Association between eight hypermethylation-related genes and gastric cancer: a systematic review and meta-analysis
Introduction
Gastric cancer (GC) is the fifth most common malignant tumor, with an estimated one million new cases worldwide each year. Since diagnosis of GC usually happens at an advanced stage (1), it has a high mortality rate, with China, Japan, and South Korea accounting for highest incidence (2). The risk factors include environmental factors, such as smoking and helicobacter pylori infection, salt, and salted foods (3). In addition, the previous study has shown that genomic DNA alterations and mutations, that contribute to deregulation of metabolic pathways, as well as epigenetic machinery alterations could also lead to GC initiation and progression (4). Epigenetic processes include DNA methylation, chromatin remodeling, histone modification and functions associated with non-coding RNA (ncRNA) (5). Out of all the epigenetic processes, DNA methylation was one of the first identified and extensively studied epigenetic alterations (6). DNA promoter methylation is a chemical modification where a methyl group is transferred from cofactor S-adenosylmethionine to the C5 position of a cytosine residue in DNA to form 5-methylcytosine (7). Folic acid can provide S-adenosyl methionine (SAM), which can regulate the methylation of CpG island cytosine DNA. The phenotypic changes caused by DNA methylation are related to folic acid metabolism. Folic acid, as a coenzyme of one-carbon unit metabolism, directly participates in the transport of methyl groups in DNA methylation (8).
Hypermethylation of DNA sequences in the promoter region has been shown to modulate GC pathogenesis by silencing tumor suppressor genes (Figure 1). Cancer-related genes often control tumor-specific signaling pathways, cell cycle, DNA repair and apoptosis, but promoter hypermethylation can result intogenomic instability (9). Since epigenetic modulation plays a crucial role in cells, any alterations could serve as new clinical biomarkers and therapeutic targets for early detection, diagnosis and treatment of GC. This meta-analysis examined the correlation between the hypermethylation status of polygenes and gastric oncogenesis. We evaluated methylation in genes such as hMLH1 and MGMT; DNA repair genes, DAPK; an apoptosis-related gene, RASSF1A; a component of RAS pathway, CDH1; a cell migration/invasion gene, as well as RUNX3; a tumor suppressor gene (10). We present the following article in accordance with the PRISMA reporting checklist (available at https://tcr.amegroups.com/article/view/10.21037/tcr-22-372/rc).
Methods
Search strategies
We performed systematic search of literature in PubMed, Embase, Cochrane Library, CNKI, Wanfang and Cqvip electronic databases before May 2021, without language restriction. For a comprehensive search, we combined subject words and free words, such as “gastric cancer”, “GC”, “gastric carcinoma”, “methylation”, “DNA methylation”, “promoter methylation”, and then applied slight adjustments according to specifications in different databases. In addition, we screened reference lists of the included articles for any further relevant references. We exported all the searched articles to Endnote citation manager software. After merging the databases, we removed duplicate studies and excluded irrelevant studies following the inclusion and exclusion criteria by reading the titles and abstracts. We then read full texts of potential articles to identify eligible ones. We included studies with detailed information on the frequency of gene methylation for both the cancer and the regular control groups and methylation status on human tissue samples. Articles from animal experiments, cell lines, human xenografts, overlapping databases, and those with same population were excluded for the analysis. Letters, conference abstracts, expert opinions, reviews, editorials, letters and case reports were also excluded. Figure 2 shows the flow chart of the searches.
Data extraction
We extracted primary data from a customized data-extraction sheet. The primary data included year of publication, first author, ethnicity, geography location, type of tissues, methods of detection of DNA methylation, number of cases in tumor and non-tumor groups, number of methylation-positive individuals in each group and tumor clinicopathological features. Two authors independently performed the data extraction, and any disagreements were resolved first by consensus, then by consultation with a third investigator in case of disagreements.
Statistical analysis
We calculated pooled odds ratios (ORs) and 95% confidence intervals (CIs) for outcome analysis. Cochran’s χ2 test and I2 statistic were used to explore heterogeneity of the articles included in the meta-analysis. Presence of significant heterogeneity was quantified using the I2. The heterogeneity was acceptable when the I2 value was less than 50, with the results measured using a fixed-effects model. When the I2 value was 50% or greater, a random effect model was used to test for significant heterogeneity. In addition, we employed stratified analysis to further investigate the potential sources of heterogeneity and then implemented a sensitivity analysis to assess the stability of the meta-analysis. Moreover, we performed funnel plots to visually assess any publication bias for asymmetry, and also used Egger’s tests to quantify publication bias. The pooled ORs analyzed promoter DNA methylation between the GC and non-tumor gastric mucosa and assessed the relationship between DNA methylation and clinical characteristics. The meta-analysis was performed using Stata12.0 software (Stata Corp, College Station, TX, USA). All P values were two-sided, and a P value less than 0.05 was considered statistically significant.
Results
Study selection and study characteristics
This meta-analysis enrolled a total of 201 studies (140 papers) following the retrieval strategy and the inclusion/exclusion criteria (Figure 2). We exclude other genes from the meta-analysis due to their scarcity and lack of enough studies. We evaluated the methodological quality of the included studies according to NOQAS.
Among the included studies, 88% used methylation-specific PCR (MSP) while 5% employed quantitative-MSP (q-MSP) to detect DNA methylation. Other detection methods such as COBRA and Bisulfite hat were rarely used (available online: https://cdn.amegroups.cn/static/application/f73b47ab7360c5fb990f4b694bd229d9/tcr-22-372-1.xlsx). A total of 175 items (87%) were from Asian populations, 18 (9%) studies were from Caucasian populations, and only 4% were from African populations. In sync with previous studies, our analysis showed that the Asian countries had high incidence rate. We summarized the methylation frequency and clinical characteristics of 8 genes (available online: https://cdn.amegroups.cn/static/application/0f268a9be74fa5ede578f9d1a2b6f594/tcr-22-372-2.xlsx).
Relationship between p16 methylation status and GC
This study consisted of 38 studies, with 2,378 GC tissues and 1,991 non-cancer tissues, and investigated the association between the methylation status of the p16 promoter and tumor histology. Given the substantial heterogeneity between the studies (χ2=I2=87%; P<0.001), we applied a random-effects model for the analysis. Results showed that GC had a significantly higher frequency of p16 promoter methylation compared to the non-neoplastic samples (OR =9.68; 95% CI: 5.41–17.31; P<0.001; Figure 3), thus suggesting that p16 promoter hypermethylation might be contributing to the carcinogenesis of GC.
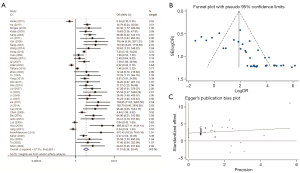
Relationship between CDH1 methylation status and GC
This analysis included 3,462 cases, with 30 studies evaluating the correlation between CDH1 methylation status and GC risk. MSP analysis showed that the overall pooled OR was 6.96 (95% CI: 4.07–10.98; Figure 4), with a sizeable between-study heterogeneity (χ2=I2=80.8%; P<0.001). The data demonstrated that hypermethylation of the CDH1 promoter increases the risk of GC.
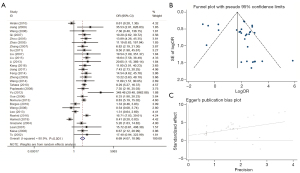
Relationship between hMLH1 methylation status and GC
We employed the random-effects model to estimate the pooled OR with the GC, and noncancerous groups showed substantial heterogeneity (χ2=I2=59.1%). The frequency of hMLH1 methylation was significantly higher in the GC group compared to the nonmalignant group (OR =9.19; 95% CI: 5.73–14.75; P<0.001; Figure 5). This finding suggested that hMLH1 promoter methylation plays a crucial role in the incidence of GC.
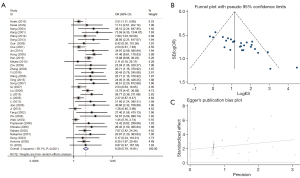
Relationship between RUNX3 methylation status and GC
We evaluated the relationship between RUNX3 methylation status and gastric carcinoma risk in 1,580 neoplastic cases and 1,438 controls from 27 selected papers. Using the random-effects model, our analysis showed that the RUNX3 promoter methylation increases the risk of GC with a pooled OR of 3.48 (95% CI: 2.50–4.86). Moreover, the included studies exhibited statistical heterogeneity (χ2=I2=73.7%; P<0.001) (Figure 6).
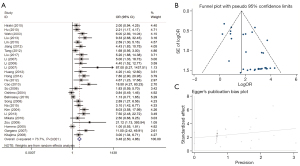
Relationship between RASSF1A methylation status and GC
To assess the relationship between RASSF1A promoter methylation status and GC risk, we selected 19 studies which had 1,286 gastric carcinoma cases and 801 controls. Based on the fixed-effect model, the results demonstrated that RASSF1A methylation was significantly related to elevated GC risk with a pooled OR of 6.41 (95% CI: 4.68–8.77) (Figure 7). Besides, the overall heterogeneity was low across the enrolled studies Figure 7.
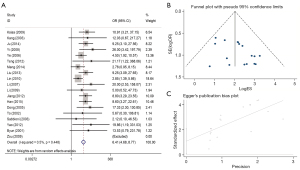
Relationship between MGMT methylation status and GC
We evaluated the methylation status of MGMT in GC using 1,789 GC specimens and 1,754 controls from 18 articles. Based on the random-effects model analysis, aberrant methylation of MGMT was a key risk factor for the development of GC with a pooled OR of 4.34 (95% CI: 2.77–6.80). In addition, there was remarkable heterogeneity across the included studies (χ2=I2=76.2%; P<0.001) (Figure 8).
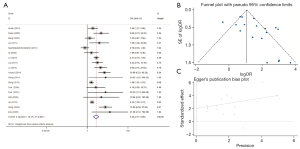
Relationship between DAPK methylation status and GC
The association between DAPK hypermethylation status and the risk for gastric carcinoma was assessed in 21 studies involving 1,233 GC samples and 1,374 controls. The included studies had significant heterogeneity (χ2=I2=65.3%; P<0.001). Results indicated that the GC group displayed markedly higher frequencies of DAPK promoter methylation than the control samples (OR =1.74; 95% CI: 1.27–2.39) (Figure 9). Thus, the aberrant methylation of DAPK might contribute to the initiation of gastric carcinogenesis.
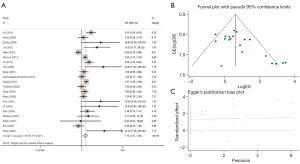
Relationship between CHFR methylation status and GC
A total of 13 studies with 1,352 cases, including 649 tumor tissues and 603 non-tumor tissues evaluated the role of methylation of CHFR gene in gastric carcinogenesis. The results revealed that the frequency of CHFR promoter methylation significantly correlated with GC tumorigenesis with a pooled OR of 4.46 (95% CI: 3.29–6.05) (Figure 10).
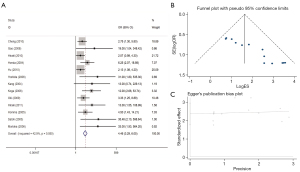
Correlation of gene promoter methylation with GC clinical features
We also examined DNA methylation in precancerous lesions (available online: https://cdn.amegroups.cn/static/application/0282356262afde11c2394cf10f278ace/tcr-22-372-3.xlsx). The risk of methylation of the p16, CDH1, hMLH1, RUNX3 and DAPK genes showed an increasing trend in the regular group—precancerous tissue—cancer tissue. p16, CDH1, hMLH1, RUNX3 and RASSF1A methylation had no statistical significance between precancerous lesions and normal tissues. On the other hand, we investigated the relationship between polygene methylation and clinical features in GC (available online: https://cdn.amegroups.cn/static/application/aac82091af91893855e0efc21dcb4d61/tcr-22-372-4.xlsx). The data showed that p16, CDH1, RUNX3, RASSF1A, MGMT, DAPK and CHFR methylation status were unrelated with GC patients’ gender or age. However, RASSF1A had a markedly higher frequency of promoter methylation in T3/4 GC compared to T1/2 GC. Moreover, gastric carcinoma patients with lymph node metastasis had a considerably higher p16, CDH1, RUNX3, MGMT methylation frequency compared to patients without the lymph node metastasis. The frequency of p16, RUNX3 methylation significantly increased in the GC patients with distant metastasis compared to those without distant metastasis. Furthermore, the methylation of p16, CDH1 and RUNX3 was higher in high-grade GC than low-grade GC. Besides, p16, hMLH1, CDH1, RUNX3, had a significantly higher methylation rate in GC patients in stage III/IV than stage I/II GC. In addition, our analysis demonstrated that the degree of methylation of CDH1 and RUNX3 genes was significantly higher in intestinal GC compared to that in diffuse GC. There was no significant correlation between the methylation status of the 8 gene promoters and GC vascular infiltration status or tumor size.
Stratified analysis and sensitivity analyses
We used meta-regression and subgroup analyses to explore the heterogeneity of the analyzed studies. The analysis showed that the methylation detection method, the specimen type and the ethnicity were not sources of heterogeneity in the overall meta-analysis for most of the included genes. In addition, studies from different countries (data not shown) could explain the significant heterogeneity in our meta-analysis (I2≥80; P<0.05).
The sensitivity analysis tested the impact of any study on the total estimate by omitting one study at a time. The analysis indicated that removing any of the included studies had no significant effect on the overall results, and this meta-analysis was robust and reliable (available online: https://cdn.amegroups.cn/static/application/aac82091af91893855e0efc21dcb4d61/tcr-22-372-4.xlsx). From the sensitivity analysis results, there were a few heterogeneous studies whose removal had no significant effect on the results.
Publication bias
We used Egger funnel plots with a 95% false confidence limits and Egger tests to estimate the publication bias of the included literature. The funnel plot was used to test the publication bias of the polygene methylation, and the symmetrical distribution of funnel plot indicated that there was no significant publication bias in this study (Figures 3B,4B,5B,6B,7B,8B,9B,10B and Figures 3C,4C,5C,6C,7C,8C,9C,10C).
Heterogeneity
The heterogeneity I2 of sensitivity analysis was 68.5%, and the Q test of sensitivity analysis showed P<0.001, indicating that the included studies were heterogeneous. After referring to the included articles, we summarized the possible sources of heterogeneity: (I) the changes of pathological stages in patients included in the study were from NA to IV. (II) Different groups are included. (III) The cut-off value of GPR set by different studies is quite different. We take heterogeneity into account when conducting the data synthesis.
Publication bias
Analysis of publication bias using STATA 16.1 (Deek’s funnel plot) did not reveal obvious publication bias (P=0.48) in the included study.
Discussion
The genes included in this meta-analysis participated in vital cell signaling pathways implicated in apoptosis, cell cycle, DNA repair as well as cell adhesion (9). The p16 gene is an inhibitor of CDK4 and CDK6 kinases, which control cell cycle G1 progression, regulates G1-S cell cycle transition by phosphorylation of Rb signaling modulates the activation of CDK4, CDK6, and cyclin D1 as well as the release of E2F to drive cell cycle progression (2,5). In addition, homozygous p16 deletion or silencing via epigenetic methylation is often observed in GC (11), and the inactivation of p16 has been implicated in gastric carcinogenesis (12). According to this study, there was significant p16 methylation in dysplasia, intestinal metaplasia, and GC compared to non-neoplastic tissue, implicating the methylation of p16 promoter as a risk factor of GC, which was consistent with a recent study (13). This study also demonstrated that the abnormal methylation state of p16 correlated with GC’s clinical and pathological characteristics. For instance, the frequency of abnormal methylation of the p16 gene promoter was significantly higher in GC patients with lymph node metastasis, distant metastasis, and TNM stage III/IV cancer tissues than in cancers without lymph node metastasis, no distant metastasis, and TNM stage I/II organization. Thus, the methylation status of the p16 promoter in GC tissue could help predict the potential of malignance and progression of GC.
CDH1 is essential in the maintenance of cell morphology and regulation of cell adhesion (14). CDH1 gene encodes E-cadherin (E-cad), which participates in the inhibition of migration and invasion of tumor cells (15). Interestingly, our study did not observe any relationship between the CDH1 methylation and GC Lauren classification, which merits further investigation. Epigenetic alterations have been shown to lead to CDH1 inactivation through an array of complex mechanisms. For instance, low E-cad expression in GC has been linked with methylation of CDH1 CpG islands, indicating that the CDH1 promoter methylation might be a critical molecular event in the initiation of GC (16). In addition, we showed that the methylation rate of CDH1 promoter in gastric carcinoma was higher than that in non-cancerous specimens, thus inactivation of the CDH1 by methylation could result into GC. The abnormal methylation status of the CDH1 gene was also correlated with GC’s clinical and pathological characteristics. The aberrant methylation frequency of CDH1 was considerably higher in T3/4, high grade, stage III/IV and intestinal GC than that in the T1/2, low grade, stage I/II as well as diffuse GC. The methylation status of the CDH1 gene in gastric carcinoma tissues could predict the progress and prognosis of gastric carcinomas.
hMLH1 and MGMT are mismatch repair (MMR) genes of DNA, which affect the endogenous repair function of cells, maintain genome stability, and inhibits GC (17-19). The MGMT gene could serve as a predictive biomarker in neuro-oncology and esophageal carcinoma (20,21). Besides, MGMT and hMLH1 correlated with GC clinicopathology. The methylation frequency of hMLH1 was higher in the age ≥60 GC group than in age <60 patients. In addition, the methylation frequency of MGMT was higher in lymphatic metastasis GC group compared those without lymphatic metastasis, suggesting that the methylation of hMLH1 or MGMT could lead to GC initiation and progression.
RUNX3 regulates the proliferation of GC cells by participating in the TGF and Wnt pathways (22-24). In addition, RUNX3 promotes the angiogenesis of GC by regulating the level of vascular endothelial growth factor (VEGF) (25). Previous experiments in animal models showed that RUNX3 dysfunction is related with the occurrence and progression of cancer (26,27). There was significant reduction in the expression of RUNX3 in GC, and the level of RUNX3 was positively correlated with the methylation of RUNX3 in the promoter region 6. In sync, our study showed that the methylation of RUNX3 affects GC and its clinicopathology. The methylation degree of the RUNX3 promoter was higher in patients with high grade, lymph node metastasis, distant metastasis, stage III/IV and intestinal GC, than in patients with low grade, stage I/II and diffuse GC. These results suggest that the degree of methylation of the RUNX3 promoter might help predict the progression of malignant GC.
RASSF1A suppresses tumor growth and is essential in apoptosis, cell cycle regulation as well as microtubule stability through the Ras signaling pathway (28,29). Methylation of RASSF1A promoter CpG island plays a significant role in the initiation and progression of GC (30). Likewise, our data showed that the abnormal methylation of the RASSF1A gene was related to GC and its clinical features. The methylation frequency of RASSF1A was higher in stage T3/4 GC than in stage T1/2 GC. The GC patients with lymph node metastasis were more likely to develop RASSF1A methylation compared to those without lymph node metastasis, suggesting that RASSF1A methylation could help predict the malignant potential and progression of GC. Overall, our results are consistent with and added more data to the prior study. (31).
The DAPK play a vital role in regulating cellular process and serve as positive mediators of apoptosis via extrinsic and intrinsic death-regulating signaling pathways (32). Moreover, DAPK has an essential function in mediating apoptotic signaling during tumorigenesis (33). The decreased expression of DAPK may lead to abnormal cell proliferation and even malignant tumors (34). Hypermethylation of DAPK is the leading cause of low expression in tumors (35). Coupled with previous meta-analysis, we suggest that methylation of DAPK was associated with gastric carcinogenesis (36). Our study demonstrated that the frequency of DAPK methylation in GC is not associated with clinical characteristics.
CHFR is a cell cycle regulator (30), a tumor suppressor gene (37), and controls cell division (38). We observed that methylation of the CHFR promoter in GC exceeded that in non-tumor tissues. The correlation between GC and its methylation status was consistent with the previous results in cell experiments (39).
In our meta-analysis, precancerous lesions showed increased frequency of methylation of MGMT, CDH1, and DAPK. Thus, gene methylation occurred at the initial stage of malignant transformation of the gastric mucosa and accumulated changes with the disease development. In addition, the levels of p16, CDH1, RUNX3, hMLH1, RASSF1A, MGMT and CHFR in GC were significantly higher than the hypermethylation levels in non-cancerous tissues, suggesting that the methylation of these genes might be a risk factor for GC. In addition, methylation of promoters of genes such as p16, CDH1, RUNX3, hMLH1, or RASSF1A were related with the clinicopathological characteristics of GC. Therefore, the level of methylation of these gene promoters in tissues can be used as prognostic indicators of GC.
Epigenetic alterations are potential biomarkers used to evaluate the risk of cancer, early identification of tumors, and predict patient’s prognosis and response to therapy (40). In addition to DNA promoter methylation of single genes or gene sets in tissues, other study shows they can be detected in liquid biopsy (biological fluids like urine, blood, saliva, and cerebrospinal fluid, among others) (41). However, no gene set is widely used for GC screening currently. Moreover, epigenetic therapeutics can be combined with traditional chemotherapies, targeted therapies, other epigenetic agents and immunotherapy modalities to broaden response rates among patients with hematologic cancers and could extend such treatments to solid tumors (42,43). Recently, various epi-drugs have been developed and tried in clinical use (44), and two classes of the epigenetic drugs, namely DNMT inhibitors (DNMTi) and HDAC inhibitors (HDACi), have shown positive results in experimental GC treatment. Nevertheless, these therapies have not yet reached clinical practice (5).
Our study was limited by the fact that we only included Chinese and English literature without retrieving other possible languages. Besides, the methylation of gene sets in plasma are potential serum biomarkers, which were not captured in our discussion. The serum biomarkers might support early detection of GC development and monitor disease progression dynamically. This article did not analyze Helicobacter pylori infection and almost all the included studies were case-control designs. In addition, most of the included literature was from the Asian population and estimates from the other areas were based on small numbers and should therefore be interpreted with caution.
Conclusions
Taken together, our data indicated that methylation of the polygene promoter perturbs gastric carcinogenesis and development. The study provides new insights into the occurrence of GC, suggesting that the methylation status of the polygene promoter could act as a new biomarker for GC diagnosis and prognosis. However, our conclusion needs further verification in large randomized controlled trials.
Acknowledgments
Funding: This study was supported by (I) Key Talents Project of Gansu Province (No. 2019RCXM020); (II) Key Project of Science and Technology in Gansu province (19ZD2WA001); (III) Science and technology project of Chengguan District of Lanzhou City (2019RCCX0034); (IV) Science and technology project of Chengguan District of Lanzhou City (2020SHFZ0039); (V) Science and technology project of Chengguan District of Lanzhou City (2020JSCX0073); (VI) Cuiying Scientific and Technological Innovation Program of Lanzhou University Second Hospital (No. CY2017-ZD01).
Footnote
Reporting Checklist: The authors have completed the PRISMA reporting checklist. Available at https://tcr.amegroups.com/article/view/10.21037/tcr-22-372/rc
Conflicts of Interest: All authors have completed the ICMJE uniform disclosure form (Available at https://tcr.amegroups.com/article/view/10.21037/tcr-22-372/coif). The authors have no conflicts of interest to declare.
Ethical Statement: The authors are accountable for all aspects of the work in ensuring that questions related to the accuracy or integrity of any part of the work are appropriately investigated and resolved.
Open Access Statement: This is an Open Access article distributed in accordance with the Creative Commons Attribution-NonCommercial-NoDerivs 4.0 International License (CC BY-NC-ND 4.0), which permits the non-commercial replication and distribution of the article with the strict proviso that no changes or edits are made and the original work is properly cited (including links to both the formal publication through the relevant DOI and the license). See: https://creativecommons.org/licenses/by-nc-nd/4.0/.
References
- Yoshida K, Yasufuku I, Terashima M, et al. International Retrospective Cohort Study of Conversion Therapy for Stage IV Gastric Cancer 1 (CONVO-GC-1). Ann Gastroenterol Surg 2021;6:227-40. [Crossref] [PubMed]
- Li Z, Liu Z, Li C, et al. CDCA1/2/3/5/7/8 as novel prognostic biomarkers and CDCA4/6 as potential targets for gastric cancer. Transl Cancer Res 2021;10:3404-17. [Crossref] [PubMed]
- van Amelsfoort RM, Walraven I, Kieffer J, et al. Quality of Life Is Associated With Survival in Patients With Gastric Cancer: Results From the Randomized CRITICS Trial. J Natl Compr Canc Netw 2022;20:261-7. [Crossref] [PubMed]
- Yamada H, Takeshima H, Fujiki R, et al. ARID1A loss-of-function induces CpG island methylator phenotype. Cancer Lett 2022;532:215587. [Crossref] [PubMed]
- Tie J, Zhang X, Fan D. Epigenetic roles in the malignant transformation of gastric mucosal cells. Cell Mol Life Sci 2016;73:4599-610. [Crossref] [PubMed]
- Bure IV, Nemtsova MV. Methylation and Noncoding RNAs in Gastric Cancer: Everything Is Connected. Int J Mol Sci 2021;22:5683. [Crossref] [PubMed]
- Cheng M, Zhan X, Xu Y, et al. DNA methylation of RNA-binding protein for multiple splicing 2 functions as diagnosis biomarker in gastric cancer pathogenesis and its potential clinical significance. Bioengineered 2022;13:4347-60. [Crossref] [PubMed]
- Mahmoud AM, Ali MM. Methyl Donor Micronutrients that Modify DNA Methylation and Cancer Outcome. Nutrients 2019;11:608. [Crossref] [PubMed]
- Pfeifer GP. Defining Driver DNA Methylation Changes in Human Cancer. Int J Mol Sci 2018;19:1166. [Crossref] [PubMed]
- Padmanabhan N, Ushijima T, Tan P. How to stomach an epigenetic insult: the gastric cancer epigenome. Nat Rev Gastroenterol Hepatol 2017;14:467-78. [Crossref] [PubMed]
- Kumari S, Kumar P, Kumar M, et al. Expression of p27 and p16 and their clinical significance in gastric cancer. Clin Transl Oncol 2021;23:856-65. [Crossref] [PubMed]
- Bae HJ, Kang SK, Kwon WS, et al. p16 methylation is a potential predictive marker for abemaciclib sensitivity in gastric cancer. Biochem Pharmacol 2021;183:114320. [Crossref] [PubMed]
- Kong R, Zhang EB, Yin DD, et al. Long noncoding RNA PVT1 indicates a poor prognosis of gastric cancer and promotes cell proliferation through epigenetically regulating p15 and p16. Mol Cancer 2015;14:82. [Crossref] [PubMed]
- De Re V, Alessandrini L, Brisotto G, et al. HER2-CDH1 Interaction via Wnt/B-Catenin Is Associated with Patients' Survival in HER2-Positive Metastatic Gastric Adenocarcinoma. Cancers (Basel) 2022;14:1266. [Crossref] [PubMed]
- Gamble LA, Rossi A, Fasaye GA, et al. Association Between Hereditary Lobular Breast Cancer Due to CDH1 Variants and Gastric Cancer Risk. JAMA Surg 2022;157:18-22. [Crossref] [PubMed]
- Liliac IM, Ungureanu BS, Mărgăritescu C, et al. E-Cadherin Modulation and Inter-Cellular Trafficking in Tubular Gastric Adenocarcinoma: A High-Resolution Microscopy Pilot Study. Biomedicines 2022;10:349. [Crossref] [PubMed]
- Tamura G. Alterations of tumor suppressor and tumor-related genes in the development and progression of gastric cancer. World J Gastroenterol 2006;12:192-8. [Crossref] [PubMed]
- Wani M, Afroze D, Makhdoomi M, et al. Promoter methylation status of DNA repair gene (hMLH1) in gastric carcinoma patients of the Kashmir valley. Asian Pac J Cancer Prev 2012;13:4177-81. [Crossref] [PubMed]
- Michailidi C, Theocharis S, Tsourouflis G, et al. Expression and promoter methylation status of hMLH1, MGMT, APC, and CDH1 genes in patients with colon adenocarcinoma. Exp Biol Med (Maywood) 2015;240:1599-605. [Crossref] [PubMed]
- Feng X, Xue F, He G, et al. Banxia xiexin decoction affects drug sensitivity in gastric cancer cells by regulating MGMT expression via IL-6/JAK/STAT3-mediated PD-L1 activity. Int J Mol Med 2021;48:165. [Crossref] [PubMed]
- Hegi ME, Ichimura K. MGMT testing always worth an emotion. Neuro Oncol 2021;23:1417-8. [Crossref] [PubMed]
- Guo X, Dai X, Liu J, et al. Circular RNA circREPS2 Acts as a Sponge of miR-558 to Suppress Gastric Cancer Progression by Regulating RUNX3/β-catenin Signaling. Mol Ther Nucleic Acids 2020;21:577-91. [Crossref] [PubMed]
- Ito K. RUNX3 in oncogenic and anti-oncogenic signaling in gastrointestinal cancers. J Cell Biochem 2011;112:1243-9. [Crossref] [PubMed]
- Song J, Liu Y, Wang T, et al. MiR-17-5p promotes cellular proliferation and invasiveness by targeting RUNX3 in gastric cancer. Biomed Pharmacother 2020;128:110246. [Crossref] [PubMed]
- Lee SH, Bae SC, Kim KW, et al. RUNX3 inhibits hypoxia-inducible factor-1α protein stability by interacting with prolyl hydroxylases in gastric cancer cells. Oncogene 2014;33:1458-67. [Crossref] [PubMed]
- Hideura E, Suehiro Y, Nishikawa J, et al. Blood Free-Circulating DNA Testing of Methylated RUNX3 Is Useful for Diagnosing Early Gastric Cancer. Cancers (Basel) 2020;12:789. [Crossref] [PubMed]
- Liu B, Han Y, Jiang L, et al. Clinicopathological and prognostic significance of the RUNX3 expression in gastric cancer: a systematic review and meta-analysis. Int J Surg 2018;53:122-8. [Crossref] [PubMed]
- Douchi D, Yamamura A, Matsuo J, et al. A Point Mutation R122C in RUNX3 Promotes the Expansion of Isthmus Stem Cells and Inhibits Their Differentiation in the Stomach. Cell Mol Gastroenterol Hepatol 2022;13:1317-45. [Crossref] [PubMed]
- Nakamura J, Tanaka T, Kitajima Y, et al. Methylation-mediated gene silencing as biomarkers of gastric cancer: a review. World J Gastroenterol 2014;20:11991-2006. [Crossref] [PubMed]
- Ebrahimi V, Soleimanian A, Ebrahimi T, et al. Epigenetic modifications in gastric cancer: Focus on DNA methylation. Gene 2020;742:144577. [Crossref] [PubMed]
- Karamitrousis EI, Balgkouranidou I, Xenidis N, et al. Prognostic Role of RASSF1A, SOX17 and Wif-1 Promoter Methylation Status in Cell-Free DNA of Advanced Gastric Cancer Patients. Technol Cancer Res Treat 2021;20:1533033820973279. [Crossref] [PubMed]
- Wang Q, Lin Y, Zhong W, et al. Regulatory Non-coding RNAs for Death Associated Protein Kinase Family. Front Mol Biosci 2021;8:649100. [Crossref] [PubMed]
- Tur MK, Daramola AK, Gattenlöhner S, et al. Restoration of DAP Kinase Tumor Suppressor Function: A Therapeutic Strategy to Selectively Induce Apoptosis in Cancer Cells Using Immunokinase Fusion Proteins. Biomedicines 2017;5:59. [Crossref] [PubMed]
- Xiang H, Zhang J, Lin C, et al. Targeting autophagy-related protein kinases for potential therapeutic purpose. Acta Pharm Sin B 2020;10:569-81. [Crossref] [PubMed]
- Li Y, Zhu M, Zhang X, et al. Clinical significance of DAPK promoter hypermethylation in lung cancer: a meta-analysis. Drug Des Devel Ther 2015;9:1785-96. [PubMed]
- Yuan W, Chen J, Shu Y, et al. Correlation of DAPK1 methylation and the risk of gastrointestinal cancer: A systematic review and meta-analysis. PLoS One 2017;12:e0184959. [Crossref] [PubMed]
- Li Y, Shi Y, Wang X, et al. Silencing of CHFR Sensitizes Gastric Carcinoma to PARP Inhibitor Treatment. Transl Oncol 2020;13:113-21. [Crossref] [PubMed]
- Scolnick DM, Halazonetis TD. Chfr defines a mitotic stress checkpoint that delays entry into metaphase. Nature 2000;406:430-5. [Crossref] [PubMed]
- Yang S, He F, Dai M, et al. CHFR promotes the migration of human gastric cancer cells by inducing epithelial-to-mesenchymal transition in a HDAC1-dependent manner. Onco Targets Ther 2019;12:1075-84. [Crossref] [PubMed]
- Grady WM. Epigenetic alterations in the gastrointestinal tract: Current and emerging use for biomarkers of cancer. Adv Cancer Res 2021;151:425-68. [Crossref] [PubMed]
- Palanca-Ballester C, Rodriguez-Casanova A, Torres S, et al. Cancer Epigenetic Biomarkers in Liquid Biopsy for High Incidence Malignancies. Cancers (Basel) 2021;13:3016. [Crossref] [PubMed]
- Bates SE. Epigenetic Therapies for Cancer. N Engl J Med 2020;383:650-63. [Crossref] [PubMed]
- Topper MJ, Vaz M, Marrone KA, et al. The emerging role of epigenetic therapeutics in immuno-oncology. Nat Rev Clin Oncol 2020;17:75-90. [Crossref] [PubMed]
- Lu Y, Chan YT, Tan HY, et al. Epigenetic regulation in human cancer: the potential role of epi-drug in cancer therapy. Mol Cancer 2020;19:79. [Crossref] [PubMed]