Ryanodine receptor (RYR) mutational status correlates with tumor mutational burden, age and smoking status and stratifies non-small cell lung cancer patient prognosis
Introduction
Calcium signaling abnormality is one key alteration in many cancers (1). It is also one of the main aberrancies in non-small cell lung cancer (NSCLC) (2). Calcium signaling is composed of many key proteins involving calcium pumps, such as sarcoplasmic retinal calcium ATPase (SERCA), and calcium channels, such as ryanodine receptors (RYRs) (3). Both of them are key transmembrane proteins regulating calcium release from intracellular calcium stores. There are many calcium channels involved in calcium signaling, mainly including voltage-gated calcium channels and ligand-gated calcium channels. Ryanodine receptor (RYR) is a calcium release channel located in endoplasmic reticulum or sarcoplasmic reticulum (ER/SR). It can rapidly release Ca2+ from ER/SR to perform a range of very important physiological functions, including excitation-contraction coupling, muscle contraction, cell growth, differentiation, metabolism, exocytosis, and apoptosis (4). RYRs also play crucial roles in maintaining intracellular calcium balance. There are three subtypes of RYRs, including RYR1 (mainly in skeletal muscle), RYR2 (mainly in heart muscle) and RYR3 (more widely distributed, mainly in the brain). The activity of RYR is regulated by many small molecules, such as calcium, magnesium and caffeine, and some large molecules, such as calmodulin (4). RYRs have been shown to play key roles in muscular, cardiac and neurological diseases (4).
The monomer of RYR1, RYR2 and RYR3 has 5,032–5,037, 4,968–4,976 and 4,872 amino acids, respectively (4-9). As a large protein, alterations of RYR key amino acids are known to play key roles in a series of rare diseases, including malignant hyperthermia (MH), central core disease (CCD), catecholaminergic polymorphic ventricular tachycardia (CPVT) and arrhythmogenic right ventricular dysplasia type 2 (ARVD2) (4-9). Point mutations of RYRs may cause these severe phenotypes, suggesting the importance of key amino acids in maintaining normal RYR channel function. It is widely known that large number of mutations can be found in NSCLC, however, the profile of mutations in calcium channels has rarely been investigated. Since RYRs are large proteins with important roles in maintaining intracellular calcium balance, it would be interesting to study their roles in cancer. Although it has long been known that calcium signaling is altered in NSCLC (3), the exact role of RYRs in NSCLC transformation and development has rarely been studied, and the mutational landscape of the three RYR isoforms and the roles of their mutations in NSCLC have not been systematically studied. Here we performed the first comprehensive study on RYR mutational landscape and its correlation with NSCLC phenotypes using the data from TCGA database. We identified characteristic RYR mutational profile in NSCLC and established its correlation with patient phenotypes. Our study provided the first observation on RYR genetic alterations and their potential influences in NSCLC. We present the following article in accordance with the REMARK reporting checklist (available at https://tcr.amegroups.com/article/view/10.21037/tcr-21-2395/rc).
Methods
The whole-exome somatic mutation data along with demographic and clinical information of 1,052 NSCLC patients was downloaded from The Cancer Genome Atlas (TCGA) database (https://portal.gdc.cancer.gov/). Patient demographic, clinical and mutational information is summarized in Table 1. Data files in mutation annotation format (MAF) format were obtained using the “TCGAbiolinks” package of R software (https://www.rstudio.com/). Mutation profile and tumor mutational burden (TMB) were analyzed using the “maftools” of R software, and the distribution of RYR1, RYR2, RYR3 mutations was displayed by lollipop plot also using the “maftools” of R software. The study was conducted in accordance with the Declaration of Helsinki (as revised in 2013).
Table 1
Factors | Categories | Number of subjects (%) |
---|---|---|
Gender, n (%) | Female | 400 (38.0) |
Male | 600 (57.0) | |
Not specified | 52 (4.9) | |
Ethnicity, n (%) | Hispanic or Latino | 15 (1.4) |
Not Hispanic or Latino | 689 (65.5) | |
Not reported | 296 (28.1) | |
Not specified | 52 (4.9) | |
Age, years, mean (SD) | – | 66.27 (9.4) |
Pathological types, n (%) | LUAD | 561 (53.3) |
LUSC | 491 (46.7) | |
Clinical stage, n (%) | Stage I | 514 (48.9) |
Stage II | 277 (26.3) | |
Stage III | 166 (15.8) | |
Stage IV | 32 (3.0) | |
Not specified | 63 (6.0) | |
T stage, n (%) | T1 | 279 (26.5) |
T2 | 565 (53.7) | |
T3 | 112 (10.6) | |
T4 | 42 (4.0) | |
TX | 2 (0.2) | |
Not specified | 52 (4.9) | |
N stage, n (%) | N0 | 636 (60.5) |
N1 | 227 (21.6) | |
N2 | 113 (10.7) | |
N3 | 7 (0.7) | |
NX | 16 (1.5) | |
Not specified | 53 (5.0) | |
M stage, n (%) | M0 | 748 (71.1) |
M1 | 32 (3.0) | |
MX | 212 (20.2) | |
Not specified | 60 (5.7) | |
Site of resection or biopsy, n (%) | Lower lobe, lung | 345 (32.8) |
Main bronchus | 9 (0.9) | |
Middle lobe, lung | 35 (3.3) | |
Overlapping lesion of lung | 11 (1.0) | |
Upper lobe, lung | 551 (52.4) | |
Not specified | 101 (9.6) | |
Residual tumor, n (%) | R0 | 729 (69.3) |
R1 | 25 (2.4) | |
R2 | 8 (0.8) | |
RX | 48 (4.6) | |
Not specified | 242 (23.0) | |
RYR1, n (%) | WT | 870 (82.7) |
Mut | 182 (17.3) | |
RYR2, n (%) | WT | 631 (60.0) |
Mut | 421 (40.0) | |
RYR3, n (%) | WT | 828 (78.7) |
Mut | 224 (21.3) | |
Total | – | 1,052 (100.0) |
NSCLC, non-small cell lung cancer; NS, not specified; SD, standard deviation; LUAD, lung adenocarcinoma; LUSC, lung squamous cell carcinoma; WT, wild type; Mut, mutant; RYR, ryanodine receptor.
Statistical analysis
All patients were divided into mutation group (Mut) and wide type group (WT) in mutational status analysis for RYR1, RYR2 and RYR3. Wilcoxon test was performed to compare the difference between the Mut and the WT groups in TMB, number-pack-years-smoked, tobacco-smoking-history grading and age-at-initial-pathologic-diagnosis. Linear regression was performed to analyze the correlation between cumulative number of mutations and cumulative TMB. Kaplan-Meier analysis and log-rank test were performed to investigate the potential stratification of RYR1, RYR2, RYR3 mutations on patient overall survival. Univariate and multivariate analyses were performed based on the stratification of prognosis by clinicopathological factors and mutational status. All analyses were performed and all figures were plotted using the corresponding packages of the R software.
Results
Mutation profiling of RYRs genes revealed characteristic genetic alterations in NSCLC
The mutation profile of RYRs in NSCLC was established first by analyzing the data of all RYR mutations. In 1,052 NSCLC patients with RYR mutation information available, 17.3% [182], 40.0% [421], and 21.3% [224] of patients were found to have at least one RYR1, RYR2 and RYR3 mutation, respectively (Table 1). It was obvious that the ratio of patients with RYR2 mutations far overweight that of RYR1 or RYR3 mutations. This is also reflected in the schemes of RYRs in Figure 1, in which the mutation rate of RYR2 (34.7%) also far overweight that of RYR1 (14.1%) and RYR3 (15.8%). It can also be observed from Figure 1 that the distribution of mutations in all three RYR isoforms was generally even across the full-length channels, with no obvious hotspot mutations.
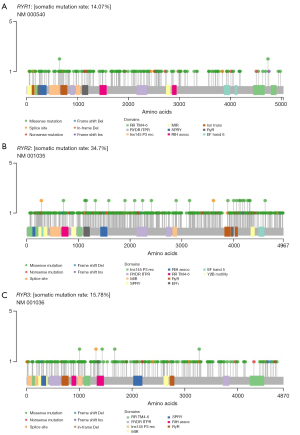
The mutational landscape of NSCLC with RYR mutations was plotted in Figure 2A to demonstrate the mutational status of patients with emphasis on RYR1, RYR2 and RYR3 related alterations. It can be observed that in a total of 502 patients with RYR mutations, RYR2 mutations accounted for 73% of patients, in contrast to 33% of patients with RYR3 mutations and 29% of patients with RYR1 mutations. Large number of significant co-mutations have been identified (Figure 2B). For example, RYR2 and RYR3, but not RYR1, co-mutated with ANK2 and APOB. RYR2, but not RYR1 and RYR3, co-mutated with PAPPA2 and FAM135B. Interestingly, some high-frequency mutations of NSCLC, such as those in TP53 (72%), TTN (69%) and SYNE1 (27%), were not significantly co-mutated with RYRs, possibly because mutations of these genes were comprehensively found in patients with or without RYR mutations (Figure 2B). More surprisingly, RYR2 mutations and RYR1 mutations were mutual exclusive, and RYR2 mutations and RYR3 mutations were mutual exclusive, while RYR1 mutations and RYR3 mutations were significantly co-mutated (Figure 2B). As previously reported, missense mutations were the predominant mutation type, with C>A and C>T base change as the main alterations. The median of variants per sample was 244.5 in this group of patients (Figure 2C). These observations suggest that RYR2 was the main RYR isoform that was mostly altered in NSCLC.
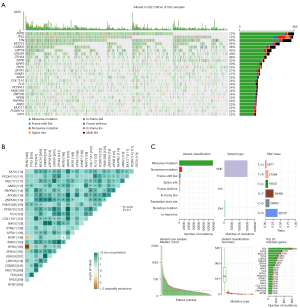
The affected functions and pathways in NSCLC with RYR mutations were further investigated by clustering enrichment analysis. Figure 3 shows the clustering enrichment results from Gene Ontology (GO), Kyoto Encyclopedia of Genes and Genomes (KEGG) and Reactome enrichments. It shows that many functions and pathways were affected, including ion channel activity, cell junction, extracellular matrix, phosphoinositide 3-kinase/protein kinase B (PI3K-AKT) signaling pathway, mitogen-activated protein kinase (MAPK) pathway, human papillomavirus (HPV) infection, herpes simplex virus 1 (HSV1) infection, neuroactive ligand-receptor interaction and receptor tyrosine kinase (RTK) signaling. All these aberrancies were reported in previous observations on lung cancer (1-3), suggesting that NSCLC patients with RYR mutations did not differ in main aberrancies from the whole NSCLC population.
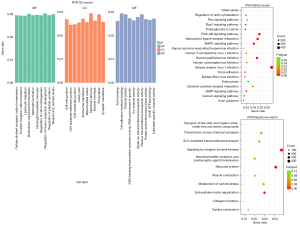
We further examined the mutational profile by investigating the correlation between RYR mutational status and TMB. Figure 4A shows significant linear correlation between the cumulative number of mutations (x-axis) and the cumulative TMB (y-axis) for RYR1, RYR2 and RYR3 from all involved patients. Moreover, patients with RYR mutations exhibited significantly higher TMB than those without RYR mutations for all three RYR isoforms (P<0.001, Figure 4B). These observations suggest that the local mutational status of RYRs correlated significantly with the mutational status of the whole exome.

RYR gene mutational status correlated with cancer risk factors and patient prognosis
The correlation between RYR mutational status and a series of clinicopathological factors was investigated. It can be seen from Table S1 that RYR2 mutational status was significantly correlated with the site of resection or biopsy and the residual tumor status, while no significant correlation was observed between RYR1/RYR3 and the examined factors. The correlation between mutational status of RYRs and age or smoking status was also investigated. Figure 5 shows that significant lower age was found in NSCLC patients with RYR2 (P<0.01) or RYR3 (P<0.05) mutations compared with those without RYR mutations, while this difference was not present with RYR1 [not significant (NS)]. Interestingly, patients with RYR1 (P<0.05), RYR2 (P<0.01) or RYR3 (P<0.001) mutations exhibited significantly higher smoking history grading than those without RYR mutations. Patient with RYR3 mutations showed significantly higher number of pack years than those without RYR3 mutations (P<0.01), while this difference was not present with RYR1 and RYR2 (NS). We further examined the potential stratification of prognosis by RYR mutations. It is clear from Figure 6 that patient with RYR2 (Figure 6B, P=0.038) mutations exhibited significantly better overall survival than those without RYR2 mutations. In contrast, RYR1 and RYR3 mutations did not exhibit significant stratification of patient survival (P=0.68 for RYR1 in Figure 6A, and P=0.19 for RYR3 in Figure 6C).
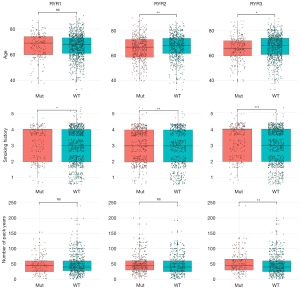
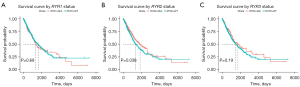
Univariate and multivariate analyses were performed with clinicopathological factors and RYR mutational status. It can be seen from Table 2 that in univariate analysis, clinical stage, T, N, M stage, residual tumor status, tumor location and RYR2 mutational status were significant factors affecting the patient prognosis. In subsequent multivariate analysis, clinical stage (stage IV), T stage (T3), residual tumor status (R1 resection) and RYR2 mutational status were significant factors affecting the patient prognosis, when compared with the corresponding reference group, suggesting these factors were independent risk factors for prognosis. It is notable that RYR2 mutational status was among the independent risk factors, suggesting RYR2 mutations alone can independently predict the patient prognosis.
Table 2
Variables | Univariate | Multivariate | |||
---|---|---|---|---|---|
HR (95% CI) | P value | HR (95% CI) | P value | ||
Gender | |||||
Female | Reference | – | – | – | |
Male | 1.17 (0.95–1.43) | 0.1461 | – | – | |
Ethnicity | |||||
Hispanic or Latino | Reference | – | – | – | |
Not Hispanic or Latino | 0.56 (0.29–1.09) | 0.0892 | – | – | |
Not reported | 0.56 (0.28–1.10) | 0.0916 | – | – | |
AJCC T | |||||
T1 | Reference | – | Reference | – | |
T2 | 1.35 (1.05–1.73) | 0.0188 | 1.23 (0.95–1.59) | 0.1524 | |
T3 | 2.26 (1.61–3.17) | 0.0000 | 2.06 (1.45–2.92) | 0.0001 | |
T4 | 2.88 (1.84–4.49) | 0.0000 | 1.88 (1.14–3.11) | 0.0013 | |
TX | 2.57 (0.36–18.49) | 0.3488 | 0.90 (0.09–8.41) | 0.9315 | |
AJCC N | |||||
N0 | Reference | – | Reference | – | |
N1 | 1.53 (1.21–1.92) | 0.0003 | 1.46 (1.15–1.86) | 0.0019 | |
N2 | 2.04 (1.54–2.72) | 0.0000 | 1.88 (1.39–2.53) | 0.0001 | |
N3 | 1.49 (0.37–6.00) | 0.5753 | 1.21 (0.27–5.24) | 0.8000 | |
NX | 1.51 (0.67–3.39) | 0.3237 | 1.70 (0.69–4.14) | 0.2461 | |
AJCC M | |||||
M0 | Reference | – | Reference | – | |
M1 | 2.25 (1.43–3.55) | 0.0005 | 1.74 (1.03–2.93) | 0.0377 | |
MX | 1.09 (0.84–1.43) | 0.5190 | 1.14 (0.86–1.51) | 0.3777 | |
Residual tumor | |||||
R0 | Reference | – | Reference | – | |
R1 | 3.47 (2.09–5.78) | 0.0000 | 2.34 (1.33–4.12) | 0.0031 | |
R2 | 1.62 (0.52–5.05) | 0.4070 | 1.32 (0.39–4.47) | 0.6470 | |
RX | 1.34 (0.81–2.22) | 0.2550 | 1.15 (0.68–1.94) | 0.5937 | |
Not specified | 0.58 (0.72–1.27) | 0.7610 | 0.95 (0.71–1.26) | 0.7012 | |
Site of resection or biopsy | |||||
Lower lobe, lung | Reference | – | – | – | |
Lung, not specified | 1.19 (0.78–1.80) | 0.4166 | 0.96 (0.63–1.46) | 0.8398 | |
Main bronchus | 0.46 (0.11–1.86) | 0.2759 | 0.40 (0.10–1.62) | 0.1976 | |
Middle lobe, lung | 2.04 (1.19–3.50) | 0.0093 | 1.31 (0.73–2.38) | 0.3667 | |
Overlapping lesion of lung | 1.24 (0.51–3.02) | 0.6418 | 0.90 (0.36–2.26) | 0.8305 | |
Upper lobe, lung | 1.11 (0.89–1.37) | 0.3682 | 1.14 (0.91–1.43) | 0.2559 | |
RYR1 | |||||
WT | Reference | – | – | – | |
Mut | 1.05 (0.82–1.36) | 0.6830 | – | – | |
RYR2 | |||||
WT | Reference | – | Reference | – | |
Mut | 0.80 (0.66–0.99) | 0.0382 | 0.80 (0.64–1.00) | 0.0390 | |
RYR3 | |||||
WT | Reference | – | – | – | |
Mut | 0.85 (0.66–1.01) | 0.1920 | – | – |
AJCC, American Joint Committee on Cancer; CI, confidence interval; HR, hazard ratio; WT, wild type; Mut, mutant; RYR, ryanodine receptor.
Discussion
The mutational landscape of NSCLC has been investigated by many studies, and calcium signaling is one of the well-known aberrancies in NSCLC (3). Calcium signaling is involved in many physiological processes, including muscle contraction, neuronal transmitter release, neural plasticity, protein phosphorylation, cell growth and death, hormone secretion and gene regulation (5). Abnormal calcium signaling leads to a dysregulation of the above processes in many cancers including NSCLC (3). Several key proteins of calcium signaling are implicated in NSCLC, such as calcium ATPase, RYR, IP3 receptor, voltage-gated calcium channels, sodium-calcium exchanger and transient receptor potential (TRP) channels (3). These receptors and channels regulate the influx and outflux of calcium across the cell membrane, ER or SR, maintaining the intracellular and extracellular calcium balance. Mutations of these receptors and channels in cancers may alter the function of these proteins and cause calcium dysregulation.
In this study, we found even distribution of mutations without hotspot mutations in all RYR isoforms, suggesting that RYR mutations in NSCLC may be the result rather than the cause of mutation accumulation during carcinogenesis. Unlike known NSCLC driver genes (such as EGFR and TP53) that exhibit high-frequency hotspot mutations (6), RYRs did not show any hotspot mutations, indicating essentially random mutation distribution across the whole length of RYRs, possibly representing a mutational background in NSCLC population. Thus, RYR mutations may not be driver gene mutations in NSCLC. Similar to other high frequency genes in NSCLC, the main type of mutation in RYRs was missense mutations, while a small proportion of mutations were nonsense and frameshift mutations. We suppose that most missense mutations may not change the protein function substantially, but nonsense and frameshift mutations may cause partial alteration or complete loss of channel function. Therefore, calcium signaling through RYRs may be compromised in patients with missense mutations at key amino acids or in those with impaired channel function caused by nonsense or frameshift mutations.
Interestingly, we found that the mutational frequency of RYR2 was much higher than that of RYR1 and RYR3, which could be the result of preferential base alterations happened to RYR2. This could be due to the findings that RYR2 was more expressed than RYR1 and RYR3 in lung tissue (7,8). RYRs expressed in skeletal muscle, cardiac muscle and brain have been extensively studied (9). However, their expression in lung has been much less studied, and their roles in lung cancer are far less understood. Therefore, our study provided new evidence for the alterations and possible roles of RYR isoforms in lung cancer. We identified significant co-mutations and mutually exclusive mutations in this study. Similar to previous lung cancer studies, substantial number of co-mutations were identified in our study (10). Strikingly, RYR2 mutations were mutual exclusive to RYR1 and RYR3 mutations, while RYR1 mutations and RYR3 mutations were significantly co-mutated, suggesting that patients with RYR2 mutations were less likely to have RYR1 or RYR3 mutations, while patients tended to have both RYR1 and RYR3 mutations. This observation highlighted the distinct role of RYR2 in lung cancer compared with RYR1 and RYR3. It was possibly because RYR2 had much more mutations than RYR1 and RYR3, leading to lower coexistence ratio of RYR2/RYR1 and RYR2/RYR3 mutations compared with RYR1/RYR3 mutations.
TMB is a widely accepted biomarker for stratification of response to cancer immunotherapy, in which patients with high TMB appeared to have better response and survival rate than those with low TMB (11,12). Our analysis demonstrated the positive correlation between higher TMB and RYR mutations. Thus, patients with RYR mutations represented a population with higher TMB, indicating that the local mutational status of RYRs reflected the whole exome mutational status. Therefore, it can be expected that patients with RYR mutations exhibited better prognosis than those without RYR mutations. Although correlation was found in populations, it is difficult to correlate RYR mutations with TMB at individual patient level, because the number of RYR mutations for each patient was low and not enough to calculate the whole-exome TMB. The sequenced exome must be long enough to provide accurate TMB estimation. Therefore, next-generation sequencing panels with panel size higher than 1 megabyte (MB) were generally used for TMB calculation (13). However, patients with RYR mutations could suggest higher chance of benefit from immunotherapy.
Age is an independent risk factor for cancer (14-16). In healthy population, sporadic genomic mutations accumulate with the increase of age, and malignant transformation of tissues during carcinogenesis exacerbates the accumulation of mutations (17,18). In our study, patients with RYR2 and RYR3 mutations showed significantly lower age compared with those with wild type RYRs, which contradicted previous observations that elder patients correlated with higher TMB (19). The number of somatic mutations is affected by many factors other than age. Mechanism of carcinogenesis, driver gene mutations and copy number variations all influence the number of mutations for certain individual patient. Since RYR mutations were correlated with higher TMB, the reason for the correlation between lower age and RYR mutations is worth more investigation. In contrast, smoking status correlated with RYR mutations in all three isoforms, which was consistent with previous observations that smokers were inclined to have higher TMB in NSCLC (20-22). Therefore, it appeared that the influence of smoking was comprehensive, and RYR mutational status was likely a reflection of the whole genomic alterations in smoking population.
In this study, we showed for the first time that RYR2 mutational status can stratify the patient prognosis, in which patients with RYR2 mutations exhibited better survival than those without mutations. RYR2 mutational status was also an independent risk factor for NSCLC patient prognosis. Although patients involved in this study belonged to a mix population with various stages and therapeutic strategies, this result suggested that RYR2 mutational status was an indicator and predictor for better prognosis in NSCLC. Since RYR mutations were correlated with higher TMB, and higher TMB was shown to correlate with better survival in both resectable and unresectable NSCLC (11,12,23,24), our data suggested that the capability of RYR mutational status in prognosis stratification could be a reflection of the capability of TMB in prognosis stratification. On the other hand, the prognosis of NSCLC is influenced by many factors. Factors including age, sex, stage and metastasis are all well-known risk factors, while status of surgery, tyrosine kinase inhibitor (TKI) therapy and immunotherapy all affect the patient survival. Key driver gene mutations, such as EGFR, ALK, ROS1, TP53 and KRAS may also affect the patient survival if untreated, and affect the decision of treatment if target therapy is available (25). Therefore, although we found RYR2 mutational status as an independent risk factor for NSCLC patient prognosis, RYR2 alone is unlikely to accurately predict the patient survival. However, our study linked calcium signaling and related ion channels such as RYRs with NSCLC phenotypes, and provided a new perspective in lung cancer research.
This study had some limitations. First, although RYR mutational status appeared to correlate with TMB, age and smoking status and stratify the patient prognosis, the influence of individual mutations had not been determined. Since no hotspot mutations were found with RYR, the influence of mutations at main functional domains may be worth more studying. Secondly, no validation study has been performed to examine the effectiveness of prognosis prediction by RYR mutational status, and future prospective cohort study is needed to confirm this. Thirdly, the mutational status of RYR alone cannot fully ensure correction prediction of patient prognosis, and clinically-used indicators should be combined with RYR for prognosis interpretation. Fourthly, the number of patients for metastatic lung cancer (stage IV) was limited in this study, and future study should expand the number of this group for stage-dependent investigation.
Acknowledgments
Funding: This study was supported by the Joint Foundation of Science & Technology Department of Yunnan Province and Kunming Medical University [grant/award No. 2019FE001(-115)], the Foundation from health commission of Yunnan Province (grant/award No. 2018NS0271), and the Opening Project of the Clinical Medical Center of the First People’s Hospital of Yunnan Province (No. 2021LCZXXF-HX11).
Footnote
Reporting Checklist: The authors have completed the REMARK reporting checklist. Available at https://tcr.amegroups.com/article/view/10.21037/tcr-21-2395/rc
Data Sharing Statement: Available at https://tcr.amegroups.com/article/view/10.21037/tcr-21-2395/dss
Conflicts of Interest: All authors have completed the ICMJE uniform disclosure form (available at https://tcr.amegroups.com/article/view/10.21037/tcr-21-2395/coif). The authors have no conflicts of interest to declare.
Ethical statement:
Open Access Statement: This is an Open Access article distributed in accordance with the Creative Commons Attribution-NonCommercial-NoDerivs 4.0 International License (CC BY-NC-ND 4.0), which permits the non-commercial replication and distribution of the article with the strict proviso that no changes or edits are made and the original work is properly cited (including links to both the formal publication through the relevant DOI and the license). See: https://creativecommons.org/licenses/by-nc-nd/4.0/.
References
- Monteith GR, Prevarskaya N, Roberts-Thomson SJ. The calcium-cancer signalling nexus. Nat Rev Cancer 2017;17:367-80. [Crossref] [PubMed]
- Yang H, Zhang Q, He J, et al. Regulation of calcium signaling in lung cancer. J Thorac Dis 2010;2:52-6. [PubMed]
- Cui C, Merritt R, Fu L, et al. Targeting calcium signaling in cancer therapy. Acta Pharm Sin B 2017;7:3-17. [Crossref] [PubMed]
- Bers DM. Cardiac excitation-contraction coupling. Nature 2002;415:198-205. [Crossref] [PubMed]
- Berridge MJ. The Inositol Trisphosphate/Calcium Signaling Pathway in Health and Disease. Physiol Rev 2016;96:1261-96. [Crossref] [PubMed]
- Takeda M, Sakai K, Terashima M, et al. Clinical application of amplicon-based next-generation sequencing to therapeutic decision making in lung cancer. Ann Oncol 2015;26:2477-82. [Crossref] [PubMed]
- Sermersheim M, Kenney AD, Lin PH, et al. MG53 suppresses interferon-β and inflammation via regulation of ryanodine receptor-mediated intracellular calcium signaling. Nat Commun 2020;11:3624. [Crossref] [PubMed]
- Croisier H, Tan X, Chen J, et al. Ryanodine receptor sensitization results in abnormal calcium signaling in airway smooth muscle cells. Am J Respir Cell Mol Biol 2015;53:703-11. [Crossref] [PubMed]
- Meissner G. The structural basis of ryanodine receptor ion channel function. J Gen Physiol 2017;149:1065-89. [Crossref] [PubMed]
- He Y, Song L, Wang H, et al. Mutational Profile Evaluates Response and Survival to First-Line Chemotherapy in Lung Cancer. Adv Sci (Weinh) 2020;8:2003263. [Crossref] [PubMed]
- Duffy MJ, Crown J. Biomarkers for Predicting Response to Immunotherapy with Immune Checkpoint Inhibitors in Cancer Patients. Clin Chem 2019;65:1228-38. [Crossref] [PubMed]
- Strickler JH, Hanks BA, Khasraw M. Tumor Mutational Burden as a Predictor of Immunotherapy Response: Is More Always Better? Clin Cancer Res 2021;27:1236-41. [Crossref] [PubMed]
- Wang Z, Duan J, Cai S, et al. Assessment of Blood Tumor Mutational Burden as a Potential Biomarker for Immunotherapy in Patients With Non-Small Cell Lung Cancer With Use of a Next-Generation Sequencing Cancer Gene Panel. JAMA Oncol 2019;5:696-702. [Crossref] [PubMed]
- Toumazis I, Bastani M, Han SS, et al. Risk-Based lung cancer screening: A systematic review. Lung Cancer 2020;147:154-86. [Crossref] [PubMed]
- Kerr J, Anderson C, Lippman SM. Physical activity, sedentary behaviour, diet, and cancer: an update and emerging new evidence. Lancet Oncol 2017;18:e457-71. [Crossref] [PubMed]
- Fattovich G, Stroffolini T, Zagni I, et al. Hepatocellular carcinoma in cirrhosis: incidence and risk factors. Gastroenterology 2004;127:S35-50. [Crossref] [PubMed]
- Blokzijl F, de Ligt J, Jager M, et al. Tissue-specific mutation accumulation in human adult stem cells during life. Nature 2016;538:260-4. [Crossref] [PubMed]
- Yokoyama A, Kakiuchi N, Yoshizato T, et al. Age-related remodelling of oesophageal epithelia by mutated cancer drivers. Nature 2019;565:312-7. [Crossref] [PubMed]
- Chalmers ZR, Connelly CF, Fabrizio D, et al. Analysis of 100,000 human cancer genomes reveals the landscape of tumor mutational burden. Genome Med 2017;9:34. [Crossref] [PubMed]
- Sharpnack MF, Cho JH, Johnson TS, et al. Clinical and Molecular Correlates of Tumor Mutation Burden in Non-Small Cell Lung Cancer. Lung Cancer 2020;146:36-41. [Crossref] [PubMed]
- Jiang T, Shi J, Dong Z, et al. Genomic landscape and its correlations with tumor mutational burden, PD-L1 expression, and immune cells infiltration in Chinese lung squamous cell carcinoma. J Hematol Oncol 2019;12:75. [Crossref] [PubMed]
- Nagahashi M, Sato S, Yuza K, et al. Common driver mutations and smoking history affect tumor mutation burden in lung adenocarcinoma. J Surg Res 2018;230:181-5. [Crossref] [PubMed]
- Devarakonda S, Rotolo F, Tsao MS, et al. Tumor Mutation Burden as a Biomarker in Resected Non-Small-Cell Lung Cancer. J Clin Oncol 2018;36:2995-3006. [Crossref] [PubMed]
- Yang QF, Wu D, Wang J, et al. Development and validation of an individualized immune prognostic model in stage I-III lung squamous cell carcinoma. Sci Rep 2021;11:12727. [Crossref] [PubMed]
- Skoulidis F, Heymach JV. Co-occurring genomic alterations in non-small-cell lung cancer biology and therapy. Nat Rev Cancer 2019;19:495-509. [Crossref] [PubMed]