis will annotate the clean tags into different categories. We defined a gene as a differentially expressed gene (DEG) when reads number fold change ≥2 (up-regulation) or ≤−2 (down-regulation), P value ≤0.05, and Q value ≤0.001. Hierarchical clustering for differentially expressed miRNAs was performed by using heatmap.
Target gene prediction, function annotation and network analysis
In order to find the possible targets to differentially expressed miRNAs, multiple softwares including miRanda and TargetScan were employed. Only the target genes predicted by both of two methods were considered as reliable targets for further analysis. Gene Ontology (GO), an international standard gene functional classification system, was used for gene functional annotation. Pathway-based analysis helps to further understand differentially expressed small RNAs (DESs) target genes biological functions. Kyoto Encyclopedia of Genes and Genomes (KEGG) (the major public pathway-related database) is used to perform pathway enrichment analysis.
Statistical analysis
The data are representative of three independent experiments which were presented as the mean ± standard deviation (SD). Statistical analysis was performed using SPSS v.12.0. The unpaired two-tailed Student’s t-test was used to perform statistical comparison between two groups. Analysis of variance (ANOVA) was used for multiple comparisons. P<0.05 was considered to indicate statistical significance.
Ethical statement
The study was conducted in accordance with the Declaration of Helsinki (as revised in 2013).
Results
Establishment of a cell senescence model
An enlarged and flattened morphology with an increased activity of β-galactosidase in cells is a prominent feature of senescent cells which tested by SA-β-gal staining. The blue-stained cells in Figure 1A are senescent neuroblastoma cells. SAHF, another feature of senescent cells, was observed in MLN8237-treated cells by using H2A.X antibody incubation and DAPI dyes staining, shown as Figure 1B, in which the nuclei of MLN8237-treated cells were enlarged with dispersed green spots. To observe the SASP of MLN8237-treated IMR32 cells, we detected the expression level of IL-6 and IL-8 factors by qRT-PCR. As shown in Figure 1C, from the first day after MLN8237 treatment to the sixth day, mRNA levels of IL-6 and IL-8 presented a pronounced increase. MLN8237 induced cell cycle arrest at G2/M phase and cell growth inhibition in vitro and in vivo have been confirmed previously (13). In a word, neuroblastoma cells of IMR32 can be induced into senescent state under 0.5 µmol/L of MLN8237 treatment to develop a senescent cell model.
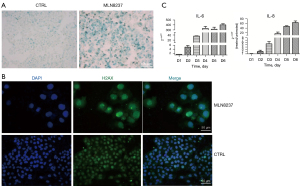
SAEVs preparation and identification
TSG101 is a membrane protein commonly used as a marker of EVs. The 78 kDa GRP78 is constitutively expressed in the ER lumen which is thought to be a marker protein for the localization of cytoplasm. Western blotting result indicated that TSG101 was present in both cell lysates and EVs, while GRP78 was nearly invisible in EVs samples (Figure 2A). The size and concentration of EVs were determined by Nanosight as shown in Figure 2B and Table 2. There was no statistically significant difference in the average particle size of EVs secreted by senescent cells (SAEVs) and control cells (EVs). The total concentration of SAEVs was significantly reduced, which may be connected to the fact that senescent cells ceased dividing and the number of cells was significantly lower than control cells. As can be seen from Table 2, the percentage of SAEVs particles with a particle size of 30–150 nm was higher than that of EVs, that is, the concentration of exosomes in SAEVs was higher than that in EVs. It means MLN8237 treatment reduced the total number of EVs. Whereas increased the magnitude of particles at 30–150 nm, of which represents exosomes.
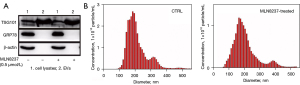
Table 2
Sample | EVs | SAEVs |
---|---|---|
Mean particle size (nm) | 218.6 | 213.8 |
Particle size peak (nm) | 187.4 | 168.7 |
Total concentration (1×1011 particle/mL) | 2.935 | 1.52 |
Particle size 30–150 nm percentage (%) | 5.8 | 16.1 |
EVs, extracellular vehicles; SAEVs, senescence-associated extracellular vehicles.
Identification of differentially expressed miRNAs in senescent cells and SAEVs
In this study, a total 5,321 miRNA (4,184 known mature miRNAs and 1,137 novel miRNAs) were detected. On average, there were 1,323, 1,358, 718, and 785 known miRNAs in C, MC, E, and ME group, respectively (Table 3). The calculated gene expression can be directly used for comparing the difference of gene expression among samples. The average DEGs in each pairwise as shown in Figure 3A and hierarchical clustering of C-vs.-MC and E-vs.-ME was performed with DEGs as shown in Figure 3B. The results showed that MLN8237 treatment resulted in the increase of down-regulated miRNAs in cells and up-regulated miRNAs in EVs (Figure 3A). The number of significant differently expressed known miRNAs is 13 (up-regulation in MC vs. C, Table 4), 32 (down-regulation in MC vs. C, Table 5), and 48 (up-regulation in ME vs. E, Table 6), and 9 (down-regulation in ME vs. E, Table 7), respectively. The upregulated miRNAs in senescent cells include miR-378b, miR-206, miR-1-3p, miR-378g, miR-184, miR-3591-5p, miR-375, miR-4532, miR-7641, miR-199b-3p, miR-4492, miR-4485-3p, and miR-3656. Heat maps were made for some representative miRNAs involved in senescent cells shown in Figure 4.
Table 3
Sample name | Known miRNA count | Novel miRNA count | Known piRNA count | Novel piRNA count | Known siRNA count | Novel siRNA count |
---|---|---|---|---|---|---|
C_1A | 1,318 | 162 | 160 | 984 | 0 | 0 |
C_2A | 1,316 | 208 | 160 | 817 | 0 | 0 |
C_3A | 1,335 | 195 | 175 | 638 | 0 | 0 |
MC_1A | 1,436 | 174 | 170 | 770 | 0 | 0 |
MC_2A | 1,430 | 199 | 184 | 649 | 0 | 0 |
MC_3A | 1,210 | 140 | 186 | 1396 | 0 | 0 |
E_1A | 749 | 295 | 77 | 361 | 0 | 0 |
E_2A | 644 | 344 | 76 | 507 | 0 | 0 |
E_3A | 761 | 404 | 82 | 1,562 | 0 | 0 |
ME_1A | 781 | 310 | 92 | 479 | 0 | 0 |
ME_2A | 752 | 176 | 100 | 318 | 0 | 0 |
ME_3A | 822 | 807 | 104 | 687 | 0 | 0 |
miRNAs, microRNA; piRNA, PIWI-interacting RNA; siRNA, small interfering RNA.
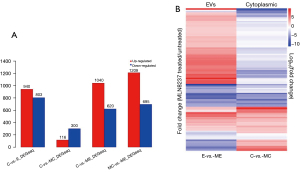
Table 4
miRNA ID | Read count (C) | Read count (MC) | Expression (C) | Expression (MC) | Log2ratio (MC/C) | Up or down | P value | Q value |
---|---|---|---|---|---|---|---|---|
Hsa-miR-378b | 88 | 39,920 | 1.446666667 | 561.8833333 | 8.621042314 | Up | 0 | 0 |
Hsa-miR-206 | 3 | 1,124 | 0.077333333 | 14.94033333 | 8.345113652 | Up | 8.43E-214 | 4.68E-213 |
Hsa-miR-1-3p | 3 | 681 | 0.053333333 | 9.06 | 7.62219832 | Up | 1.49E-139 | 6.61E-139 |
Hsa-miR-378g | 226 | 6,542 | 3.456666667 | 87.03 | 4.650986914 | Up | 0 | 0 |
Hsa-miR-184 | 20 | 375 | 0.356666667 | 5.25 | 4.024468523 | Up | 1.92E-75 | 7.12E-75 |
Hsa-miR-3591-5p | 93 | 1,351 | 1.406666667 | 18.87666667 | 3.656302981 | Up | 3.64E-250 | 2.35E-249 |
Hsa-miR-375 | 120 | 1,419 | 2.02 | 19.72666667 | 3.359418111 | Up | 6.80E-246 | 4.20E-245 |
Hsa-miR-4532 | 5,153 | 54,574 | 86.09333333 | 735.1433333 | 3.200379125 | Up | 0 | 0 |
Hsa-miR-7641 | 51 | 312 | 0.796666667 | 4.183333333 | 2.40862671 | Up | 3.20E-40 | 9.61E-40 |
Hsa-miR-199b-3p | 2,459 | 13,351 | 51.07666667 | 186.4066667 | 2.236453998 | Up | 0 | 0 |
Hsa-miR-4492 | 34 | 181 | 0.64 | 2.426666667 | 2.208032878 | Up | 8.21E-22 | 1.90E-21 |
Hsa-miR-4485-3p | 249 | 1,261 | 4 | 17.58 | 2.136000461 | Up | 6.41E-136 | 2.76E-135 |
Hsa-miR-3656 | 323 | 1,582 | 5.65 | 21.30666667 | 2.087793362 | Up | 3.27E-165 | 1.58E-164 |
Only miRNAs with a ratio greater than 2 were listed. miRNAs, microRNA.
Table 5
miRNA ID | Read count (C) | Read count (MC) | Expression (C) | Expression (MC) | Log2ratio (MC/C) | Up or down | P value | Q value |
---|---|---|---|---|---|---|---|---|
Hsa-miR-205-5p | 752 | 1 | 10.83666667 | 0.014 | −9.758939019 | Down | 8.41E-139 | 3.71E-138 |
Hsa-miR-378d | 22,264 | 214 | 372.66 | 3.026666667 | −6.905308374 | Down | 0 | 0 |
Hsa-miR-378f | 1,013 | 24 | 24.65 | 0.323333333 | −5.603806125 | Down | 2.02E-258 | 1.34E-257 |
Hsa-miR-365b-3p | 16,840 | 1,064 | 382.17 | 14.9 | −4.18867225 | Down | 0 | 0 |
Hsa-miR-378i | 1,706 | 154 | 24.59333333 | 2.126666667 | −3.673965558 | Down | 0 | 0 |
Hsa-miR-33b-5p | 547 | 56 | 12.65333333 | 0.786666667 | −3.492392268 | Down | 8.14E-114 | 3.33E-113 |
Hsa-miR-548am-5p | 173 | 26 | 3.236666667 | 0.37 | −2.938538677 | Down | 3.48E-32 | 9.57E-32 |
Hsa-miR-107 | 2,720 | 412 | 49.53333333 | 5.76 | −2.927240576 | Down | 0 | 0 |
Hsa-miR-199a-3p | 9,488 | 1,564 | 137.1866667 | 21.89333333 | −2.805213665 | Down | 0 | 0 |
Hsa-miR-378e | 15,916 | 2,680 | 226.8533333 | 37.18 | −2.774523066 | Down | 0 | 0 |
Hsa-miR-190a-3p | 161 | 28 | 3.213333333 | 0.39 | −2.727912123 | Down | 5.37E-28 | 1.37E-27 |
Hsa-miR-1307-5p | 2,394 | 431 | 50.08666667 | 6.123333333 | −2.678013545 | Down | 0 | 0 |
Hsa-miR-20a-3p | 657 | 126 | 14.42333333 | 1.766666667 | −2.586819804 | Down | 7.63E-103 | 2.99E-102 |
Hsa-miR-876-5p | 197 | 38 | 4.586666667 | 0.533333333 | −2.578474473 | Down | 5.57E-32 | 1.52E-31 |
Hsa-miR-20b-5p | 139 | 27 | 3.2 | 0.38 | −2.568403738 | Down | 5.88E-23 | 1.41E-22 |
Hsa-miR-152-5p | 106 | 21 | 2.2 | 0.29 | −2.539953199 | Down | 1.11E-17 | 2.39E-17 |
Hsa-miR-196a-3p | 186 | 37 | 4.146666667 | 0.52 | −2.534055613 | Down | 9.69E-30 | 2.53E-29 |
Hsa-miR-101-3p | 5,670 | 1,143 | 129.5633333 | 16.00666667 | −2.514873499 | Down | 0 | 0 |
Hsa-miR-30e-5p | 6,281 | 1,390 | 140.1466667 | 19.49 | −2.380259554 | Down | 0 | 0 |
Hsa-miR-190a-5p | 4,150 | 922 | 71.18333333 | 12.85333333 | −2.374622848 | Down | 0 | 0 |
Hsa-miR-19a-3p | 8,836 | 1,986 | 208.19 | 27.77666667 | −2.357877963 | Down | 0 | 0 |
Hsa-miR-362-3p | 729 | 165 | 15.85 | 2.32 | −2.347802957 | Down | 3.92E-102 | 1.53E-101 |
Hsa-miR-4286 | 427 | 101 | 7.866666667 | 1.426666667 | −2.284230944 | Down | 1.06E-58 | 3.54E-58 |
Hsa-miR-19b-1-5p | 1,145 | 276 | 24.86 | 3.86 | −2.256957594 | Down | 5.36E-152 | 2.48E-151 |
Hsa-miR-873-5p | 527 | 128 | 11.72 | 1.786666667 | −2.246009319 | Down | 1.36E-70 | 4.91E-70 |
Hsa-miR-590-5p | 1,096 | 281 | 25.04666667 | 3.933333333 | −2.16795593 | Down | 1.25E-138 | 5.49E-138 |
Hsa-miR-374a-5p | 17,073 | 4,403 | 352.93 | 61.32333333 | −2.159508003 | Down | 0 | 0 |
Hsa-miR-29b-3p | 14,471 | 3,758 | 319.3666667 | 52.75333333 | −2.149477816 | Down | 0 | 0 |
Hsa-miR-452-5p | 2,585 | 684 | 55.73333333 | 9.58 | −2.122446218 | Down | 0 | 0 |
Hsa-miR-148b-5p | 167 | 45 | 3.676666667 | 0.63 | −2.096201363 | Down | 9.90E-22 | 2.29E-21 |
Hsa-miR-1268b | 1,515 | 417 | 31.79 | 5.843333333 | −2.065548672 | Down | 1.26E-179 | 6.27E-179 |
Hsa-miR-551b-3p | 4,212 | 1,210 | 93.85666667 | 16.95333333 | −2.003848556 | Down | 0 | 0 |
Only miRNAs with a ratio of less than 2 were listed. miRNAs, microRNA.
Table 6
miRNA ID | Read count (E) | Read count (ME) | Expression (E) | Expression (ME) | Log2ratio (ME/E) | Up or down | P value | Q value |
---|---|---|---|---|---|---|---|---|
Hsa-miR-205-5p | 1 | 804 | 0.030666667 | 13.49366667 | 9.786761203 | Up | 4.63E-144 | 1.49E-143 |
Hsa-miR-124-3p | 16 | 1,829 | 0.440333333 | 46.57366667 | 6.972548872 | Up | 0 | 0 |
Hsa-miR-363-3p | 4 | 409 | 0.093666667 | 10.42333333 | 6.811666545 | Up | 3.69E-100 | 9.88E-100 |
Hsa-miR-378i | 4 | 301 | 0.073666667 | 8.417 | 6.369329189 | Up | 1.33E-75 | 3.15E-75 |
Hsa-miR-20b-5p | 3 | 119 | 0.063666667 | 3.033666667 | 5.445564775 | Up | 3.75E-31 | 6.05E-31 |
Hsa-miR-155-5p | 33 | 1,149 | 0.896666667 | 29.277 | 5.257478475 | Up | 1.83E-283 | 8.22E-283 |
Hsa-miR-378f | 82 | 2,763 | 1.683333333 | 58.667 | 5.210177354 | Up | 0 | 0 |
Hsa-miR-146b-5p | 37 | 968 | 0.83 | 24.76666667 | 4.845119384 | Up | 2.38E-234 | 9.53E-234 |
Hsa-miR-199a-5p | 22 | 508 | 0.513333333 | 12.53333333 | 4.66496258 | Up | 2.44E-122 | 7.43E-122 |
Hsa-miR-362-5p | 4 | 91 | 0.070666667 | 2.306666667 | 4.643504152 | Up | 2.61E-23 | 3.59E-23 |
Hsa-miR-374c-3p | 15 | 338 | 0.323666667 | 8.583333333 | 4.629698353 | Up | 8.26E-82 | 2.06E-81 |
Hsa-miR-142-3p | 122 | 2,229 | 3.203333333 | 56.74666667 | 4.327153076 | Up | 0 | 0 |
Hsa-miR-150-5p | 1248 | 19,320 | 30.89333333 | 492.57 | 4.088114767 | Up | 0 | 0 |
Hsa-miR-103b | 76 | 1,156 | 1.986666667 | 28.56666667 | 4.062707681 | Up | 7.43E-258 | 3.06E-257 |
Hsa-miR-126-5p | 64 | 907 | 1.673333333 | 22.93666667 | 3.960668253 | Up | 1.11E-199 | 4.19E-199 |
Hsa-miR-103a-3p | 105 | 1,234 | 2.36 | 31.54666667 | 3.690590674 | Up | 2.11E-258 | 8.80E-258 |
Hsa-miR-199b-3p | 118 | 1,279 | 2.916666667 | 32.44333333 | 3.573867012 | Up | 1.70E-261 | 7.21E-261 |
Hsa-miR-30e-5p | 13 | 110 | 0.316666667 | 2.67 | 3.216629507 | Up | 3.01E-22 | 4.00E-22 |
Hsa-miR-148b-3p | 28 | 232 | 0.633333333 | 5.693333333 | 3.186335585 | Up | 1.05E-44 | 2.00E-44 |
Hsa-miR-29b-3p | 26 | 211 | 0.483333333 | 4.56 | 3.156368983 | Up | 1.74E-40 | 3.17E-40 |
Hsa-miR-374a-5p | 14 | 110 | 0.276666667 | 2.75 | 3.109714304 | Up | 1.26E-21 | 1.62E-21 |
Hsa-miR-449a | 20 | 153 | 0.44 | 2.78 | 3.07116926 | Up | 4.00E-29 | 6.07E-29 |
Hsa-miR-3613-5p | 116 | 849 | 2.6 | 21.26333333 | 3.007349261 | Up | 1.82E-150 | 6.05E-150 |
Hsa-miR-200c-3p | 101 | 711 | 2.206666667 | 13.52666667 | 2.951203779 | Up | 4.36E-124 | 1.34E-123 |
Hsa-miR-140-5p | 129 | 891 | 2.76 | 22.19666667 | 2.923763878 | Up | 1.34E-153 | 4.56E-153 |
Hsa-miR-28-5p | 247 | 1,593 | 5.853333333 | 40.15666667 | 2.824872832 | Up | 8.83E-264 | 3.85E-263 |
Hsa-miR-9-5p | 44 | 283 | 1.016666667 | 5.353333333 | 2.820936136 | Up | 2.30E-48 | 4.47E-48 |
Hsa-miR-26a-5p | 13,243 | 77,782 | 314.1466667 | 1964.033333 | 2.689915863 | Up | 0 | 0 |
Hsa-miR-203a-3p | 23 | 135 | 0.623666667 | 2.29 | 2.688963153 | Up | 7.31E-23 | 9.95E-23 |
Hsa-miR-223-5p | 28 | 160 | 0.633333333 | 4.120333333 | 2.650282685 | Up | 2.09E-26 | 3.05E-26 |
Hsa-miR-9-3p | 18 | 102 | 0.396666667 | 2.510333333 | 2.638209853 | Up | 2.48E-17 | 2.86E-17 |
Hsa-miR-223-3p | 1,996 | 11,151 | 48.10666667 | 286.1366667 | 2.61769898 | Up | 0 | 0 |
Hsa-miR-590-3p | 47 | 250 | 1.07 | 6.186666667 | 2.546904945 | Up | 1.16E-38 | 2.07E-38 |
Hsa-miR-26b-3p | 17 | 89 | 0.406666667 | 2.243333333 | 2.523980102 | Up | 1.17E-14 | 1.24E-14 |
Hsa-miR-30b-5p | 159 | 805 | 3.46 | 20.03666667 | 2.47567153 | Up | 1.07E-116 | 3.01E-116 |
Hsa-miR-454-3p | 55 | 277 | 1.22 | 6.773333333 | 2.468091965 | Up | 3.21E-41 | 5.93E-41 |
Hsa-miR-106b-5p | 39 | 195 | 0.78 | 4.75 | 2.457637607 | Up | 2.19E-29 | 3.36E-29 |
Hsa-miR-26b-5p | 2,451 | 12,123 | 59.76333333 | 303.3766667 | 2.44201388 | Up | 0 | 0 |
Hsa-miR-135b-5p | 40 | 193 | 0.833333333 | 4.44 | 2.406238454 | Up | 1.94E-28 | 2.93E-28 |
Hsa-miR-27a-5p | 25 | 119 | 0.516666667 | 2.8 | 2.386671086 | Up | 5.43E-18 | 6.44E-18 |
Hsa-miR-340-5p | 76 | 351 | 2.03 | 8.856666667 | 2.343109219 | Up | 8.03E-49 | 1.57E-48 |
Hsa-miR-214-3p | 38 | 160 | 0.71 | 4.033333333 | 2.209710094 | Up | 1.08E-21 | 1.40E-21 |
Hsa-miR-126-3p | 3,157 | 13,189 | 80.12666667 | 335.2633333 | 2.198418529 | Up | 0 | 0 |
Hsa-miR-142-5p | 127 | 514 | 3.436666667 | 13.07 | 2.152649375 | Up | 6.98E-64 | 1.53E-63 |
Hsa-miR-186-5p | 104 | 395 | 2.323333333 | 9.136666667 | 2.060978637 | Up | 6.05E-47 | 1.17E-46 |
Hsa-miR-3529-3p | 801 | 3,025 | 18.48 | 72.67333333 | 2.052770507 | Up | 0 | 0 |
Hsa-miR-940 | 34 | 128 | 0.766666667 | 2.736666667 | 2.048246671 | Up | 3.39E-16 | 3.77E-16 |
Hsa-miR-31-5p | 293 | 1,092 | 6.656666667 | 21.95666667 | 2.033709799 | Up | 2.22E-124 | 6.92E-124 |
Only miRNAs with a ratio greater than 2 were listed. miRNAs, microRNA.
Table 7
miRNA ID | Read count (E) | Read count (ME) | Expression (E) | Expression (ME) | Log2ratio (ME/E) | Up or down | P value | Q value |
---|---|---|---|---|---|---|---|---|
Hsa-miR-146a-3p | 112 | 0 | 3.350666667 | 0.001 | −7.67164541 | Down | 7.68E-25 | 1.09E-24 |
Hsa-miR-483-5p | 374 | 3 | 6.520666667 | 0.084 | −6.826222447 | Down | 1.27E-83 | 3.22E-83 |
Hsa-miR-378e | 6,511 | 75 | 147.6166667 | 1.833333333 | −6.304135221 | Down | 0 | 0 |
Hsa-miR-204-5p | 26,456 | 580 | 777.6066667 | 14.46 | −5.375688728 | Down | 0 | 0 |
Hsa-miR-3656 | 552 | 20 | 9.79 | 0.46 | −4.65088685 | Down | 6.95E-121 | 2.08E-120 |
Hsa-miR-320d | 130,535 | 18,609 | 2,570.156667 | 376.01 | −2.674654737 | Down | 0 | 0 |
Hsa-miR-320a | 43,493 | 8,065 | 823.9766667 | 177.35 | −2.295327274 | Down | 0 | 0 |
Hsa-miR-423-5p | 6,146 | 1,359 | 165.99 | 35.59333333 | −2.041392799 | Down | 0 | 0 |
Hsa-miR-204-3p | 871 | 196 | 18.47 | 4.923333333 | −2.016109552 | Down | 7.77E-90 | 2.03E-89 |
Only miRNAs with a ratio of less than 2 were listed. miRNAs, microRNA.
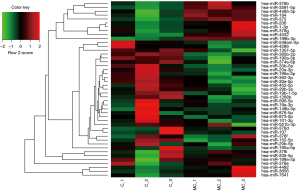
Validation of up-regulated miRNAs in MLN8237-treated senescent cells
To verify the RNA-Seq data, four up-regulated miRNAs like, miR-378b, miR-206, miR-375, and miR-199b were selected to test their expression levels by qRT-PCR. (Figure 5). In senescent cells, the expression levels of four miRNA were significantly up-regulated. In particular, miR-378b level was more than 100 times higher than that of the untreated cells. The results of qPCR were consistent with that of RNA-Seq, implying that the deep sequencing results were reliable and appropriate for further analysis.
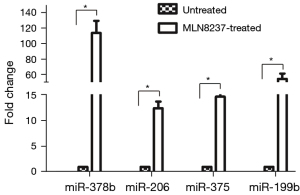
Target prediction, pathway enrichment and network analysis
Using several software, we predicted the possible targets of differentially expressed miRNAs in senescent IMR32 cells. And GO enrichment analysis was performed for screened targets. Targets usually interact with each other to play roles in certain biological functions. We performed pathway enrichment analysis of targets based on KEGG database. We also generated a scatter plot for the top 20 of KEGG enrichment results as Figure 6. Proteins controlled by senescent-related miRNAs are mainly concentrated in metabolic pathways.
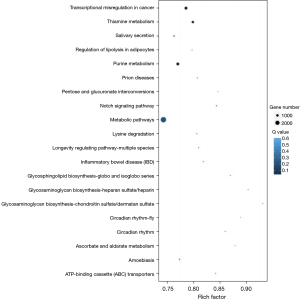
Discussion
Low concentration chemotherapeutic drugs provoked tumor cell senescence is the mechanism of chemotherapy control of tumor. In previous studies we observed the effects of chemotherapy drugs of doxorubicin, cisplatin, and MLN8237 on neuroblastoma cell lines of IMR32, SK-N-SH and SK-N-BE. Among the three drugs, AURKA inhibitor MLN8237 had the most obvious effect, about 87.4% IMR32 cells were arrested at G2/M phase. Nearly every cell under the microscope was blue stained, and the cells were huge and flat, showing classic senescent state (12). MLN8237 is a fascinating inhibitor of AURKA. It provoked the highest proportion of cell senescence, but at the same time, it also activated the intracellular Akt/Stat3 pathway, making cells “aged but not dead” (13). Due to miRNAs being highly sensitive to environmental stress, they are regarded as hormones to regulate cell-to-cell communication. Hence, we were interested in the change of miRNA expression profile in MLN8237-treated senescent IMR32 cells and their secreted vesicles. There are 13 miRNAs markedly increased and 33 miRNAs declined in senescence cells. In SAEVs, there were 48 up-regulated miRNAs and 9 down-regulated miRNAs, compared with normal IMR32 cells secreted extracellular vesicles. GO and KEGG analysis revealed that the differentially expressed miRNA target genes were concentrated in cellular metabolic pathways.
Among the up-regulated miRNAs in senescent IMR32 cells, miR-378b is the highest rank of significant differentially expressed miRNAs. Furthermore, the absolute abundance of miR-378b was the second highest level in senescent cells (Table S1). Another member of miR-378 family, miR-378g, whose absolute abundance ranked fourth in senescent cells. In addition, miR-378d and miR-378f are significantly decreased in senescent IMR32 cells. MiR-378/378* is an intronic miRNA, located within the peroxisome proliferator-activated receptor γ coactivator-1β (PGC-1β) genomic sequence. PGC-1β is a transcriptional coactivator that regulates metabolism and mitochondrial biogenesis through stimulation of nuclear hormone receptors and other transcription factors. MiR-378/378* is co-expressed with its host gene and seems to counterbalance the metabolic actions of PGC-1β (14). Recent research found that cellular senescence is also accompanied by a deep reshaping of miRNA expression and by the modulation of mitochondria activity, both master regulators of the SASP. These miRNAs are known as senescence-related miRNA (SA-miRNAs). SA-miRNAs can translocate to mitochondria (SA-mitomiRs) and may affect the energetic, oxidative, and inflammatory status of senescent cells (15). As one of SA-miRNAs, mitomiR-378 targets mt-ATP6 with a concomitant reduction in the functionality of the ATP synthase. MiR-378 antagomir resulted in an increase of ATP synthase activity, which was significantly decreased in diabetic (16). Mice genetically lacking miR-378 exhibit enhanced mitochondrial fatty acid metabolism and elevated oxidative capacity of insulin-target tissues (14), proving its key role in mitochondrial respiration. In addition to metabolism regulation and mitochondrial biogenesis, miR-378 also plays important roles in regulating lipid metabolism through controlling the expression of a set of lipoic genes (17) and being involved in the regulation of the cell differentiation process (18,19). In a word, miR-378/378* may act as a mediator for MLN-induced cell senescence and play a role in the metabolism of senescent cells. Although we did not verify the relationship between miR-378/378* and PGC-1β, the up-regulation of miR-378b, miR-378g and down-regulation of miR-378d, miR-378f in senescent cells are sufficient to demonstrate the complex relationship between miR-378/378* and PGC-1β, which is worthy of further careful study.
Our previous results showed SAEVs harbored distinct RNA signatures and modify bystander macrophage reactions (11). Herein, we analyzed the differentially expressed known miRNAs and found miR-205 topped the list of up-regulated miRNAs in SAEVs (Table 6). Interestingly, miR-205 also topped the list of down-regulated miRNAs in senescent cells (Table 5). It seems that cells packaged miR-205 out in response to senescence pressures. In this case, what is the role of miR-205 playing or what do senescent cells want miR-205 to do? This is a fascinating question. In literature, the majority of studies have indicated that miRNA-205 is a tumor suppressor (20-27). Nevertheless, numerous studies have presented contradictory results, demonstrating that miRNA-205 may function as an oncogene (28-33). A meta-analysis of 17 studies indicates a prognostic value of miR-205 in multiple human malignant neoplasms (34). Their results imply that miRNA-205 is a promising biomarker for predicting the recurrence and progression of patients with adenocarcinomas or breast cancer. Could miR-205 in SAEVs carrying “seed message” conserved by tumor cells for survival and escape through vesicles for future recurrence? Nevertheless, the role of miR-205 in the communication between senescent cells and the environment is also worthy of further study.
Another miRNA worth mentioning is miR-26a, which has the highest abundance in SAEVs (Table S2). MiR-26a subtype belongs to a functional family of miR-26. The expression of miR-26a varied in different kinds of tumors and exhibited alteration during developmental and normal tissue growth (35). Like miR-205, miR-26a likewise shows a dual role in a different kind of cancer diseases, being a tumor suppressor in some (36,37) and a tumor promoter in others (38). Previous studies have also indicated that miR-26a played a marked role in governing the metabolism of glucose, lipids, and insulin sensitivity (39) and pointed to its regulatory effect on oxidative stress caused by hydrogen peroxide produced in vascular smooth muscle cells (40). What is the intention of senescent cells to deliver miRNAs with complex roles like as miR-205 and miR-26a to the distal locations? which functions the miRNAs in EVs exerts depends on the specific tumor context and target genes. Further studies are warranted to throw light on better understanding of tumor cell interactions with surroundings under drug stress.
In summary, we used AURKA inhibitor MLN8237 to treat neuroblastoma cell line IMR32 to establish a cell senescence model. Through small RNA deep sequencing, we observed the miRNA profiles in senescent cells and SAEVs. The top 1 miRNA of differentially expressed in senescence cells and SAEVs is miR-378b and miR-205-5p, respectively. The two miRNAs play important roles in cellular metabolism regulation according to the pathway enrichment analysis of DESs target. Interestingly, miR-205-5p is also top 1 of down-regulated miRNAs in senescence cells. It seems that cells package miR-205-5p out into EVs in response to senescence pressures. Studying the roles of these miRNAs in cellular senescence will contribute to understanding the remodeling of the microenvironment by TIS and provide ideas for clinical tumor treatment.
Acknowledgments
Funding: The study was funded by General Project of Natural Science Foundation of Hubei Province (No. 2019CFB440), and Health and Family Planning Scientific Research Project of Hubei Province (No. WJ2019M135).
Footnote
Reporting Checklist: The authors have completed the MDAR reporting checklist. Available at https://tcr.amegroups.com/article/view/10.21037/tcr-21-2438/rc
Data Sharing Statement: Available at https://tcr.amegroups.com/article/view/10.21037/tcr-21-2438/dss
Conflicts of Interest: All authors have completed the ICMJE uniform disclosure form (available at https://tcr.amegroups.com/article/view/10.21037/tcr-21-2438/coif). XZ received funding from Health and Family Planning Scientific Research Project of Hubei Province (No. WJ2019M135). YY received funding from General Project of Natural Science Foundation of Hubei Province (No. 2019CFB440). The other authors have no conflicts of interest to declare.
Ethical Statement: The authors are accountable for all aspects of the work in ensuring that questions related to the accuracy or integrity of any part of the work are appropriately investigated and resolved. The study was conducted in accordance with the Declaration of Helsinki (as revised in 2013).
Open Access Statement: This is an Open Access article distributed in accordance with the Creative Commons Attribution-NonCommercial-NoDerivs 4.0 International License (CC BY-NC-ND 4.0), which permits the non-commercial replication and distribution of the article with the strict proviso that no changes or edits are made and the original work is properly cited (including links to both the formal publication through the relevant DOI and the license). See: https://creativecommons.org/licenses/by-nc-nd/4.0/.
References
- Ewald JA, Desotelle JA, Wilding G, et al. Therapy-induced senescence in cancer. J Natl Cancer Inst 2010;102:1536-46. [Crossref] [PubMed]
- Milanovic M, Fan DNY, Belenki D, et al. Senescence-associated reprogramming promotes cancer stemness. Nature 2018;553:96-100. [Crossref] [PubMed]
- Schosserer M, Grillari J, Breitenbach M. The Dual Role of Cellular Senescence in Developing Tumors and Their Response to Cancer Therapy. Front Oncol 2017;7:278. [Crossref] [PubMed]
- Lehmann BD, Paine MS, Brooks AM, et al. Senescence-associated exosome release from human prostate cancer cells. Cancer Res 2008;68:7864-71. [Crossref] [PubMed]
- Kadota T, Fujita Y, Yoshioka Y, et al. Emerging role of extracellular vesicles as a senescence-associated secretory phenotype: Insights into the pathophysiology of lung diseases. Mol Aspects Med 2018;60:92-103. [Crossref] [PubMed]
- Turchinovich A, Drapkina O, Tonevitsky A. Transcriptome of Extracellular Vesicles: State-of-the-Art. Front Immunol 2019;10:202. [Crossref] [PubMed]
- Ambros V. The functions of animal microRNAs. Nature 2004;431:350-5. [Crossref] [PubMed]
- Bartel DP. MicroRNAs: genomics, biogenesis, mechanism, and function. Cell 2004;116:281-97. [Crossref] [PubMed]
- Bayraktar R, Van Roosbroeck K, Calin GA. Cell-to-cell communication: microRNAs as hormones. Mol Oncol 2017;11:1673-86. [Crossref] [PubMed]
- Leung AK, Sharp PA. MicroRNA functions in stress responses. Mol Cell 2010;40:205-15. [Crossref] [PubMed]
- Liewer S, Huddleston A. Alisertib: a review of pharmacokinetics, efficacy and toxicity in patients with hematologic malignancies and solid tumors. Expert Opin Investig Drugs 2018;27:105-12. [Crossref] [PubMed]
- Zhou Q, Ding LL, Li F, et al. Study of isolation and characterization of extracellular vesicles in senescent tumor cells induced by chemotherapeutics. Chinese Journal of Immunology 2020;36:1800-4.
- Yang Y, Ding L, Zhou Q, et al. Silencing of AURKA augments the antitumor efficacy of the AURKA inhibitor MLN8237 on neuroblastoma cells. Cancer Cell Int 2020;20:9. [Crossref] [PubMed]
- Carrer M, Liu N, Grueter CE, et al. Control of mitochondrial metabolism and systemic energy homeostasis by microRNAs 378 and 378*. Proc Natl Acad Sci U S A 2012;109:15330-5. [Crossref] [PubMed]
- Giuliani A, Prattichizzo F, Micolucci L, et al. Mitochondrial (Dys) Function in Inflammaging: Do MitomiRs Influence the Energetic, Oxidative, and Inflammatory Status of Senescent Cells? Mediators Inflamm 2017;2017:2309034. Erratum in: Mediators Inflamm 2019;2019:8716351. [Crossref] [PubMed]
- Jagannathan R, Thapa D, Nichols CE, et al. Translational Regulation of the Mitochondrial Genome Following Redistribution of Mitochondrial MicroRNA in the Diabetic Heart. Circ Cardiovasc Genet 2015;8:785-802. [Crossref] [PubMed]
- Gerin I, Bommer GT, McCoin CS, et al. Roles for miRNA-378/378* in adipocyte gene expression and lipogenesis. Am J Physiol Endocrinol Metab 2010;299:E198-206. [Crossref] [PubMed]
- Hupkes M, Sotoca AM, Hendriks JM, et al. MicroRNA miR-378 promotes BMP2-induced osteogenic differentiation of mesenchymal progenitor cells. BMC Mol Biol 2014;15:1. [Crossref] [PubMed]
- You L, Gu W, Chen L, et al. MiR-378 overexpression attenuates high glucose-suppressed osteogenic differentiation through targeting CASP3 and activating PI3K/Akt signaling pathway. Int J Clin Exp Pathol 2014;7:7249-61. [PubMed]
- Hulf T, Sibbritt T, Wiklund ED, et al. Epigenetic-induced repression of microRNA-205 is associated with MED1 activation and a poorer prognosis in localized prostate cancer. Oncogene 2013;32:2891-9. [Crossref] [PubMed]
- Markou A, Yousef GM, Stathopoulos E, et al. Prognostic significance of metastasis-related microRNAs in early breast cancer patients with a long follow-up. Clin Chem 2014;60:197-205. [Crossref] [PubMed]
- Hou SX, Ding BJ, Li HZ, et al. Identification of microRNA-205 as a potential prognostic indicator for human glioma. J Clin Neurosci 2013;20:933-7. [Crossref] [PubMed]
- Hagman Z, Haflidadottir BS, Ceder JA, et al. miR-205 negatively regulates the androgen receptor and is associated with adverse outcome of prostate cancer patients. Br J Cancer 2013;108:1668-76. [Crossref] [PubMed]
- Quesne JL, Jones J, Warren J, et al. Biological and prognostic associations of miR-205 and let-7b in breast cancer revealed by in situ hybridization analysis of micro-RNA expression in arrays of archival tumour tissue. J Pathol 2012;227:306-14. [Crossref] [PubMed]
- Hanna JA, Hahn L, Agarwal S, et al. In situ measurement of miR-205 in malignant melanoma tissue supports its role as a tumor suppressor microRNA. Lab Invest 2012;92:1390-7. [Crossref] [PubMed]
- Schaefer A, Jung M, Mollenkopf HJ, et al. Diagnostic and prognostic implications of microRNA profiling in prostate carcinoma. Int J Cancer 2010;126:1166-76. [PubMed]
- Childs G, Fazzari M, Kung G, et al. Low-level expression of microRNAs let-7d and miR-205 are prognostic markers of head and neck squamous cell carcinoma. Am J Pathol 2009;174:736-45. [Crossref] [PubMed]
- Kalogirou C, Spahn M, Krebs M, et al. MiR-205 is progressively down-regulated in lymph node metastasis but fails as a prognostic biomarker in high-risk prostate cancer. Int J Mol Sci 2013;14:21414-34. [Crossref] [PubMed]
- Zhang YK, Zhu WY, He JY, et al. miRNAs expression profiling to distinguish lung squamous-cell carcinoma from adenocarcinoma subtypes. J Cancer Res Clin Oncol 2012;138:1641-50. [Crossref] [PubMed]
- Tran MN, Choi W, Wszolek MF, et al. The p63 protein isoform ΔNp63α inhibits epithelial-mesenchymal transition in human bladder cancer cells: role of MIR-205. J Biol Chem 2013;288:3275-88. [Crossref] [PubMed]
- Karaayvaz M, Zhang C, Liang S, et al. Prognostic significance of miR-205 in endometrial cancer. PLoS One 2012;7:e35158. [Crossref] [PubMed]
- Radojicic J, Zaravinos A, Vrekoussis T, et al. MicroRNA expression analysis in triple-negative (ER, PR and Her2/neu) breast cancer. Cell Cycle 2011;10:507-17. [Crossref] [PubMed]
- Markou A, Tsaroucha EG, Kaklamanis L, et al. Prognostic value of mature microRNA-21 and microRNA-205 overexpression in non-small cell lung cancer by quantitative real-time RT-PCR. Clin Chem 2008;54:1696-704. [Crossref] [PubMed]
- Zhang JY, Sun MY, Song NH, et al. Prognostic role of microRNA-205 in multiple human malignant neoplasms: a meta-analysis of 17 studies. BMJ Open 2015;5:e006244. [Crossref] [PubMed]
- Calin GA, Croce CM. MicroRNA signatures in human cancers. Nat Rev Cancer 2006;6:857-66. [Crossref] [PubMed]
- Fu X, Meng Z, Liang W, et al. miR-26a enhances miRNA biogenesis by targeting Lin28B and Zcchc11 to suppress tumor growth and metastasis. Oncogene 2014;33:4296-306. [Crossref] [PubMed]
- Yang X, Zhang XF, Lu X, et al. MicroRNA-26a suppresses angiogenesis in human hepatocellular carcinoma by targeting hepatocyte growth factor-cMet pathway. Hepatology 2014;59:1874-85. [Crossref] [PubMed]
- Zhang J, Han C, Wu T. MicroRNA-26a promotes cholangiocarcinoma growth by activating β-catenin. Gastroenterology 2012;143:246-56.e8. [Crossref] [PubMed]
- Fu X, Dong B, Tian Y, et al. MicroRNA-26a regulates insulin sensitivity and metabolism of glucose and lipids. J Clin Invest 2015;125:2497-509. [Crossref] [PubMed]
- Peng J, He X, Zhang L, et al. MicroRNA-26a protects vascular smooth muscle cells against H2O2-induced injury through activation of the PTEN/AKT/mTOR pathway. Int J Mol Med 2018;42:1367-78. [Crossref] [PubMed]