Optimizing radiation for cancer immunotherapy
Various protocols are under investigation for the purpose of optimizing radiotherapy for cancer treatment. Due to recent advances in image guidance and radiation delivery, a new option is to treat tumors with a single intense dose of radiation, 30 Gy or more (1,2). Alternatively, radiation doses can be “fractionated” over multiple treatment periods. Radiotherapy kills tumor cells and their associated stromal and vascular cells, and in some instances can induce T cell-mediated immunity that is effective at killing tumors outside the radiated area, a phenomenon called the “abscopal effect” (3,4). Since complete tumor remission usually depends on an effective anti-tumor immune response, it is important to determine how different radiation regimens influence anti-tumor immune responses. Filatenkov and coworkers (5) investigated the effect of radiation delivery protocols on the numbers and properties of immune cells in the microenvironments of colon cancer cells in mice. Weakly immunogenic ectopic CT26 colon tumors grown in syngeneic Balb/c mice usually responded to a single 30 Gy dose of intense radiation with durable tumor remissions due to T cell-mediated tumor killing. Fractionated radiation regimens were not as effective at stimulating T cells responses or durable remissions. The authors conclude that an examination of the tumor immune response may be useful for optimizing radiation regimens applied to various tumors.
A critical role for myeloid cells in tumor immunosuppression following radiation
Solid tumors produce factors that normally evoke an immune response, but tumors also create an immunosuppressive microenvironment by producing additional anti-inflammatory factors such as adenosine (6), TGFβ (7) and nitric oxide (8). Tumor cells also express indoleamine 2,3-dioxygenase (IDO) that converts L-tryptophan to L-kynurenine (9). Tryptophan depletion and kynurenine accumulation inhibit immune effector cell proliferation. These factors also stimulate the production of immunosuppressive cells such as myeloid-derived suppressor cells (MDSCs), tolerogenic (M2) tumor-associated macrophages (TAMs), and T regulatory (Treg) cells. These suppressor cell populations influence T cells to express additional inhibitory signaling molecules (PD-1, CTLA-4, and Tim-3). Filatenkov and coworkers (5) found that high intensity radiation of tumors, as opposed to fractionated radiation, resulted in tumor remissions that were associated with an increase in tumor-associated CD8+ T cells and a reduction in CD11b+/Gr1+ MDSCs over 14 days following radiation. These responses were dependent on CD8+ dendritic cells (DCs), CD4+/CD40L+ T helper cells, CD8+ cytotoxic T cells and IFNγ (Figure 1). Tumor remission in response to intense radiation was not observed in Batf3−/− mice that lack CD8+ DCs (10). The findings implicate the minor CD8+ DC subset, as important for tumor antigen cross presentation and CD8+ T cell expansion in tumors and/or tumor-draining lymph nodes. They also suggest that radiation, together with T cells activation and IFNγ, cooperate to reduce MDSCs in tumors, to enhance the ratio of CD8+ DCs/MDSCs and thereby produce anti-tumor immunity.
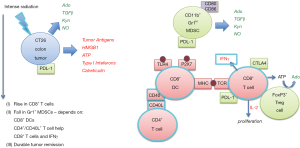
Effects of chemotherapeutic agents on tumor immunity
As with intense radiation, the antitumor activity of some chemotherapeutic agents is mediated in part by activation of host immunity. Gemcitabin (11) and 5-fluouracil (12) are cytotoxic to MDSCs and activate tumor T cells. Cisplatin was found to increase numbers of tumor-associated DCs, decrease MDSCs, and enhance the immune response in melanoma-bearing mice (13). Paclitaxel (14) and docetaxel (15) were also found to reduce numbers of tumor-associated MDSCs. It will be of interest to determine if these responses depend on CD8+ DCs, as is the case with intense radiation.
Effects of adenosine triphosphate (ATP) and adenosine of tumor immunity
ATP released from stressed or apoptotic tumor cells in response to radiation is acutely excitatory to the immune system by activating pro-inflammatory ATP-receptors (P2X and P2Y receptors) found on myeloid and lymphoid cells (16). However, certain tumors and tumor-associated suppressor cells express CD73 or CD39, which are ecto-enzymes that rapidly convert pro-inflammatory ATP into anti-inflammatory adenosine (17). Myeloid-selective deletion of immunosuppressive adenosine A2A receptors (A2AR) was recently found to change the phenotype of MDSCs by greatly reducing their IL-10 production, and to suppress the growth and metastasis of 4T1-12B breast cancer cells (6). A2AR blockers in tumors stimulate the activation of T cells, much like “check point inhibitors” such as anti-PD-1 and anti-CTLA4. In fact, A2AR blockade and anti-PD-1 synergistically inhibit the growth of breast and colon cancer cells (18). Since intense radiation causes tumor cell necrosis and apoptosis, rapidly released ATP can be degraded to adenosine. It will be of interest in future studies to determine if the combination of intense radiation and adenosine receptor blockade robustly stimulates anti-tumor immunity, and the roles for CD8+ DCs and MDSCs in these responses.
Acknowledgments
Funding: None.
Footnote
Provenance: This is a Guest Commentary commissioned by Section Editor Hongcheng Zhu, MD, PhD (Department of Radiation Oncology, The First Affiliated Hospital of Nanjing Medical University, Nanjing, China).
Conflicts of Interest: The author owns equity in Lewis and Clark, Pharmaceuticals, a company developing drugs targeting adenosine receptors.
Open Access Statement: This is an Open Access article distributed in accordance with the Creative Commons Attribution-NonCommercial-NoDerivs 4.0 International License (CC BY-NC-ND 4.0), which permits the non-commercial replication and distribution of the article with the strict proviso that no changes or edits are made and the original work is properly cited (including links to both the formal publication through the relevant DOI and the license). See: https://creativecommons.org/licenses/by-nc-nd/4.0/.
References
- Musunuru HB, Loblaw A. Clinical trials of stereotactic ablative radiotherapy for prostate cancer: updates and future direction. Future Oncol 2015;11:819-31. [Crossref] [PubMed]
- Rekers NH, Troost EG, Zegers CM, et al. Stereotactic ablative body radiotherapy combined with immunotherapy: present status and future perspectives. Cancer Radiother 2014;18:391-5. [Crossref] [PubMed]
- Reynders K, Illidge T, Siva S, et al. The abscopal effect of local radiotherapy: using immunotherapy to make a rare event clinically relevant. Cancer Treat Rev 2015;41:503-10. [Crossref] [PubMed]
- Park SS, Dong H, Liu X, et al. PD-1 Restrains Radiotherapy-Induced Abscopal Effect. Cancer Immunol Res 2015;3:610-9. [Crossref] [PubMed]
- Filatenkov A, Baker J, Mueller AM, et al. Ablative Tumor Radiation Can Change the Tumor Immune Cell Microenvironment to Induce Durable Complete Remissions. Clin Cancer Res 2015;21:3727-39. [Crossref] [PubMed]
- Cekic C, Day YJ, Sag D, et al. Myeloid expression of adenosine A2A receptor suppresses T and NK cell responses in the solid tumor microenvironment. Cancer Res 2014;74:7250-9. [Crossref] [PubMed]
- Shvedova AA, Kisin ER, Yanamala N, et al. MDSC and TGFβ Are Required for Facilitation of Tumor Growth in the Lungs of Mice Exposed to Carbon Nanotubes. Cancer Res 2015;75:1615-23. [Crossref] [PubMed]
- Jayaraman P, Parikh F, Lopez-Rivera E, et al. Tumor-expressed inducible nitric oxide synthase controls induction of functional myeloid-derived suppressor cells through modulation of vascular endothelial growth factor release. J Immunol 2012;188:5365-76. [Crossref] [PubMed]
- Munn DH, Mellor AL. Indoleamine 2,3-dioxygenase and tumor-induced tolerance. J Clin Invest 2007;117:1147-54. [Crossref] [PubMed]
- Hildner K, Edelson BT, Purtha WE, et al. Batf3 deficiency reveals a critical role for CD8alpha+ dendritic cells in cytotoxic T cell immunity. Science 2008;322:1097-100. [Crossref] [PubMed]
- Suzuki E, Kapoor V, Jassar AS, et al. Gemcitabine selectively eliminates splenic Gr-1+/CD11b+ myeloid suppressor cells in tumor-bearing animals and enhances antitumor immune activity. Clin Cancer Res 2005;11:6713-21. [Crossref] [PubMed]
- Vincent J, Mignot G, Chalmin F, et al. 5-Fluorouracil selectively kills tumor-associated myeloid-derived suppressor cells resulting in enhanced T cell-dependent antitumor immunity. Cancer Res 2010;70:3052-61. [Crossref] [PubMed]
- Chen J, Huang X, Huang G, et al. Preconditioning chemotherapy with cisplatin enhances the antitumor activity of cytokine-induced killer cells in a murine melanoma model. Cancer Biother Radiopharm 2012;27:210-20. [Crossref] [PubMed]
- Naiditch H, Shurin MR, Shurin GV. Targeting myeloid regulatory cells in cancer by chemotherapeutic agents. Immunol Res 2011;50:276-85. [Crossref] [PubMed]
- Kodumudi KN, Woan K, Gilvary DL, et al. A novel chemoimmunomodulating property of docetaxel: suppression of myeloid-derived suppressor cells in tumor bearers. Clin Cancer Res 2010;16:4583-94. [Crossref] [PubMed]
- Aymeric L, Apetoh L, Ghiringhelli F, et al. Tumor cell death and ATP release prime dendritic cells and efficient anticancer immunity. Cancer Res 2010;70:855-8. [Crossref] [PubMed]
- Kumar V. Adenosine as an endogenous immunoregulator in cancer pathogenesis: where to go? Purinergic Signal 2013;9:145-65. [Crossref] [PubMed]
- Beavis PA, Milenkovski N, Henderson MA, et al. Adenosine Receptor 2A Blockade Increases the Efficacy of Anti-PD-1 through Enhanced Antitumor T-cell Responses. Cancer Immunol Res 2015;3:506-17. [Crossref] [PubMed]