Identification of ACSL4 as a biomarker and contributor of ferroptosis in clear cell renal cell carcinoma
Introduction
Renal cell carcinoma (RCC), which is among the most prevalent malignancy affecting the urinary tract, is attributed to 2–3 percent of all cancers that occur in adults. Clear cell renal cell carcinoma (ccRCC), which is the most prevalent type of RCC, is typically characterized by an elevated metastatic risk and unfavorable responsiveness to radiation and chemotherapy (1,2). It is important to highlight that 30% of individuals with ccRCC experienced localized advancement and distant metastases when they are being diagnostically identified, and this is the major cause of the increased fatality rates for these patients (3). Consequently, it is beneficial to create diagnosis and therapy targets by identifying genes that have varying levels of expression in ccRCC.
Acyl-CoA synthetase long-chain family member 4 (ACSL4), which is often referred to as FACL4, was found in the year 2002 as the mutant gene attributed to the non-specific X-linked mental retardation (4). According to the findings of Maloberti and his colleagues, ACSL4 has a substrate selectivity for arachidonic acid (AA) as well as eicosapentaenoic acid (EPA). Even more intriguing is the observation that the levels of free AA may, in turn, influence the levels of the ACSL4 protein inside the cells (5). To this point, it has been demonstrated that dysregulation of ACSL4 is associated with a significant variety of disorders, some of which include diabetes (6), acute kidney injury (7), and malignant tumors (8).
Cancer cells often exhibit either innate or acquired resistance to the programmed cell death process known as apoptosis. As a consequence, the generation of non-apoptotic types of controlled cell death has emerged as a promising therapeutic technique for treating cancers (9). The newly identified, non-apoptotic, controlled cell death known as ferroptosis was found for the first time in cancerous cells with an oncogenic Ras mutation (10). In recent research, it was shown that silencing ACSL4 prevented erastin from inducing ferroptosis in HL60 and HepG2 cells, and that overexpressing ACSL4 reverted K562 and LNCaP cells back to their previous levels of sensitivity to the ferroptosis mediator erastin (11). The lowered ACSL4 expression level, which anticipates a cell line’s susceptibility to ferroptosis, may be found in a variety of RCC cell lines (12). As a result, the levels of ACSL4 expression could be connected to the advancement of ccRCC because of the role it plays in inducing ferroptosis. Nonetheless, neither the expression patterns nor the functional relevance of ACSL4 in ccRCC has been investigated to this point. In light of this, the current work evaluated the expression ACSL4 in ccRCC as well as its correlations with patients' clinical and pathological variables and prognoses. Additionally, the impact of ACSL4 on ferroptosis was explored by undertaking experimental and bioinformatics studies. I present the following article in accordance with the REMARK reporting checklist (available at https://tcr.amegroups.com/article/view/10.21037/tcr-21-2157/rc).
Methods
Analysis of ACSL4 using bioinformatics
To determine the expression levels of ACSL4 mRNA in ccRCC and corresponding normal samples, we employed the Oncomine database (https://www.oncomine.org) (13), including Beroukhim Renal dataset, Lenburg renal datasets, Gumz Renal and Jones Renal datasets. Subsequently, we screened The Cancer Genome Atlas (TCGA) KIRC database to determine the link between ACSL4 mRNA expression and ccRCC patients’ prognoses from UALCAN (http://ualcan.path.uab.edu) (14). In The Human Protein Atlas (HPA) (https://www.proteinatlas.org) (15), immunohistochemical imaging (Antibody HPA005552) was utilized to undertake a comparative assessment of the expression levels of ACSL4 protein found in human ccRCC and normal samples. Utilizing a web-based database named Kaplan-Meier (KM) Plotter (https://kmplot.com), we investigated the potential prognostic significance of overexpressed ACSL4 (16). The KM Plotter dataset contains an analysis of tumor mutation burden (TMB), which is described as the actual number of indel mutations per million bases, base substitutions, and somatic coding errors. This analysis provides an accurate assessment of both the total neoantigen load as well as the mutational load. All samples were divided into high and low tumor mutation burden groups with median value as the cutoff point. cBioPortal (http://www.cbioportal.org) was utilized to conduct a study of ACSL4 gene expression based on the TCGA-KIRC dataset (17). Functional analyses of ACSL4 were conducted by Gene Ontology (GO) and Kyoto Encyclopedia of Genes and Genomes (KEGG) in the Database for Annotation, Visualization, and Integrated Discovery (DAVID) (https://david.ncifcrf.gov/summary.jsp) (18). The ccA and ccB molecular subtypes of RCC are defined as previous research reported (2).
Specimens of human ccRCC tissue
In total, this research comprised 16 participants who received ccRCC diagnoses at the cancer center of Sun Yat-sen University between the years 2018 and 2019. The cancer samples of these patients, together with 5 cm of adjoining non-tumor tissues, were taken for further qPCR testing. Prior to undergoing a surgical operation, these patients did not receive any adjuvant treatment, including radiation, chemotherapy, or any other kind of treatment. The study was conducted in accordance with the Declaration of Helsinki (as revised in 2013). This study was approved by the Academic Committee of Sun Yat-sen University Cancer Center (No. B2018-006-01), and informed consent was taken from all the patients.
Cell culture
The American Type Culture Collection (ATCC, Manassas, VA, USA) supplied all four of the ccRCC cell lines that were used in this study: RCC4, A498, 769-P, and 786-O. We cultured the cells in a humid chamber at 37 ℃ comprising 5% carbon dioxide utilizing a DMEM high glucose medium supplemented with 10% fetal bovine serum (FBS) and 100 U/mL of streptomycin/penicillin.
Cell proliferation
The Cell Counting Kit-8 (CCK-8) experiment was undertaken to ascertain the proliferative capacity of cells in compliance with the specifications provided by the manufacturer.
Western blotting assays
Lysis of the samples or cells was achieved utilizing RIPA buffer, and the BCA kit (Beyotime Institute of Biotechnology, Shanghai, China) was adopted to quantify the protein concentration. SDS-PAGE was performed on 30 µg total proteins derived from the cells or tissues, after which they were deposited into a PVDF membrane (Millipore, Bedford, MA, USA). After being blocked with skim milk at a concentration of 5% for one hour at ambient temperature, the PVDF membranes were subjected to overnight incubation in a primary antibody at 4 ℃. The next step involved rinsing the membranes thrice using PBST and subsequently incubating them for 2 h at ambient temperature with a secondary antibody. Eventually, visualization of the signals was done with exposure to X-ray films and super or enhanced chemiluminescence (Pierce, Rockford, IL, USA).
Quantitative real-time polymerase chain reaction (qPCR) analysis
Synthesis of first-strand cDNA was accomplished with the aid of the Reverse Transcription System Kit (OriGene Technologies) following the guidelines stipulated by the manufacturer. cDNA obtained from the cell specimens was amplified utilizing appropriate primers (ACSL4: 5'-AGGTGCTCCAACTCTGCCAGTA-3' and 5'-GCTATCTCCTCAGACACACCGA-3') and the results were standardized with respect to actin RNA (5'-AGGTCTTTGCGGATGTCCACGT-3' and 5'-CACCATTGGCAATGAGCGGTTC-3').
RNAi and gene transfection
Sigma Aldrich (St. Louis, MO, USA) was the supplier for the human ACSL4-shRNA. OriGene Technologies supplied the human ACSL4-cDNA. The Lipofectamine™ 3000 or Lentivirus Transduction System was utilized to carry out the transfections in line with the specifications provided by the manufacturer.
Iron assay
The level of ferrous iron (Fe2+) contained inside cells or within mitochondria was measured with an iron assay kit obtained from Sigma Aldrich following the requirements stipulated by the manufacturer.
Lipid ROS assays
After being treated in the manner specified, the cells were subjected to trypsinization and resuspension in a medium that contained 10% FBS to perform a lipid ROS assay. After that, 10 µM of C11-BODIPY (Thermo Fisher, Waltham, MA, USA) was introduced into the specimens, which were subsequently incubated for 30 minutes at 37 ℃ with 5% carbon dioxide while being shielded from light. To get rid of any residual C11-BODIPY, the cells were rinsed twice in PBS. By utilizing a flow cytometer, we assessed the fluorescence of C11-BODIPY (581/591) by simultaneously collecting red (581/610 nm) and green (484/510 nm) signals at different wavelengths.
Statistical analysis
GraphPad Prism 6.0 (GraphPad Software, San Diego, CA, USA) and SPSS 22.0 (IBM SPSS, Chicago, IL, USA) were utilized to execute all analyses of statistical data. The results from the statistical analysis were presented as means ± SD. The two-tailed Student’s t-test and the ANOVA LSD test were implemented to assess whether there was a significant difference across the subgroups. The threshold for statistical significance was determined to be P value <0.05.
Results
Expression of ACSL4 is down-modulated in ccRCC
Premised on the analysis of the Oncomine database, we discovered that the level of ACSL4 mRNA expression was considerably lower in ccRCC in contrast with the normal samples (Figure 1). The use of qPCR allowed for the detection of ACSL4 expression in postoperative ccRCC tumor samples relative to normal kidney samples. When tumor tissues (n=16) were subjected to a comparison with their matched normal tissues (n=16), the relative ACSL4 expression level was found to be considerably lower in the former (P<0.001, Figure 2A). Following that, we examined the HPA database to find evidence of the ACSL4 protein expression. It was shown that ACSL4 IHC is expressed partly in some tubules in normal kidney with moderate to high staining (Figure 2B). The percentage of samples with positive ACSL4 expression was 72.7 percent (8/11 samples). In contrast, nearly no or extremely weak ACSL4 staining was found in the majority of tumor samples, and staining was mostly distributed on the cytoplasm and the cell membrane (Figure 2C,2D).
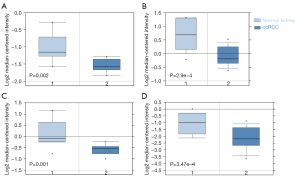
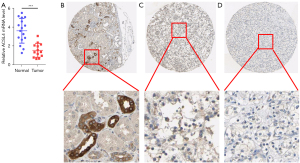
Decreased ACSL4 mRNA expression is linked to malignant clinical-pathological characteristics in ccRCC patients
We additionally evaluated the association of ACSL4 mRNA expression with clinical and pathological characteristics based on the UALCAN data set. These results showed the level of ACSL4 mRNA was considerably lowered in the ccRCC group in contrast with the normal group (Figure 3A, P<0.001), despite cancer stages, tumor grade, nodal invasion, ccRCC subtypes, or gender (Figure 3B-3F). By employing the survival data retrieved from the KM plotter, we plotted KM curves of RFS (relapse-free survival) and OS (overall survival) based on data from 530 patients (including 195 ACSL4 low expression patients and 335 ACSL4 high expression cases) to examine the relationship of mRNA expression of ACSL4 with ccRCC patients’ prognoses. According to the findings, patients who had upregulated ACSL4 exhibited remarkably longer OS time (P=0.014; Figure 4A). In terms of RFS, higher expression of ACSL4 was also related to better survival, but it is not statistically significant (P=0.29; Figure 4B). Additional subgroup analyses illustrated favorable OS with higher ACSL4 expression for female patients (P=0.013; Figure 4C), those with a more advanced grade and stage of the tumor (P=0.046 and P=0.13, correspondingly; Figure 4D,4E), and those with TMB (P=0.013; Figure 4F).

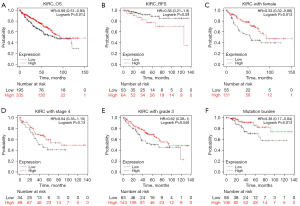
ACSL4 upregulation enhances ferroptosis
To verify our hypotheses that the ACSL4 dysregulation was associated with ferroptosis in ccRCC, we measured ACSL4 expression in 4 renal cancer cells. RCC4 and A498 cells expressed relatively elevated levels of ACSL4, in contrast with 769-P and 786-O cells, which expressed extremely low levels (Figure 5A). Next, we explored whether or not the ACSL4 expression is responsible for erastin's anticancer effect (a ferroptosis inducer). The transfection of ACSL4 cDNA into 769-P and 786-O cells resulted in the upregulation of the mRNA levels of ACSL4 (Figure 5B). Upregulation of ACSL4 expression mediated by gene transfection greatly enhanced the susceptibility of 769-P and 786-O cells to the erastin-mediated cell death (Figure 5C,5D), providing further evidence for the hypothesis that ACSL4 functions as a positive modulator of ferroptosis. Both lipid peroxidation and the buildup of ferrous ion (Fe2+) are necessary steps in the process of ferroptosis induction. As a consequence, we investigated whether or not ACSL4 affected these events during ferroptosis. Notably, erastin-mediated lipid ROS generation was considerably enhanced by the upregulation of ACSL4 (Figure 5E). Conversely, erastin-mediated Fe2+ levels were not influenced by the ACSL4 upregulation (Figure 5F), indicating that ACSL4 modulates the cellular lipid composition rather than iron accumulation. The results above showed that ACSL4 leads to a decrease in cell number and overload of lipid peroxidation, all of which may partially result in ferroptosis of ccRCC cells.
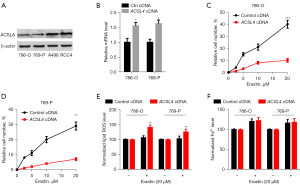
ACSL4 knockdown suppresses ferroptosis
To additionally validate the function of ACSL4 in ferroptosis, we transfected a selective shRNA targeting ACSL4 into RCC4 and A498 cell lines, which resulted in a considerable reduction in the levels of ACSL4 mRNA (Figure 6A). The observation that inhibiting ACSL4 expression substantially attenuated cell death caused by erastin (Figure 6B,6C) revealed that ACSL4 performs an important part in the ferroptosis process. In addition, we examined the degree of iron buildup and lipid peroxidation in RCC4 and A498 cells once the expression of ACSL4 was silenced using shRNA. After treatment with erastin, the level of lipid ROS was greatly reduced (Figure 6D), but there was no change in the number of accumulated Fe2+ (Figure 6E) in RCC4 and A498 cells. We concluded that ACSL4 might partly participate in ferroptosis by enhancing erastin-mediated lipid peroxidation, mainly because lipid peroxidation is both a defining characteristic of ferroptosis and an inducer of the process.
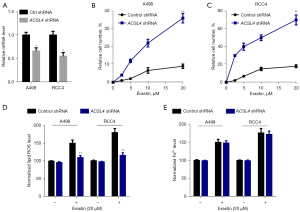
GO functional annotation and pathway enrichment of ACSL4
An investigation into the possible mechanisms of ACSL4’s involvement in the malignant bioactivity of KIRC was carried out by conducting a TCGA-KIRC Gene co-expression network analysis. The cBioPortal of Cancer Genomics was employed in the TCGA-KIRC dataset to determine the highly co-expressed genes with ACSL4. A criterion of absolute Spearman’s r ≥0.5 was used to identify 295 genes shown to be co-expressed with ACSL4 (website: https://cdn.amegroups.cn/static/public/tcr-21-2157-01.pdf). After that, these genes were entered into DAVID so that additional GO analysis and KEGG pathway analysis could be carried out. Figure 7 contains a list of the ten most important terms that were uncovered by the GO and the KEGG analysis. The ubiquitin-mediated proteolysis pathway was shown to be the most substantially enriched for genes co-expressed with ACSL4 (Figure 7A). In the biological process and molecular function ontology, protein ubiquitination (GO: 0016567) and ubiquitin-protein transferase activity (GO: 0004842) were the primary enriched GO categories (Figure 7B,7C). In the cellular component ontology, the endocytic vesicle (GO: 0030139) is the top category in the pathophysiological process (Figure 7D).
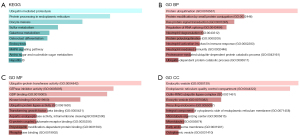
Discussion
Ferroptosis was not discovered until 2012 when Dixon et al. published their findings on a study they had conducted on a small molecule known as erastin (10). The process of ferroptosis is a novel kind of cell death that is reliant on iron and is linked to oxidative stress (10). Ferroptosis is often hallmarked by an accumulation of cytoplasmic and lipid ROS, a decrease in the volume of the mitochondria, an enhancement in the density of the mitochondrial membrane, the perforation or deletion of mitochondrial cristae, as well as the disintegration of the outer membrane of the mitochondria (19). Recently, it was established that ACSL4 serves as an important factor of ferroptosis by gene filtering utilizing Genome-Wide CRISPR as well as microarray assessment of anti-ferroptosis cell lines (20). In the current investigation, the expression profiles of ACSL4 along with the clinical-pathological characteristics that are linked to ccRCC were analyzed. Furthermore, indicators of ferroptosis such as lipid reactive oxygen species (ROS) and ferric ion (Fe2+) were investigated upon ACSL4 was dysregulation. As a result, we deduced that the level of ACSL4 in ccRCC is lower than that in normal tissues, and hypothesized that a lower level of ferroptosis would be linked to the onset and advancement of ccRCC.
According to several research reports, ferroptosis has a very strong correlation with a variety of human disorders (6,7,19). There have only been a few studies done on ferroptosis in relation to ccRCC. Miess et al. proposed that decreased metabolism of fatty acids as a result of restriction of beta-oxidation makes renal cancerous cells extremely reliant on the GSH/GPX pathway to avoid ferroptosis and lipid peroxidation (21). ACSL4, in addition to its roles as an important indicator and modulator of ferroptosis, performs an integral function in determining the degree to which ferroptosis is sensitive by altering the cellular lipid content (11). ACSL4 is responsible for the enrichment of the membrane with the oxidation-prone ω-6 PUFA AA. ACSL4 is associated and aberrantly regulated in various cancers (4). Recently, the researchers found the level of ACSL4 expression in RCC cells decreased, whereas raised ACSL4 levels could promote ferroptosis in RCC cells (12). Consistent with the findings from other studies, we discovered that the expression of ACSL4 was down-modulated in tumors and that this was linked to an unfavorable prognosis, implying that ACSL4 might represent a valuable biological marker and a possible treatment target for ccRCC. However, according to the findings of the research conducted by Peng Lee, ectopic ACSL4 expression in ACSL4-negative prostate cancer (PCa) cells contributed to an enhanced capacity of cells to proliferate, migrate, and invade. On the other hand, deletion of ACSL4 in PCa cells that express endogenous ACSL4 results in attenuation in proliferative, migratory, and invasive capacities of cells. They also found that ACSL4 is responsible for the upregulation of many different pathway proteins, particularly β-catenin LSD1, and p-AKT (22).
Ferroptosis, like other types of cell death, is intimately linked to particular signaling pathways. The presence of ferroptosis is closely linked to iron buildup and lipid peroxidation as the two most important causal factors (10). An ACSL4 upregulation plasmid or a silencing plasmid against ACSL4 was transfected into 4 distinct ccRCC cell lines to confirm the involvement of ACSL4 in ferroptosis in ccRCC. According to the findings, blocking ACSL4 may protect cells from death mediated by erastin as well as the generation of lipid ROS. However, the expression of ACSL4 did not have any effect on the buildup of Fe2+ that was induced by erastin. ACSL4, which functions as an enzyme that activates fatty acids, preferentially works with long-chain polyunsaturated fatty acids as its substrates. ACSL4 is responsible for catalyzing the synthesis of these fatty acids as well as the associated coenzyme A (11), thus influencing the process of lipid peroxidation.
Besides investigating the interaction that occurs between ferroptosis and other kinds of cell death, it is pertinent to examine the functionality and assess the molecular and pathway components connected to ferroptosis. Some recently discovered proteins, including metallothionein-1G, NCOA4, and PEBP1 have been linked to ferroptosis via the processes of iron metabolism and lipid peroxidation (23). By applying GO and KEGG, we confirmed that the gene that is co-expressed with ACSL4 is remarkably enriched in the pathway that is linked to protein ubiquitination. According to the findings of many research reports, the process of ubiquitination has a role in the modulation of the ferroptosis pathway (24). Recent research has shown that the protein BAP1 reduces the degree of histone 2A ubiquitination affinity to the promoters of the ferroptosis suppressor gene SLC7A11, which in turn suppresses the expression of SLC7A11 in a deubiquitinating-dependent way (25). Additionally, BAP1 prevents the absorption of cystine by suppressing the expression of SLC7A11. This results in an increase in lipid peroxidation as well as ferroptosis. The current work demonstrates that similar to SLC7A11 ubiquitination, the ubiquitination of ACSL4 could potentially be engaged in the ferroptosis process, which calls for more investigation on the topic.
Additional research on ferroptosis is required not only to shed light on the molecular basis behind the process but also to open the door to the possibility of developing innovative therapeutic approaches. Sorafenib resistance, for instance, has been found to occur in the treatment of metastatic hepatocellular carcinoma as a consequence of the metallothionein-1G induced suppression of ferroptosis (26). Doll et al. additionally showed that pharmacologic targeting of ACSL4 using the antidiabetic drug family known as thiazolidinediones reduces the degree of tissue death found in a mouse ferroptosis model, indicating that ACSL4 suppression is a feasible therapeutic strategy for preventing ferroptosis-associated illnesses (20).
Noteworthy shortcomings of our work include the absence of a considerable number of previously analyzed tumor and normal specimens from the database, heterogeneous data, and platform heterogeneity. Furthermore, the majority of the community ethics data in the TCGA database is limited to white and black communities, making it difficult to apply these results to other ethnicities. Prospective research concentrating on the mechanisms of ACSL4 as predicted by bioinformatics, both in vivo and in vitro, is warranted to make progress in the field.
Conclusions
Our study demonstrated that ACSL4 is down-regulated in ccRCC and correlated with patients’ clinicopathological features. ACSL4 suppresses proliferation of ccRCC cells, at least in part, by activating ferroptosis. These results indicate that ACSL4 may be regarded as a potential therapeutic target for the treatment of ccRCC and underlie future studies on pathways involving ACSL4 in a clinical setting.
Acknowledgments
The author thanks Bullet Edits Limited for the linguistic editing and proofreading of the manuscript.
Funding: This study was supported by Guangdong Esophageal Cancer Institute Science and Technology Program (No. Q-201706).
Footnote
Reporting Checklist: The author has completed the REMARK reporting checklist. Available at https://tcr.amegroups.com/article/view/10.21037/tcr-21-2157/rc
Conflicts of Interest: The author has completed the ICMJE uniform disclosure form (available at https://tcr.amegroups.com/article/view/10.21037/tcr-21-2157/coif). The author has no conflicts of interest to declare.
Ethical Statement: The author is accountable for all aspects of the work in ensuring that questions related to the accuracy or integrity of any part of the work are appropriately investigated and resolved. The study was conducted in accordance with the Declaration of Helsinki (as revised in 2013). The study was approved by Academic Committee of Sun Yat-sen University Cancer Center (No. B2018-006-01) and informed consent was taken from all the patients.
Open Access Statement: This is an Open Access article distributed in accordance with the Creative Commons Attribution-NonCommercial-NoDerivs 4.0 International License (CC BY-NC-ND 4.0), which permits the non-commercial replication and distribution of the article with the strict proviso that no changes or edits are made and the original work is properly cited (including links to both the formal publication through the relevant DOI and the license). See: https://creativecommons.org/licenses/by-nc-nd/4.0/.
References
- Hsieh JJ, Purdue MP, Signoretti S, et al. Renal cell carcinoma. Nat Rev Dis Primers 2017;3:17009. [Crossref] [PubMed]
- Brannon AR, Reddy A, Seiler M, et al. Molecular Stratification of Clear Cell Renal Cell Carcinoma by Consensus Clustering Reveals Distinct Subtypes and Survival Patterns. Genes Cancer 2010;1:152-63. [Crossref] [PubMed]
- Farber NJ, Kim CJ, Modi PK, et al. Renal cell carcinoma: the search for a reliable biomarker. Transl Cancer Res 2017;6:620-32. [Crossref] [PubMed]
- Kuwata H, Hara S. Role of acyl-CoA synthetase ACSL4 in arachidonic acid metabolism. Prostaglandins Other Lipid Mediat 2019;144:106363. [Crossref] [PubMed]
- Orlando UD, Castillo AF, Dattilo MA, et al. Acyl-CoA synthetase-4, a new regulator of mTOR and a potential therapeutic target for enhanced estrogen receptor function in receptor-positive and -negative breast cancer. Oncotarget 2015;6:42632-50. [Crossref] [PubMed]
- Sha W, Hu F, Xi Y, et al. Mechanism of Ferroptosis and Its Role in Type 2 Diabetes Mellitus. J Diabetes Res 2021;2021:9999612. [Crossref] [PubMed]
- Wang Y, Zhang M, Bi R, et al. ACSL4 deficiency confers protection against ferroptosis-mediated acute kidney injury. Redox Biol 2022;51:102262. [Crossref] [PubMed]
- Sha R, Xu Y, Yuan C, et al. Predictive and prognostic impact of ferroptosis-related genes ACSL4 and GPX4 on breast cancer treated with neoadjuvant chemotherapy. EBioMedicine 2021;71:103560. [Crossref] [PubMed]
- Mou Y, Wang J, Wu J, et al. Ferroptosis, a new form of cell death: opportunities and challenges in cancer. J Hematol Oncol 2019;12:34. [Crossref] [PubMed]
- Dixon SJ, Lemberg KM, Lamprecht MR, et al. Ferroptosis: an iron-dependent form of nonapoptotic cell death. Cell 2012;149:1060-72. [Crossref] [PubMed]
- Yuan H, Li X, Zhang X, et al. Identification of ACSL4 as a biomarker and contributor of ferroptosis. Biochem Biophys Res Commun 2016;478:1338-43. [Crossref] [PubMed]
- Du Y, Zhao HC, Zhu HC, et al. Ferroptosis is involved in the anti-tumor effect of lycorine in renal cell carcinoma cells. Oncol Lett 2021;22:781. [Crossref] [PubMed]
- Rhodes DR, Kalyana-Sundaram S, Mahavisno V, et al. Oncomine 3.0: genes, pathways, and networks in a collection of 18,000 cancer gene expression profiles. Neoplasia 2007;9:166-80. [Crossref] [PubMed]
- Chandrashekar DS, Bashel B, Balasubramanya SAH, et al. UALCAN: A Portal for Facilitating Tumor Subgroup Gene Expression and Survival Analyses. Neoplasia 2017;19:649-58. [Crossref] [PubMed]
- Uhlen M, Zhang C, Lee S, et al. A pathology atlas of the human cancer transcriptome. Science 2017;357:eaan2507. [Crossref] [PubMed]
- Nagy Á, Munkácsy G, Győrffy B. Pancancer survival analysis of cancer hallmark genes. Sci Rep 2021;11:6047. [Crossref] [PubMed]
- Gao J, Aksoy BA, Dogrusoz U, et al. Integrative analysis of complex cancer genomics and clinical profiles using the cBioPortal. Sci Signal 2013;6:pl1. [Crossref] [PubMed]
- Huang da W. Sherman BT, Lempicki RA. Systematic and integrative analysis of large gene lists using DAVID bioinformatics resources. Nat Protoc 2009;4:44-57. [Crossref] [PubMed]
- Peng J, Hao Y, Rao B, et al. A ferroptosis-related lncRNA signature predicts prognosis in ovarian cancer patients. Transl Cancer Res 2021;10:4802-16. [Crossref] [PubMed]
- Doll S, Proneth B, Tyurina YY, et al. ACSL4 dictates ferroptosis sensitivity by shaping cellular lipid composition. Nat Chem Biol 2017;13:91-8. [Crossref] [PubMed]
- Miess H, Dankworth B, Gouw AM, et al. The glutathione redox system is essential to prevent ferroptosis caused by impaired lipid metabolism in clear cell renal cell carcinoma. Oncogene 2018;37:5435-50. [Crossref] [PubMed]
- Wu X, Deng F, Li Y, et al. ACSL4 promotes prostate cancer growth, invasion and hormonal resistance. Oncotarget 2015;6:44849-63. [Crossref] [PubMed]
- Stockwell BR, Friedmann Angeli JP, Bayir H, et al. Ferroptosis: A Regulated Cell Death Nexus Linking Metabolism, Redox Biology, and Disease. Cell 2017;171:273-85. [Crossref] [PubMed]
- Wang X, Wang Y, Li Z, et al. Regulation of Ferroptosis Pathway by Ubiquitination. Front Cell Dev Biol 2021;9:699304. [Crossref] [PubMed]
- Zhang Y, Shi J, Liu X, et al. BAP1 links metabolic regulation of ferroptosis to tumour suppression. Nat Cell Biol 2018;20:1181-92. [Crossref] [PubMed]
- Chen X, Kang R, Kroemer G, et al. Broadening horizons: the role of ferroptosis in cancer. Nat Rev Clin Oncol 2021;18:280-96. [Crossref] [PubMed]