Hesperetin ameliorates glioblastoma by inhibiting proliferation, inducing apoptosis, and suppressing metastasis
Introduction
Glioblastoma, the most common malignant tumor of the brain, is characterized by rapid progression and poor prognosis (1). According to the CBTRUS Statistical Report: Primary Brain, approximately 9.23 individuals per 100,000 have glioblastoma in the United States (2). Although the survival rate has improved owing to progress in available treatment strategies, the 5-year survival rate remains only 5.8% (2-4). Patients newly diagnosed with glioblastoma undergo standard treatments, including postoperative radiotherapy with temozolomide (TMZ) and adjuvant temozolomide treatment. However, no standard treatment for managing recurrence is currently available, and various treatments (such as cytotoxic drugs and bevacizumab) are routinely employed. In addition to classic treatments, such as surgery, radiotherapy, and chemotherapy, novel therapeutic options, such as immunotherapy and precision oncology, have also been explored (1). Despite marked improvements in glioblastoma treatments, the 5-year survival rate remains ˂10% (5), strongly indicating that specific drugs and therapeutic strategies targeting glioblastoma are needed.
Malignant tumors are characterized by malignant hyperplasia and a strong tendency toward metastasis. Metastasis, including epithelial-mesenchymal transition (EMT), invasion, and migration, is the primary factor contributing to cancer-related deaths (6). Growing number of evidence shows that EMT is vital for cancer physiological processes. EMT is connected with enhanced invasion and malignancy of tumor cells. EMT can be used to elevate the invasion in glioblastoma cells. Besides, EMT induces drug resistance and is related to the recurrence of cancer cells (6). Therefore, antimetastatic properties of anticancer drugs should be considered during their development. In addition, as the blood-brain barrier (BBB) protects the brain from harmful toxins in the blood but restricts anticancer drugs from targeting brain tumors, crossing this BBB is a major challenge in the development of antitumor drugs.
Several epidemiological and experimental studies have shown that dietary phytochemicals exert chemopreventive and chemotherapeutic effects (7-10). In recent years, several natural products [alkaloids, such as berberine (8), flavonoids, such as curcumin (9), and silibinin (10)] isolated from Chinese herbal product have been found to inhibit proliferation, induce apoptosis, and delay metastasis, exhibiting anticancer potential in vitro and in vivo (11). Hesperetin (3',5,7-trihydroxy-4'-methoxyflavanone; HSP), a type of flavanone (a subclass of flavonoids), is mainly found in citrus fruits. It is an important bioactive ingredient of the traditional Chinese herb known as Chen Pi in Chinese. In recent years, HSP has been gained considerable attention owing to its various biological properties, which confer anticancer (12), cardioprotective (13), and antidiabetic effects (14). In addition, in vitro models have shown that HSP is capable of traversing the BBB with facility (15). Li et al. (16) have reported that HSP can induce apoptosis in human glioblastoma cells via p38 mitogen-activated protein kinase (MAPK) activation. Ersoz et al. (17) have shown that HSP exerts anti-proliferative, anti-oxidant, and pro-apoptotic properties in C6 glioma cancer cells. However, few studies have explored the effect of HSP on glioblastoma invasion and migration.
In the present study, we evaluated the anticancer activity of HSP using mouse glioblastoma cells (GL261) and explored the related underlying mechanisms (antiproliferation, proapoptosis, and particularly antimetastasis). The results may provide a deeper insight into the mechanisms of Hsp and shows the gaps in our knowledge about Hsp. The findings drawn from this research may contribute to a more comprehensive understanding of the anticancer effects of HSP and thus facilitate the future development of glioblastoma treatment. We present the following article in accordance with the MDAR reporting checklist (available at https://tcr.amegroups.com/article/view/10.21037/tcr-22-1497/rc).
Methods
Materials
HSP [analysis standard: high performance liquid chromatography (HPLC) ≥98%] was purchased from Shanghai Yuanye Bio-Technology Co., Ltd (Shanghai, China) (Figure 1A). Phosphate-buffered saline, dimethyl sulfoxide, fetal bovine serum (FBS), and Dulbecco’s modified Eagle’s medium (DMEM) were purchased from Gibco-Invitrogen (Carlsbad, CA, USA). The cell culture freezing medium was purchased from Procell Life Science & Technology (Wuhan, Hubei, China). Phosphoinositide 3-kinase (PI3K), Phosphorylated- PI3K (p-PI3K), protein kinase B (AKT), p-AKT, nuclear factor-kappa B (NF-κB), p-NF-κB, glyceraldehyde-3-phosphate dehydrogenase (GAPDH), poly (ADP-ribose) polymerase (PARP), caspase-3, cleaved-caspase-3, E-cadherin, N-cadherin, vimentin, matrix metalloproteinase-2 (MMP-2), MMP-9, and goat anti-mouse IgG (H+L) antibodies were purchased from ABclonal Technology (Boston, MA, USA). Cell Counting Kit-8 (CCK-8) was purchased from Glpbio (Montclair, CA, USA). Crystal violet staining solution and Hoechst 33342 were obtained from Beyotime Biotechnology (Shanghai, China). The Annexin V-APC/ propidine iodide (PI) apoptosis kit was purchased from KeyGEN BioTECH (Nanjing, Jiangsu, China).
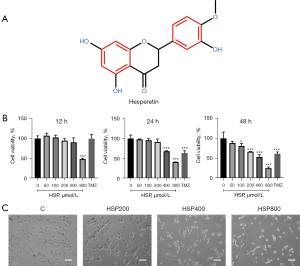
Cell culture
GL261 cells were purchased from the American Type Culture Collection (Manassas, VA, USA) and cultured in DMEM, supplemented with 10% FBS, 100 µg/mL of streptomycin, and 100 U/mL of penicillin.
CCK-8 assay
Cell viability was assessed with CCK-8 assay. GL261 cells in the growth phase were plated in 96-well culture plates (8×103 cells/well). The cells were then treated with HSP at indicated concentrations for 24 or 48 h. After addition of the CCK-8 solution to the medium for 1 h, a microplate reader (Molecular Devices, Sunnyvale, CA, USA) was used to measure the absorbance for the evaluation of cell viability.
Hoechst staining assay
GL261 cells were plated into 6-well culture plates and treated with HSP at indicated concentrations for 24 h. The cells were then washed twice, stained with Hoechst 33342 for 30 min, washed twice again, and then observed under a fluorescence microscope. Apoptotic cell nuclei appeared shrunken and were deeply stained under fluorescence microscope examination.
Apoptosis detection assay
The apoptosis rate was determined using an Annexin V-APC/PI apoptosis kit (eBioscience; Thermo Fisher Scientific, Inc.). GL261 cells were treated with HSP at indicated concentrations for 24 h. The cells were then harvested and stained with Annexin V-APC and propidium iodide (PI). The apoptosis rate was calculated using a flow cytometer and FlowJo software (version 10; BD, Franklin Lakes, NJ, USA).
Western blotting
After treatment with HSP at indicated concentrations for 24 h, the cells were harvested and lysed with RIPA buffer containing protease and phosphatase inhibitors for the isolation of total protein. Meanwhile, western blotting was performed to measure the expression of a target protein. Briefly, proteins were separated and transferred to polyvinylidene fluoride membranes. Next, membranes were blocked for 1 h and incubated with primary antibodies overnight at 4 ℃. After incubation with horseradish peroxidase-conjugated secondary antibodies for 2 h, immunoblots were observed using an enhanced chemiluminescence (ECL) 3' labeling kit (ECL) detection reagent (Thermo Fisher Scientific, Inc.). Images were acquired using a Tanon-S200 imaging system (Olympus, Singapore). ImageJ software (National Institutes of Health, Bethesda, MD, USA) was used to analyze the expression of the target protein.
Wound-Healing assay
The GL261 cells were plated into 6-well plates and cultured until 80–90% confluency was achieved. A pipette tip was used to scratch a wound in a well, and washing was performed to remove unattached cells. After the cells were treated with HSP at indicated concentrations, the wounds were photographed at 0, 24, and 48 h. The migration area was calculated using the following formula:
where M0 represents the initial wound area and Mn represents the wound area at the measurement point.
Transwell assay
The invasion was observed on GL261 cells using Matrigel-coated Transwells (BD Biosciences). After treatment with HSP at indicated concentrations for 24 h, the cells were seeded into the upper chamber with DMEM and 5% FBS, and DMEM supplemented with 20% FBS was added to the lower chamber. After incubation for 24 h, the cells were fixed with paraformaldehyde (4%), stained with crystal violet (0.5%), and photographed under a microscope.
Statistical analysis
Data are presented as the mean ± standard deviation (SD). Statistical analysis was performed using 1-way analysis of variance (ANOVA), and Duncan’s multiple range test was used to compare mean values. Statistical significance was set at P<0.05.
Results
HSP inhibited cell proliferation
Cell viability was measured using the CCK8 assay. We observed that HSP inhibited cell proliferation in a time- and concentration-dependent manner (Figure 1B). Treatment of GL261 cells with 800 µM of HSP for 12 h was found to significantly suppress cell viability (P<0.001); treatment with 400 and 100 µM of HSP for 24 h was also found to significantly suppress cell viability (P<0.001, P<0.05). Moreover, cell viability was more suppressed after treatment with 800 µM of HSP than after treatment with temozolomide (400 µg/mL) regardless of the duration of treatment.
Furthermore, we found that the number of GL261 cells decreased with increased HSP concentration (Figure 1C). In addition, GL261 cells were found to be shrunken and round after HSP treatment, indicating that HSP induced apoptosis. These findings provide evidence that HSP has antiglioblastoma effects and is a potential antiglioblastoma drug.
HSP induced cell apoptosis
Changes in cell morphology indicate apoptosis. As shown in Figure 2A, the treatment of GL261 cells with HSP resulted in shrunken and densely stained nuclei, which suggested that HSP induced apoptosis. In addition, HSP increased the apoptosis rate in a concentration-dependent manner (Figure 2B,2C), with 200 µM of HSP increasing the apoptosis rate significantly (P<0.05). Finally, 400 µM of HSP induced apoptosis in 22.73% (SD 1.73%) of GL261 cells, while 800 µM of HSP induced apoptosis in 27.50% (SD 2.56%) of GL261 cells (Figure 2C).
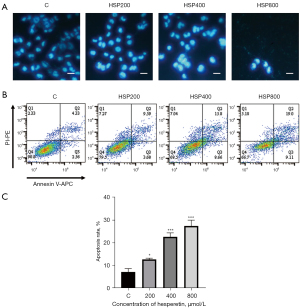
HSP-induced apoptosis through the PI3K/AKT and NF-κB pathways
Apoptosis is not a passive process and is regulated by a series of genes or proteins, including the caspase family. Western blotting results revealed that increasing concentrations of HSP upregulated the expression levels of cleaved caspase-3 and cleaved-PARP. The ratio of BAX to BCL-2 increased with the increase of HSP concentration (Figure 3A). These results further confirmed HSP-induced apoptosis in GL261 cells, indicating that an intrinsic pathway mediated HSP-induced apoptosis.
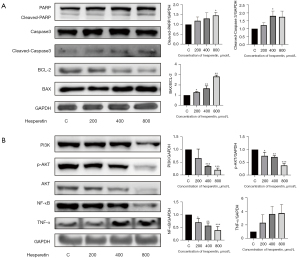
Moreover, the treatment of GL261 cells with HSP for 24 h significantly downregulated the expression of PI3K, AKT, and p-AKT (Figure 3B). These results suggested that HSP-induced apoptosis may occur through the PI3K/AKT pathway. In addition, the expression of NF-κB was downregulated after treatment with increasing concentrations of HSP; however, the expression of tumor necrosis factor-α (TNF-α) was upregulated (Figure 3B), thus indicating that HSP-induced apoptosis may be mediated via the NF-κB pathway. On the basis of these findings, we speculated upon the possible signaling pathways that mediate HSP-induced apoptosis (Figure 4).
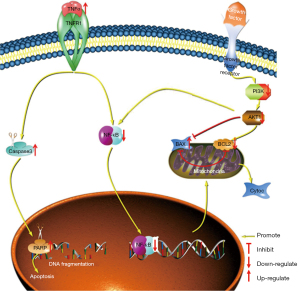
HSP inhibited cell migration and invasion
Along with malignant hyperplasia, migration and invasion are the critical characteristics of tumors. The migration ability of GL261 cells treated with HSP at the indicated concentrations was assessed with a wound-healing assay. As shown in Figure 5A,5B, treatment with HSP significantly inhibited wound healing. The size of the wound area was increased after 24 and 48 h of treatment with 800 µM of HSP. These results indicated that HSP suppressed cell migration. The Transwell assay was used to measure invasive ability. We observed that the number of cells that passed through the Matrigel-coated Transwell chamber significantly decreased with the increase of HSP concentration (Figure 5C,5D). Matrigel-coated Transwell chambers were further used to mimic the extracellular matrix, with the results suggesting that HSP inhibited cell invasion.
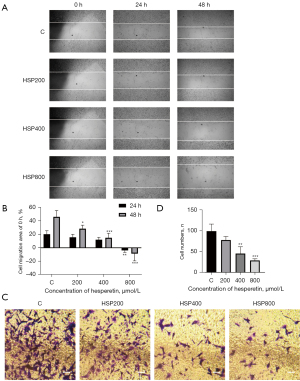
HSP downregulated the expression of MMP-2 and MMP-9
MMPs play a vital role in tumor invasion and metastasis by degrading various protein components in the extracellular matrix, with MMP-2 and MMP-9 being the more well-known MMPs. As shown in Figure 6, the expression levels of MMP-2 and MMP-9 were significantly downregulated with increased HSP concentration, indicating that HSP inhibited cell migration and invasion.
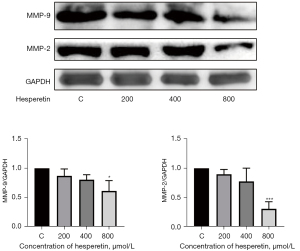
HSP suppressed EMT
EMT is an important biological process regulated by multiple genes and proteins, such as E-cadherin, N-cadherin, and vimentin, resulting in malignant tumors with invasive and metastatic capabilities. Decreased E-cadherin expression weakens cell adhesion, whereas increased vimentin and N-cadherin expression levels endow cells with a mesenchymal phenotype. We observed that increasing HSP concentration enhanced E-cadherin expression while suppressing N-cadherin and vimentin expression levels (Figure 7). In addition, HSP suppressed EMT.
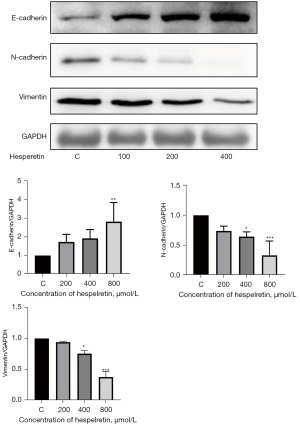
Discussion and conclusions
Glioblastoma is one of the most malignant adult brain tumors, remains incurable with a bleak median survival (1-4). In spite of the development of therapeutic strategies for glioblastoma treatment were improved, the glioblastoma patients still have a poor prognosis. For now there is a few FDA (such as TMZ) approved drugs for its treatment, which are not universally efficacious. This is due to the high malignancy and invasiveness of glioblastoma cells. Therefore, further understanding the molecular mechanism and appropriate prognostic markers and therapeutic targets is necessary. The progress of novel anti-tumor drugs, such as natural products, can improve the efficacy.
Hsp is citrus flavonoids with several advantages, such as its low cost, convenience, and safety. Hsp has gained much attention due to its various biological activities including anticancer, anti-inflammatory, neuroprotective, cardioprotection and antioxidant properties (12,14,18). Notably, administration a high amount of Hsp is not associated with a substantial increase in the half-life of HSP and it is not fully figure out that how Hsp exerts great therapeutic effects (12).
Glioblastoma tend to recur and obstruct the BBB, and therapeutic options for treating glioblastoma are limited. One potential treatment, HSP, possesses a variety of anticancer effects (16-19). For instance, using in vitro models, Youdim et al. (15) revealed that HSP can cross the BBB. With this in mind, we further assessed the antiglioblastoma activity of HSP and explored the underlying mechanism in GL261 cells. We observed that HSP exerted an antiglioblastoma effect on GL261 cells and that HSP suppressed cell proliferation. In addition, HSP induced apoptosis, with this process possibly being regulated through the PI3K/AKT and NF-κB pathways. HSP also inhibited cell migration and invasion by downregulating the expression of MMP-2 and MMP-9. Furthermore, HSP inhibited EMT by upregulating the expression of E-cadherin and downregulating the expression of N-cadherin and vimentin. It has been demonstrated that a number of targets and signaling pathways are involved in the progression and malignancy of cancer cells in previous studies, including NF-κB, TNF-α, IL-1β, IL-6, Nrf2, p38, COX-2, MMP2 and MMP9 and so on (7,12,16,18). And they may serve as new therapeutic targets for glioblastoma treatment.
Induction of apoptosis is the primary mechanism through which anticancer drugs kill tumor cells (20), and apoptotic signaling pathways are mainly divided into extrinsic and intrinsic pathways. The extrinsic pathway is initiated by the binding of the death ligand to the death receptor (e.g., TNF-α) (21), while the intrinsic pathway is initiated by various intracellular stimuli (e.g., DNA damage) and is chiefly regulated by BCL-2 family members (22). Several factors regulate apoptosis, including PI3K/AKT (23) and NF-κB (24). However, once apoptosis occurs, the cell eventually collapses by activating a member of the caspase family, such as caspase-3 (20). In the present study, HSP was found to upregulate the expression of cleaved-caspase-3 and cleaved-PARP, which were cleaved by caspase-3. In addition, HSP increased the ratio of BAX to BCL-2, indicating that HSP induced apoptosis through an intrinsic pathway.
The PI3K/AKT pathway is a classic signaling pathway associated with cell proliferation, differentiation, and apoptosis, and flavonoids can induce apoptosis through the PI3K/AKT pathway (25,26). He et al. (27) reported that HSP could prevent hypoxia/reoxygenation injury in rat cardiomyocytes in vitro by activating the PI3K/Akt signaling pathway. Similarly, our results showed that HSP downregulated the expression of PI3K and AKT (p-AKT), indicating that HSP might induce apoptosis via the PI3K/AKT signaling pathway.
NF-κB plays a key regulatory role in cytokine-induced gene expression and participates in regulating inflammation, apoptosis, and tumorigenesis by modulating the expression of multiple genes (28). Studies have shown that flavonoids exert anti-inflammatory and anticancer effects by regulating the NF-κB signaling pathway (25,29). Hazafa et al. (30) reported that flavonoids (including catechin, quercetin, and HSP) could inhibit cell proliferation by suppressing the NF-κB pathway in various cancers. In our study, HSP induced apoptosis, upregulated TNF-α expression, and downregulated NF-κB expression. These results indicate that HSP might also induce apoptosis via the NF-κB signaling pathway. Moreover, some studies have reported that NF-κB is the downstream molecule in regulating apoptosis in the PI3K/Akt signaling pathway (25,31). Considering these findings collectively, we speculated upon the potential mechanism underlying HSP-induced apoptosis in GL261 cells (Figure 4). The metastasis of malignant tumors typically leads to a poor prognosis and a low survival rate. Therefore, metastasis is often considered the main reason for tumor treatment failure and the primary hindrance to the development of anticancer drugs (6). Tumor metastasis usually involves the following steps (32): (I) EMT endows tumor cells with invasive and migration properties, (II) tumor cells invade the basement membrane, and (III) tumor cells migrate to other sites or tissues. Numerous studies have indicated that flavonoids exhibit antimetastatic properties via the regulation of those signaling pathways associated with cell migration and invasion (33-38). For example, Fan et al. (34) demonstrated that luteolin and quercetin could inhibit migration and invasion of squamous carcinoma by suppressing the Src/Stat3/S100A7 signaling pathway. In addition, Wang et al. (35) reported that HSP could inhibit gastric cancer metastasis. In the present study, our results revealed that HSP inhibited cell migration and invasion by downregulating the expression of MMP-2 and MMP-9 EMT plays an important role in tumor metastasis and is mainly regulated by E-cadherin, N-cadherin, and vimentin (36). Choi et al. (37) reported that HSP can inhibit EMT via the mTOR pathway in EMT-elicited podocytes, while Wang et al. (38) showed that HSP suppresses EMT by upregulating E-cadherin expression and downregulating N-cadherin expression in cervical cancer cells. Similarly, in the present study, HSP inhibited EMT by upregulating E-cadherin expression and downregulating N-cadherin and vimentin expression, consequently resulting in reduced migration and invasion of glioblastoma cells. Integrating these results with those related to tumor metastasis, we speculated upon the potential antimetastasis mechanism of HSP in GL261 cells (Figure 8).
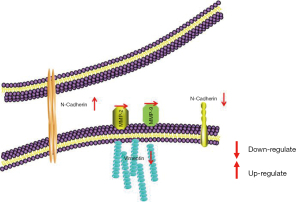
In summary, we observed that HSP ameliorated glioblastoma by inhibiting proliferation, inducing apoptosis, and suppressing metastasis. HSP is a food-derived bioactive ingredient with several advantages, such as its low cost, convenience, and safety. In addition, in vitro models demonstrated that HSP could easily cross the BBB . Therefore, HSP may be an effective preventive and therapeutic antiglioblastoma drug that is worth developing as a therapeutic option for patients with recurrent glioblastoma.
Acknowledgments
Funding: This work was supported by the Medical Scientific Research Foundation of Guangdong Province, China (No. B2021398).
Footnote
Reporting Checklist: The authors have completed the MDAR reporting checklist. Available at https://tcr.amegroups.com/article/view/10.21037/tcr-22-1497/rc
Data Sharing Statement: Available at https://tcr.amegroups.com/article/view/10.21037/tcr-22-1497/dss
Conflicts of Interest: All authors have completed the ICMJE uniform disclosure form (available at https://tcr.amegroups.com/article/view/10.21037/tcr-22-1497/coif). The authors have no conflicts of interest to declare.
Ethical Statement: The authors are accountable for all aspects of the work in ensuring that questions related to the accuracy or integrity of any part of the work are appropriately investigated and resolved.
Open Access Statement: This is an Open Access article distributed in accordance with the Creative Commons Attribution-NonCommercial-NoDerivs 4.0 International License (CC BY-NC-ND 4.0), which permits the non-commercial replication and distribution of the article with the strict proviso that no changes or edits are made and the original work is properly cited (including links to both the formal publication through the relevant DOI and the license). See: https://creativecommons.org/licenses/by-nc-nd/4.0/.
References
- Tan AC, Ashley DM, López GY, et al. Management of glioblastoma: State of the art and future directions. CA Cancer J Clin 2020;70:299-312. [Crossref] [PubMed]
- Ostrom QT, Gittleman H, Truitt G, et al. CBTRUS Statistical Report: Primary Brain and Other Central Nervous System Tumors Diagnosed in the United States in 2011-2015. Neuro Oncol 2018;20:iv1-iv86. [Crossref] [PubMed]
- Ostrom QT, Cote DJ, Ascha M, et al. Adult Glioma Incidence and Survival by Race or Ethnicity in the United States From 2000 to 2014. JAMA Oncol 2018;4:1254-62. [Crossref] [PubMed]
- Ostrom QT, Gittleman H, Farah P, et al. CBTRUS statistical report: Primary brain and central nervous system tumors diagnosed in the United States in 2006-2010. Neuro Oncol 2013;15:ii1-56. [Crossref] [PubMed]
- Stupp R, Hegi ME, Mason WP, et al. Effects of radiotherapy with concomitant and adjuvant temozolomide versus radiotherapy alone on survival in glioblastoma in a randomised phase III study: 5-year analysis of the EORTC-NCIC trial. Lancet Oncol 2009;10:459-66. [Crossref] [PubMed]
- Steeg PS. Targeting metastasis. Nat Rev Cancer 2016;16:201-18. [Crossref] [PubMed]
- Lee JH, Khor TO, Shu L, et al. Dietary phytochemicals and cancer prevention: Nrf2 signaling, epigenetics, and cell death mechanisms in blocking cancer initiation and progression. Pharmacol Ther 2013;137:153-71. [Crossref] [PubMed]
- Choi MS, Yuk DY, Oh JH, et al. Berberine inhibits human neuroblastoma cell growth through induction of p53-dependent apoptosis. Anticancer Res 2008;28:3777-84. [PubMed]
- López-Lázaro M. Anticancer and carcinogenic properties of curcumin: considerations for its clinical development as a cancer chemopreventive and chemotherapeutic agent. Mol Nutr Food Res 2008;52:S103-27. [Crossref] [PubMed]
- Singh RP, Raina K, Sharma G, et al. Silibinin inhibits established prostate tumor growth, progression, invasion, and metastasis and suppresses tumor angiogenesis and epithelial-mesenchymal transition in transgenic adenocarcinoma of the mouse prostate model mice. Clin Cancer Res 2008;14:7773-80. [Crossref] [PubMed]
- Tan W, Lu J, Huang M, et al. Anti-cancer natural products isolated from chinese medicinal herbs. Chin Med 2011;6:27. [Crossref] [PubMed]
- Salehi B, Cruz-Martins N, Butnariu M, et al. Hesperetin's health potential: moving from preclinical to clinical evidence and bioavailability issues, to upcoming strategies to overcome current limitations. Crit Rev Food Sci Nutr. 2022;62:4449-64. [Crossref] [PubMed]
- Trivedi PP, Kushwaha S, Tripathi DN, et al. Cardioprotective effects of hesperetin against doxorubicin-induced oxidative stress and DNA damage in rat. Cardiovasc Toxicol 2011;11:215-25. [Crossref] [PubMed]
- Guo J, Tao H, Cao Y, et al. Prevention of Obesity and Type 2 Diabetes with Aged Citrus Peel (Chenpi) Extract. J Agric Food Chem 2016;64:2053-61. [Crossref] [PubMed]
- Youdim KA, Dobbie MS, Kuhnle G, et al. Interaction between flavonoids and the blood-brain barrier: in vitro studies. J Neurochem 2003;85:180-92. [Crossref] [PubMed]
- Li Q, Miao Z, Wang R, et al. Hesperetin Induces Apoptosis in Human Glioblastoma Cells via p38 MAPK Activation. Nutr Cancer 2020;72:538-45. [Crossref] [PubMed]
- Ersoz M, Erdemir A, Duranoglu D, et al. Comparative evaluation of hesperetin loaded nanoparticles for anticancer activity against C6 glioma cancer cells. Artif Cells Nanomed Biotechnol 2019;47:319-29. [Crossref] [PubMed]
- Roohbakhsh A, Parhiz H, Soltani F, et al. Molecular mechanisms behind the biological effects of hesperidin and hesperetin for the prevention of cancer and cardiovascular diseases. Life Sci 2015;124:64-74. [Crossref] [PubMed]
- Nalini N, Aranganathan S, Kabalimurthy J. Chemopreventive efficacy of hesperetin (citrus flavonone) against 1,2-dimethylhydrazine-induced rat colon carcinogenesis. Toxicol Mech Methods 2012;22:397-408. [Crossref] [PubMed]
- Pistritto G, Trisciuoglio D, Ceci C, et al. Apoptosis as anticancer mechanism: function and dysfunction of its modulators and targeted therapeutic strategies. Aging (Albany NY) 2016;8:603-19. [Crossref] [PubMed]
- Guicciardi ME, Gores GJ. Life and death by death receptors. FASEB J 2009;23:1625-37. [Crossref] [PubMed]
- Green DR, Kroemer G. The pathophysiology of mitochondiral cell death. Science 2004;305:626-9. [Crossref] [PubMed]
- Li Y, Xia J, Jiang N, et al. Corin protects H2O2-induced apoptosis through PI3K/AKT and NF-κB pathway in cardiomyocytes. Biomed Pharmacother 2018;97:594-9. [Crossref] [PubMed]
- He M, Zhang Y, Xie F, et al. Role of PI3K/Akt/NF-κB and GSK-3β pathways in the rat model of cardiopulmonary bypass-related lung injury. Biomed Pharmacother 2018;106:747-54. [Crossref] [PubMed]
- Lee HJ, Nagappan A, Park HS, et al. Flavonoids isolated from Citrus platymamma induce mitochondrial-dependent apoptosis in AGS cells by modulation of the PI3K/AKT and MAPK pathways. Oncol Rep 2015;34:1517-25. [Crossref] [PubMed]
- Zhang HW, Hu JJ, Fu RQ, et al. Flavonoids inhibit cell proliferation and induce apoptosis and autophagy through downregulation of PI3Kγ mediated PI3K/AKT/mTOR/p70S6K/ULK signaling pathway in human breast cancer cells. Sci Rep 2018;8:11255. [Crossref] [PubMed]
- He S, Wang X, Zhong Y, et al. Hesperetin post-treatment prevents rat cardiomyocytes from hypoxia/reoxygenation injury in vitro via activating PI3K/Akt signaling pathway. Biomed Pharmacother 2017;91:1106-12. [Crossref] [PubMed]
- Zhang Q, Lenardo MJ, Baltimore D. 30 Years of NF-κB: A Blossoming of Relevance to Human Pathobiology. Cell 2017;168:37-57. [Crossref] [PubMed]
- Peng L, Gao X, Nie L, et al. Astragalin Attenuates Dextran Sulfate Sodium (DSS)-Induced Acute Experimental Colitis by Alleviating Gut Microbiota Dysbiosis and Inhibiting NF-κB Activation in Mice. Front Immunol 2020;11:2058. [Crossref] [PubMed]
- Hazafa A, Rehman KU, Jahan N, et al. The Role of Polyphenol (Flavonoids) Compounds in the Treatment of Cancer Cells. Nutr Cancer 2020;72:386-97. [Crossref] [PubMed]
- Ranjani S, Kowshik J, Sophia J, et al. Activation of PI3K/Akt/NF-kB Signaling Mediates Swedish Snus Induced Proliferation and Apoptosis Evasion in the Rat Forestomach: Modulation by Blueberry. Anticancer Agents Med Chem 2020;20:59-69. [Crossref] [PubMed]
- Wan L, Pantel K, Kang Y. Tumor metastasis: moving new biological insights into the clinic. Nat Med 2013;19:1450-64. [Crossref] [PubMed]
- Liskova A, Koklesova L, Samec M, et al. Flavonoids in Cancer Metastasis. Cancers (Basel) 2020;12:1498. [Crossref] [PubMed]
- Fan JJ, Hsu WH, Lee KH, et al. Dietary Flavonoids Luteolin and Quercetin Inhibit Migration and Invasion of Squamous Carcinoma through Reduction of Src/Stat3/S100A7 Signaling. Antioxidants (Basel) 2019;8:557. [Crossref] [PubMed]
- Wang SW, Sheng H, Zheng F, et al. Hesperetin promotes DOT1L degradation and reduces histone H3K79 methylation to inhibit gastric cancer metastasis. Phytomedicine 2021;84:153499. [Crossref] [PubMed]
- Mittal V. Epithelial Mesenchymal Transition in Tumor Metastasis. Annu Rev Pathol 2018;13:395-412. [Crossref] [PubMed]
- Choi D, Kim CL, Kim JE, et al. Hesperetin inhibit EMT in TGF-β treated podocyte by regulation of mTOR pathway. Biochem Biophys Res Commun 2020;528:154-9. [Crossref] [PubMed]
- Wang J, Chen H, Hu Z, et al. Hesperetin regulates transforming growth factor-β1/Smads pathway to suppress epithelial-mesenchymal transition -mediated invasion and migration in cervical cancer cell. Anticancer Drugs 2021;32:930-8. [Crossref] [PubMed]