Effects of gold nanoparticles on normal hepatocytes in radiation therapy
Introduction
Nano-sized materials, with a size similar to that of most biological molecules, have substantial potential for a wide variety of applications in the biomedical field, especially in terms of treatment options for serious medical challenges. Recently, the addition of metal-based nanoparticles to standard radiation therapy has been proposed as a novel strategy to improve the therapeutic index in cancer treatment (1). Among these nanomaterials, gold nanoparticles (GNP, AuNPs) have received substantial attention as radiosensitizers owing to their attractive properties such as good biocompatibility, chemical stability, ease of surface modification, and high X-ray absorption coefficients (2), along with their tunable size, unique surface chemistry, electronic properties (3), and low osmolality (4). Several studies have suggested that the biological mechanisms of AuNP radiosensitization may include triggering cell cycle arrest and oxidative stress-mediated apoptosis, necrosis, or DNA damage (2,5). It is notable that the efficacy of AuNPs as a potential radiation sensitizer has been determined in multiple cancer cell types, including in vitro and in vivo studies, and using various radiation sources, through simulations with Monte Carlo calculations (2,6-9). To date, however, data are lacking on the efficacy of using AuNPs alone and/or in combination with radiation for improving treatment outcomes of hepatocellular carcinoma (HCC) without high cytotoxicity. Very few studies have evaluated the normal in vitro toxicity of AuNPs at megavoltage energies on hepatocytes, which is necessary to provide a scientific rationale for using AuNPs with radiotherapy.
Normal hepatocytes are sensitive to X-ray radiation; high-dose radiation therapy causes hepatic necrosis (10,11). Thus, radiation treatment should be planned to avoid damaging normal liver tissue as much as possible. It is possible to reduce several side effects when irradiating with low doses in radiation therapy. Previous studies demonstrated that AuNPs have a radiosensitizing effect on HCC both in vitro and in vivo (7,12). However, these studies did not report the effect of the AuNPs on normal tissue damage in combination with radiation therapy. In particular, the effect of AuNPs on sensitization of hepatocyte cells to radiation at clinically relevant kilovoltage energies has not been demonstrated to date.
Nanoparticles deposited in liver tissue may affect normal physiological and biochemical functioning by influencing liver parenchymal cells and other constituent cells of the liver (13). The hepatic lobule is the basic functional unit of the liver, comprising 60% solid cells (hepatocytes) and 30–35% non-solid cells (hepatic stellate cells, Kupffer cells, and sinusoidal endothelial cells) (14-17). Therefore, the aim of this study was to evaluate the in vitro toxicity of AuNPs at kilovoltage energies on normal hepatocytes and hepatic stellate cells, which can provide a scientific rationale for the use of AuNPs with radiotherapy in the treatment of HCC. We present the following article in accordance with the MDAR reporting checklist (available at https://tcr.amegroups.com/article/view/10.21037/tcr-21-1855/rc).
Methods
Cell culture
Human hepatocyte cells (HH) were obtained from Sciencell Research Laboratories (Catalog #5200; Carlsbad, CA, USA) and cultured in hepatocyte medium supplemented with 5% fetal bovine serum (FBS), 1% penicillin and streptomycin, and 1% hepatocyte growth supplement (all from Sciencell). The cells were grown at 37 ℃ in 5% CO2, 0.1% hepatocyte growth supplement, and 0.1% penicillin-streptomycin solution. Human Hepatic stellate cells (LX-2; Catalog #SCC064) were provided by Professor Yongju Park, College of Pharmacy, Kyungsung University, and cultured in Dulbecco’s Modified Eagle Medium (WelGene, Daegu, Korea) containing 10 % (v/v) FBS and 1% (v/v) penicillin-streptomycin (both from Gibco® Life Technologies, Carlsbad, CA, USA). Huh7 and HepG2 HCC cells were cultured in RPMI 1640 medium (WelGene, Daegu, Korea), supplemented with 10% (v/v) FBS (Lonza, MD, USA) and 1% (v/v) penicillin-streptomycin (Lonza, MD, USA) at 37 ℃ in a humidified incubator containing 5% CO2.
AuNPs
AuNPs, about 5 nm in diameter, were purchased from Cytodiagnostics (Burlington, ON, Canada).
Transmission electron microscope (TEM) studies
The cellular uptake of AuNPs was observed using TEM. Before exposure to AuNP, HH cells at a concentration of 1×106 cells/dish (60 mm; Corning Incorporated, Corning, NY, USA) were incubated for a 24-h period. Following integrated incubation with AuNPs for the same period, TEM analysis was performed. At the initial stage, the cells were kept for 2 h in 1% osmium tetroxide before undergoing a dehydration process using ethanol with ascending concentrations for a quarter of an hour. This was followed by embedding the cells in a resin at 80 ℃ for propylene oxide polymerization. The final stage was TEM analysis of ultrathin section samples.
Irradiation
Dishes measuring 60 mm were used to plate the cells before undergoing incubation in a humidified incubator at 5% CO2 and 37 ℃. Cells were then developed to between 70% and 80% confluency. Irradiation was performed using an X-Rad 320 (Precision X-ray, Inc., USA) operating at 225 kV, 13 mA, and with a 3.45 Gy/min dose rate at a 1 to 5 GY irradiation intensity.
Water-soluble tetrazolium (WST-1) cytotoxicity assay
The cytotoxicity assay for the cells was performed using cells cultured at a density of 1×103 cells per well in 96-well plates. This was followed by irradiation with the AuNPs for different periods. The WST-1 cytotoxicity assay (Roche Diagnostics, Laval, Quebec, Canada) was performed according to the manufacturer’s instructions. Cell viability was assessed by determining the initial absorbance value of the cell culture media at 450 nm, and absorbance at 2 h after the addition of WST-1. The results are presented as the absorbance value percentage of the untreated control cells. This was defined as 100% cell viability using the following formula: (1 − Aexp/Acon) × 100. Aexp and Acon denote the absorbance values of the experimental and control groups, respectively.
Clonogenic assay
The cells were preincubated with 1 mM AuNPs for 24 h before radiation exposure and then further incubated for 14–20 days. Colonies that formed were stained with 0.4% crystal violet (Sigma-Aldrich, St. Louis, MO, USA). Plating efficiency (PE) was calculated as the percentage of seeded cells that grew into colonies. The survival fraction, expressed as a function of irradiation, was calculated as follows: (colonies counted/cells seeded × PE) × 100.
Detection of apoptotic cells by annexin V staining
Following exposure to AuNPs, the cells were subjected to 48-h radiation treatment, as described previously. This was followed by an ice-cold phosphate-buffered saline wash, trypsinization, and resuspension in 1× binding buffer (10 mM HEPES/NaOH pH 7.4, 140 mM NaCl, and 2.5 mM CaCl2) at 1×106 cells/mL. Aliquots (100 µL) of the cell solution were prepared. They were then mixed with 5 µL annexin V-fluorescein isothiocyanate (BD PharMingen, Franklin Lakes, NJ, USA) and 10 µL propidium iodide (PI) stock solution (50 µg/mL in PBS) using gentle vortexing. The next stage was 15-min incubation in the dark at room temperature. The cells were resuspended in 1× binding buffer (400 µL) before analysis using a FACScan flow cytometer (BD Biosciences, Franklin Lakes, NJ, USA). No less than 10,000 cells were identified in every sample, and the proportion of apoptotic cells was determined using the CellQuest software (BD Biosciences).
Western blotting
Normal hepatocytes were exposed to AuNPs for 24 h and 48 h before radiation treatment. Cell lysis was performed using a radio-immunoprecipitation assay buffer. Equal amounts of protein were separated by sodium dodecyl sulfate-polyacrylamide gel electrophoresis before being transferred to nitrocellulose membranes. A solution of 1% (v/v) non-fat dry milk in Tris-buffered saline containing 0.05% Tween 20 was used to block the membranes. The cells were then incubated with the listed antibodies (1:1,000 dilution). Antibodies targeting cyclin B and β-actin from Santa Cruz Biotechnology (Santa Cruz, CA, USA); antibodies against cleaved caspase-3 and poly (ADP-ribose) polymerase (PARP) from Cell Signaling Technology (Danvers, MA, USA); and anti-gamma H2A histone family, member X (γH2AX) from Millipore (Billerica, MA, USA). The membranes were then incubated with the following secondary antibodies at a 1:5,000 dilution: cyclin-B (mouse), β-actin (mouse), cleaved caspase-3 (rabbit), cleaved PARP (rabbit), and γH2AX (mouse). Immunoreactive protein bands were visualized using an enhanced chemiluminescence system (Amersham Biosciences, Piscataway, NJ, USA) and scanned.
Flow cytometry
The cells were cultured before irradiation or treatment with AuNPs. Cells were harvested at designated times before staining with PI (1 µg/mL, Sigma-Aldrich) in accordance with the manufacturer’s instructions. Analysis using the FACScan flow cytometer was performed. At least 10,000 cells were counted for every sample, followed by analysis of the data using the CellQuest software.
Measurement of interleukin-8 (IL-8) concentration
An enzyme-linked immunosorbent assay (ELISA) was used to establish the influence of AuNPs on the degree of IL-8 secretion by hepatocytes. Following 3 d of co-culture, the cells were analyzed using the Human IL-8 ELISA kit (cat. no. 950.050.192; Diaclone SAS, Besançon, France) according to the manufacturer’s recommendations. The ELISA plate was read at 450 nm (Titertek Multiskan PLUS MK II; Thermo Fisher Scientific, Inc., 81 Wyman Street, Waltham, MA, USA) using secreted cell culture medium. IL-8 concentrations were determined by constructing a standard curve utilizing recombinant IL-8. The measurements were performed in triplicate.
Statistical analysis
Statistical significance was determined using Tukey’s method with two-way analysis of variance (ANOVA) statistical test followed by GraphPad Prism 6 software (La Jolla, California, USA). Differences were considered significant if the P value was less than 0.05 or 0.001 (*, P<0.05; **, P<0.01; ***, P<0.001).
Ethical consideration
The study was conducted in accordance with the Declaration of Helsinki (as revised in 2013). All in vitro experiments were approved by the Committee at Daegu Catholic University Medical Center.
Results
Effects of AuNP + radiation combined treatment on the viability of normal hepatocytes and normal human hepatic stellate cell line
To evaluate the effects of AuNPs or radiation on the in vitro sensitivity of normal human liver cells, we used the MTT [3-(4,5-dimethylthiazol-2-yl)-2,5-diphenyltetrazolium bromide] tetrazolium reduction assay (Figure 1A). Normal HH cell was exposed to different dose of AuNPs or radiation at the indicated time points and the results showed little toxicity in these conditions. And next, to prove the AuNPs uptake into cells, HH cells were cultured in complete medium containing AuNPs (5 nm) for 24 h and visualized using TEM. Figure 1B shows the internalization and distribution of AuNPs in HH cells. Hepatocytes and Hepatic Stellate cell line were exposed to radiation alone or with radiation of different doses plus AuNPs. The results of the WST-1 assay for determining the cytotoxic effects are shown in Figure 1C. Irradiation combined with AuNP treatment had no significant effect on cell proliferation in normal hepatocytes and hepatic stellate cell line compared with that of radiation alone.
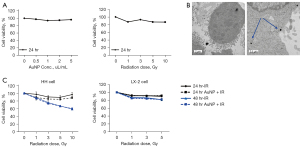
Effects of AuNP + radiation combined treatment on cell survival of normal hepatocytes
To establish the underlying cause for little significant reduction in total cell number using the combination treatment of irradiation and AuNPs, a colony forming assay was performed. Dose-response curves of the normal hepatocyte cell line irradiated in the presence or absence of AuNPs are shown in Figure 2. The results showed that the combination of irradiation at various doses and AuNPs had no significant effect on colony formation in normal hepatocytes compared with that of radiation alone, confirming the lack of cytotoxic effects.
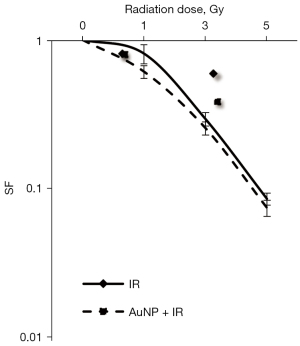
Effects of AuNP + radiation combined treatment on hepatocyte apoptosis
To further explore the apoptotic effect in combination treatment, we investigated whether AuNPs promoted irradiation-induced apoptosis. Combined radiation and AuNP treatment for 48 h did not significantly increase the percentage of early apoptotic cells compared with that of radiation treatment alone (Figure 3A). We next investigated whether the AuNP-enhanced radiation cytotoxicity resulted from increased activation of caspase, resulting in enhanced apoptotic cell death. There was no or only a slight (non-significant) increase in caspase-3 and PARP cleavage in response to combined radiation and AuNP treatment compared with that of AuNP treatment alone (Figure 3B). Sub-G1 cells, which represent apoptotic cells, were only moderately increased by X-ray or AuNP, and hardly increased by the combined treatment (Figure 3C). These results suggest that AuNPs, under the conditions studied here, may not significantly radiosensitize normal hepatocytes.
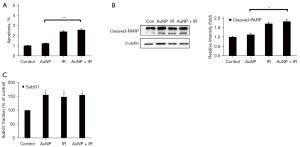
Effects of AuNPs on radiation-induced DNA damage and the cell cycle
As shown in Figure 4A, following exposure to radiation, cell cycle progression was impeded, and the cells in the G2/M phase accumulated a little. However, the combination treatment resulted in no or little change in the proportion of cells in the G2/M phase compared to that of radiation alone. This result was consistent with that of the western blotting of cyclin B, key cell cycle regulators involved in the G2/M transition. To analyze the effect of AuNPs on DNA double-strand break (DSB) processing during radiation treatment, the level of phosphorylated H2AX (γ-H2AX), a DBS marker, was assessed using western blotting. As illustrated in Figure 4B, a slight increase in γ-H2AX expression was observed after 24 h of exposure to radiation and in the presence of AuNPs. To further examine the mechanisms underlying cell viability following AuNP treatment, western blotting was performed on human normal hepatocyte proteins to evaluate changes in cell cycle distribution.
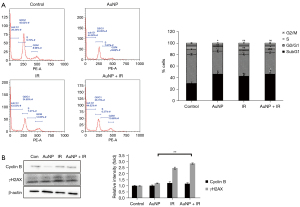
IL-8 expression of X-ray irradiation + AuNP combination treatment of hepatocytes
Radiation or AuNP treatment alone reduced the amount of IL-8 secretion of normal hepatocytes, whereas the combination of the two treatments showed little significant decrease in secretion compared to the control treatment (Figure 5).
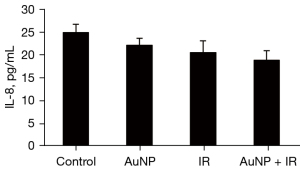
Biological activities of X-ray irradiation + AuNP combination treatment of liver cancer cells
To evaluate the AuNP-induced cytotoxicity for radiation on liver cancer cells, a cell viability assay was performed using Huh7 and HepG2 cells. In the combined treatment group, the cell viability was decreased significantly compared with that of single treatment (Figure 6A). The cell death detection was performed in the examination of early apoptosis for ascertaining the introduction of apoptosis in liver cancer cells with AuNP and radiation collaboratively. The number of cells in apoptosis of liver cancer cells increased more substantially due to 72 hours of AuNP combined radiation therapy compared with that of normal cells (Figure 6B).
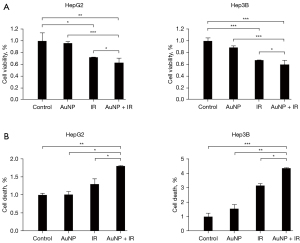
Discussion
Nanoparticles have been widely investigated to selectively amplify the radiation dose in tumors, highlighting an important tool to potentially boost the outcomes of radiation therapy based on both in vitro and in vivo studies (18,19). The high atomic number (Z) of AuNPs indicates their potential to penetrate the tumor vasculature (20). The studies conducted by Zhang et al. and De Jong et al. showed that size-limited nanoparticles travel through the body more efficiently than do large ones (21,22). Additionally, Chen et al. established that the toxicity of the smallest nanoparticles was not as high as that of their larger counterparts (23). In addition, Chithrani et al., Jiang et al., and Trono et al. (24-26) discovered enhanced mid-range size uptake compared with the uptake of smaller sizes. This means that there is a possibility that the smallest AuNPs always move too easily away from the tumor, along with the tissues of the lungs, which could lead to lower toxicity and uptake. This is in line with the findings of Chithrani et al.; a better radiation improvement effect was produced by 50 nm AuNPs than by the smaller ones (24). As such, in the event that more AuNPs enter the tumor, radiation enhancement will likely be recorded.
Using the same treatment protocol applied to tumor cell lines, we exposed normal hepatocytes to AuNPs and/or radiation; combination treatment with AuNPs and radiation had no little significant effect on cell survival in normal hepatocytes. According to Valeriote and Carpentier with formula, we found that the effect of AuNPs and IR neither additive nor synergistic on normal hepatocytes. This was similar to the finding of Zhang et al. who observed that it takes more than this combination to have a distinct effect on cell survival (21). In addition, we found that radiation alone caused an increase slightly in caspase-3 and PARP cleavage indicating apoptosis, whereas these additive effects were not observed in combination with radiation. These results suggest that AuNPs may not be able to radiosensitize normal hepatocytes for the enhancement of irradiation-induced apoptosis. We also did not find an increase in γ-H2AX 24 h after radiation exposure in the presence of AuNPs. This effect was consistent in enhancing apoptosis and cell cycle arrest, and in increasing DNA damage, which our group have observed with radiation on normal cells (27).
To further examine the mechanisms underlying the inhibited viability after treatment with AuNPs alone, flow cytometric analysis of PI-stained cells was carried out in normal human hepatocytes, demonstrating no major effect of AuNPs on apoptosis, but an accumulation of cells in the G2/M phase, which did not occur in combination with radiation. This was in line with the observations of Chen et al. who examined the effects of bovine serum albumin-capped AuNPs on radiotherapy both in vitro and in vivo (23). Radiation or AuNPs alone reduced the amount of IL-8 secretion of normal hepatocytes and, as expected, the combination of both did not significantly decrease secretion.
We previously reported that the point of our study is ultimately the treatment of hepatocarcinoma. We also defined the mechanisms driving the cellular response to high-linear energy transfer (LET) uncharged radiation (neutrons) and low-LET radiation (photons) (28). Therefore, our study provides the basis for the clinical application of AuNPs by showing the combined efficacy of radiation with AuNPs in HCC, and by demonstrating the low toxicity to normal liver cells.
Notably, cells are always found to comprise various organic radicals because of the way their metabolism works. It is for this reason that AuNPs are capable of altering the cell chemistry that affects the processes of radical, even prior to the application of ionizing radiation, which can principally obliterate the resistivity of the cell toward radiation ionization (29). Therefore, our findings were taken into consideration for AuNP’s catalytic impact for healthy and tumor-infested cells. This implies that catalyzing certain REDOX reactions within the cancerous cells results in their low ionization resistivity.
In fact, 5-chlorodeoxycytidine has been shown to be an effective radiosensitizer for therapeutic treatment of neoplastic disease (30). However, the research on AuNPs is still in its infancy and many problems still need to be urgently solved, such as how to reduce biological toxicity while improving biological stability. Similar to our results, the cell killing effect has been reported to be large in HCC cancer cells when combined with AuNPs and radiation (31). However, this treatment did not show significant cytotoxicity in normal HH and human hepatic stellate cells (LX-2) as reported in cancer cells. The controversial findings relating to GNP radiosensitization could arise from variations in the examinations performed in terms of major parameters, including the shape of the GNP, size, cell line type, and concentration, as well as radiation type and energy (31). On the basis of our results, we show that AuNPs in combination with radiation can potentially improve outcomes in the treatment of HCC without high cytotoxicity on especially HH and Human Hepatic stellate cells (LX-2) cell lines, although more research like especially in vivo tests and other several in vitro cell lines, is needed prior to clinical application. In the near future, AuNPs as radiosensitizers may play an important role in the treatment of HCC with reduced biological toxicity.
Acknowledgments
Funding: This study was supported by Bumsuck Academic Research Fund in 2020.
Footnote
Reporting Checklist: The authors have completed the MDAR reporting checklist. Available at https://tcr.amegroups.com/article/view/10.21037/tcr-21-1855/rc
Data Sharing Statement: Available at https://tcr.amegroups.com/article/view/10.21037/tcr-21-1855/dss
Peer Review File: Available at https://tcr.amegroups.com/article/view/10.21037/tcr-21-1855/prf
Conflicts of Interest: All authors have completed the ICMJE uniform disclosure form (available at https://tcr.amegroups.com/article/view/10.21037/tcr-21-1855/coif). The authors have no conflicts of interest to declare.
Ethical Statement: The authors are accountable for all aspects of the work in ensuring that questions related to the accuracy or integrity of any part of the work are appropriately investigated and resolved. The study was conducted in accordance with the Declaration of Helsinki (as revised in 2013). All
Open Access Statement: This is an Open Access article distributed in accordance with the Creative Commons Attribution-NonCommercial-NoDerivs 4.0 International License (CC BY-NC-ND 4.0), which permits the non-commercial replication and distribution of the article with the strict proviso that no changes or edits are made and the original work is properly cited (including links to both the formal publication through the relevant DOI and the license). See: https://creativecommons.org/licenses/by-nc-nd/4.0/.
References
- Babaei M, Ganjalikhani M. The potential effectiveness of nanoparticles as radio sensitizers for radiotherapy. Bioimpacts 2014;4:15-20. [PubMed]
- Su XY, Liu PD, Wu H, et al. Enhancement of radiosensitization by metal-based nanoparticles in cancer radiation therapy. Cancer Biol Med 2014;11:86-91. [PubMed]
- Kim D, Park S, Lee JH, et al. Antibiofouling polymer-coated gold nanoparticles as a contrast agent for in vivo X-ray computed tomography imaging. J Am Chem Soc 2007;129:7661-5. [Crossref] [PubMed]
- Daniel MC, Astruc D. Gold nanoparticles: assembly, supramolecular chemistry, quantum-size-related properties, and applications toward biology, catalysis, and nanotechnology. Chem Rev 2004;104:293-346. [Crossref] [PubMed]
- Zhang P, Qiao Y, Xia J, et al. Enhanced radiation therapy with multilayer microdisks containing radiosensitizing gold nanoparticles. ACS Appl Mater Interfaces 2015;7:4518-24. [Crossref] [PubMed]
- Liu P, Jin H, Guo Z, et al. Silver nanoparticles outperform gold nanoparticles in radiosensitizing U251 cells in vitro and in an intracranial mouse model of glioma. Int J Nanomedicine 2016;11:5003-14. [Crossref] [PubMed]
- Zheng Q, Yang H, Wei J, et al. The role and mechanisms of nanoparticles to enhance radiosensitivity in hepatocellular cell. Biomed Pharmacother 2013;67:569-75. [Crossref] [PubMed]
- Lara-Cruz C, Jiménez-Salazar JE, Arteaga M, et al. Gold nanoparticle uptake is enhanced by estradiol in MCF-7 breast cancer cells. Int J Nanomedicine 2019;14:2705-18. [Crossref] [PubMed]
- Teraoka S, Kakei Y, Akashi M, et al. Gold nanoparticles enhance X-ray irradiation-induced apoptosis in head and neck squamous cell carcinoma in vitro. Biomed Rep 2018;9:415-20. [Crossref] [PubMed]
- Lett JT. Advances in Radiation Biology. Elsevier; 1990.
- Nakajima T, Ninomiya Y, Nenoi M. Radiation-Induced Reactions in The Liver - Modulation of Radiation Effects by Lifestyle-Related Factors. Int J Mol Sci 2018;19:3855. [Crossref] [PubMed]
- Guo M, Sun Y, Zhang XD. Enhanced Radiation Therapy of Gold Nanoparticles in Liver Cancer. Applied Sciences 2017; [Crossref]
- Yao Y, Zang Y, Qu J, et al. The Toxicity of Metallic Nanoparticles on Liver: The Subcellular Damages, Mechanisms, And Outcomes. Int J Nanomedicine 2019;14:8787-804. [Crossref] [PubMed]
- Blouin A, Bolender RP, Weibel ER. Distribution of organelles and membranes between hepatocytes and nonhepatocytes in the rat liver parenchyma. A stereological study. J Cell Biol 1977;72:441-55. [Crossref] [PubMed]
- Do H, Healey JF, Waller EK, et al. Expression of factor VIII by murine liver sinusoidal endothelial cells. J Biol Chem 1999;274:19587-92. [Crossref] [PubMed]
- Hamid AA, Frank MJ, Moore CI. Wave-like dopamine dynamics as a mechanism for spatiotemporal credit assignment. Cell 2021;184:2733-2749.e16. [Crossref] [PubMed]
- Hayakawa M, Sakata A, Hayakawa H, et al. Characterization and visualization of murine coagulation factor VIII-producing cells in vivo. Sci Rep 2021;11:14824. [Crossref] [PubMed]
- Rahman WN, Bishara N, Ackerly T, et al. Enhancement of radiation effects by gold nanoparticles for superficial radiation therapy. Nanomedicine 2009;5:136-42. [Crossref] [PubMed]
- Cooper DR, Bekah D, Nadeau JL. Gold nanoparticles and their alternatives for radiation therapy enhancement. Front Chem 2014;2:86. [Crossref] [PubMed]
- Popovtzer R, Agrawal A, Kotov NA, et al. Targeted gold nanoparticles enable molecular CT imaging of cancer. Nano Lett 2008;8:4593-6. [Crossref] [PubMed]
- Zhang XD, Wu D, Shen X, et al. Size-dependent in vivo toxicity of PEG-coated gold nanoparticles. Int J Nanomedicine 2011;6:2071-81. [Crossref] [PubMed]
- De Jong WH, Hagens WI, Krystek P, et al. Particle size-dependent organ distribution of gold nanoparticles after intravenous administration. Biomaterials 2008;29:1912-9. [Crossref] [PubMed]
- Chen YS, Hung YC, Liau I, et al. Assessment of the In Vivo Toxicity of Gold Nanoparticles. Nanoscale Res Lett 2009;4:858-64. [Crossref] [PubMed]
- Chithrani BD, Ghazani AA, Chan WC. Determining the size and shape dependence of gold nanoparticle uptake into mammalian cells. Nano Lett 2006;6:662-8. [Crossref] [PubMed]
- Jiang W, Kim BY, Rutka JT, et al. Nanoparticle-mediated cellular response is size-dependent. Nat Nanotechnol 2008;3:145-50. [Crossref] [PubMed]
- Trono JD, Mizuno K, Yusa N, et al. Size, concentration and incubation time dependence of gold nanoparticle uptake into pancreas cancer cells and its future application to X-Ray Drug Delivery System. J Radiat Res 2011;52:103-9. [Crossref] [PubMed]
- Kim EH, Kim MS, Cho CK, et al. Low and high linear energy transfer radiation sensitization of HCC cells by metformin. J Radiat Res 2014;55:432-42. [Crossref] [PubMed]
- Kim EH, Kim MS, Song HS, et al. Gold nanoparticles as a potent radiosensitizer in neutron therapy. Oncotarget 2017;8:112390-400. [Crossref] [PubMed]
- Shcherbakov V, Denisov SA, Mostafavi M. Selective Oxidation of Transient Organic Radicals in the Presence of Gold Nanoparticles. Nanomaterials (Basel) 2021;11:727. [Crossref] [PubMed]
- Greer SB. Treatment of neoplastic diseases by radiation. WIPO; 2000.
- Mesbahi A. A review on gold nanoparticles radiosensitization effect in radiation therapy of cancer. Rep Pract Oncol Radiother 2010;15:176-80. [Crossref] [PubMed]