Hypoxia upregulates the expression of lncRNA H19 in non-small cell lung cancer cells and induces drug resistance
Introduction
Lung cancer has the highest cancer-related mortality rate among all cancers globally (1). Non-small cell lung cancer (NSCLC) accounts for 80–85% of lung cancers. However, most patients are at an advanced stage at diagnosis and are not eligible for surgery (2). For these patients, treatment is difficult and the prognosis is poor. For patients with positive expression of specific lung cancer driver genes, some precise targeted therapies can be employed. However, patients without driver genes are required to undergo chemotherapy (3); these patients frequently exhibit drug resistance and their mortality rate is high. At present, the mechanism underlying tumor drug resistance is unknown.
Numerous studies on the mechanism of multi-tumor drug resistance have focused on the tumor cells (4). However, the initiation, development, and progression of tumors, as well as treatment response, are closely related to the tumor microenvironment (5,6). Tumor cells proliferate rapidly and use up oxygen, creating a hypoxic microenvironment in tumor tissues. Hypoxia induces the secretion of a variety of cytokines and promotes changes in cellular signaling pathways, which has a significant impact on tumor development. Studies (7,8) have shown that stimulates the proliferation of tumors and induces the invasion and migration of tumor cells by affecting the expression of various genes in tumor cells. It also enables tumor cells to resist radiotherapy and leads to tumor recurrence and drug resistance (9,10). The hypoxia-inducible factor (HIF) transcription factor is the main regulator of the hypoxic responses in cells and a major focus of research on the hypoxic tumor microenvironment (11,12). HIF activates multiple apoptosis and cell proliferation signaling pathways, inducing the expressions of proteins associated with chemoradiotherapy- and hypoxic-injury resistance and thereby enabling tumor cell survival (13). HIF1-α is the major subunit involved in HIF activity, and inhibiting HIF1-α represents a potentially important strategy for cancer treatment (14,15).
Long non-coding ribonucleic acid (lncRNA) refers to a diverse class of long ribonucleic acid (RNA) transcripts (>200 nucleotides) that are non-protein encoding. LncRNAs are involved in regulating gene expression at multiple levels, including gene chromatin modification, transcription, and processing, with functions in various biological processes. In addition, studies have shown that lncRNAs play an important role in the occurrence and development of a variety of cancers (16). LncRNA H19, which is encoded by a gene located on human chromosome 11, is involved in the regulation of embryonic development (17). LncRNA H19 has been shown to promote cancer cell proliferation and metastasis in solid tumors, including lung, gastric, bladder, and ovarian cancers. Studies have also demonstrated lncRNA H19 involvement in the generation of tumor drug resistance (18-20). Recent studies have shown that in some tumors, hypoxic conditions can promote the expression of LncRNA H19 (21,22), which suggests that hypoxia may have a potential relationship with LncRNA H19 in the occurrence and development of malignant tumors. A study (23) has shown that H19 has a role in promoting tumor growth in lung cancer, but there is no study between H19 and lung cancer cells under hypoxic conditions.
Objectives
The objective of this study was to examine whether hypoxia influences lncRNA H19 expression in NSCLC and induces drug resistance. We evaluated the expressions of HIF1-α, lncRNA H19, P-glycoprotein (P-gp), heme oxygenase-1 (HO-1), and multi-drug resistant-associated protein 1 (MRP1) in tumor cells under normoxic and hypoxic conditions. In addition, we investigated the effects of hypoxia on the invasion and migration of tumor cells as well as cisplatin-induced apoptosis. We present the following article in accordance with the MDAR reporting checklist (available at https://tcr.amegroups.com/article/view/10.21037/tcr-22-1812/rc).
Methods
Cell culture
The human NSCLC cell lines, A549 (RRID: CVCL_0023) and H1650 (RRID: CVCL_1483), were purchased from the Cell Bank of Shanghai Institute for Biological Sciences, National Infrastructure of Cell Line Resource. The cells were cultured in a humidified incubator (Forma 4111; Thermo Fisher Scientific, USA) at 37 ℃ in Dulbecco’s modified Eagle’s medium with 4.5 g/L glucose (Cat No 15-017-CVR, Corning, No. 200 Qinjiang Road, Xuhui District, Shanghai) supplemented with 10% fetal bovine serum (FBS) and 1% penicillin-streptomycin.
We used cobaltous chloride (CoCl2) 200 µmol/L to create hypoxic culture environments for the A549 and H1650 cells cultured alone and co-cultured with MSCs (2% oxygen, 5% carbon dioxide, 93% nitrogen).
MTT cell proliferation assay
The cells were plated at 1,000–10,000 cells/well and cultured until they reached confluency. Cisplatin was added to each well and the cells were incubated for 24 hours, the concentration were given successively 10, 20, 40, 80 µmol/L. Thiazolyl blue tetrazolium bromide (MTT) (20 µL; 5 mg/mL) was then added to each well and the cells were incubated for an additional 4 h. The culture medium was removed, and 150 µL of dimethyl sulfoxide (DMSO) was added to each well. Absorbance values (490 nm) were obtained from each well using an enzyme-linked immunosorbent assay kit (HBS-1101, Nanjing Detie, Building A7, No. 9 Kechuang Avenue, Zhongshan Science and Technology Park, Jiangbei New District, Nanjing, China).
RNA extraction
RNA was extracted from the cultured cells and tissue samples using TRIzol reagent (Cat No 15596-026; Thermo Fisher Scientific) according to the manufacturer’s protocol. The concentration and quality of the extracted RNA were determined using a Nanodrop 2000/2000c spectrophotometer (Thermo Fisher Scientific).
Quantitative real-time polymerase chain reaction (qRT-PCR)
Total RNA (2 µg) was reverse-transcribed using a High Capacity complementary deoxyribonucleic acid (cDNA) Reverse Transcription Kit (Cat No 4368814, Thermo Fisher Scientific) to produce lncRNA H19. The samples were evaluated by quantitative polymerase chain reaction (qPCR) using the UltraSYBR Mixture (Cat No CW0957M; CW Biotech, TQB building, No. 1 Yaocheng Avenue, Taizhou City). All qPCR assays were performed on a Light Cycler 480 Instrument II System (Roche, Switzerland). The primer sequences used for qPCR are listed in Table 1.
Table 1
Primer designation | Sequence |
---|---|
HIF1-α | F: GTTTACTAAAGGACAAGTCACC |
R: TCCTGTTTGTTGAAGGGAG | |
MRP1 | F: AACCTGGACCCATTCAGCC |
R: GACTGGATGAGGTCGTCCGT | |
HO-1 | F: ACAGAAGAGGCTAAGACCG |
R: CAGGCATCTCCTTCCATT | |
P-gp | F: TAATGCCGAACACATTGGAA |
R: TCTTCACCTCCAGGCTCAGT | |
H19 | F: GCATGGGAAGTAGGGGTCAC |
R: AGCACAATCCAGGCAACTCC | |
GAPDH | F: TGGAGTCGTACTGGCGTCTT |
R: TGTCATATTCTCGTGGTTCA |
PCR, polymerase chain reaction; HIF1-α, hypoxia-inducible factor 1-α; MRP1, multi-drug resistant associated protein 1; HO-1, heme oxygenase-1; P-gp, p-glycoprotein; GAPDH, glyceraldehyde-3-phosphate dehydrogenase.
Western blot
Proteins extracted from cells cultured under different oxygen conditions were subjected to a western blot test using standard procedures. The primary antibodies are listed in Table 2. They are HIF1-α Rabbit pAb (ABclonal Cat# A11945, RRID:AB_2758885), HO-1 Rabbit pAb (ABclonal Cat# A19062, RRID:AB_2862555), p-gp Rabbit pAb (ABclonal Cat# A19093, RRID:AB_2862585), GAPDH Rabbit pAb (ABclonal Cat# A19056, RRID:AB_2862549), MRP1 Rabbit pAb (Abcam Cat# ab260038, RRID:AB_2889834). The secondary antibodies horseradish peroxidase-labeled goat anti-rabbit immunoglobulin G (IgG) (H + L) (Beyotime Cat# A0208, RRID:AB_2892644) was purchased from Beyotime (Shanghai, China). Protein bands were detected by Western Blotting Substrate (ECL). Glyceraldehyde-3-phosphate dehydrogenase (GAPDH) was used as an internal reference.
Table 2
Antibody name | Company | Article number |
---|---|---|
HIF1-α Rabbit pAb | ABclonal | A11945 |
HO-1 Rabbit pAb | ABclonal | A19062 |
P-gp Rabbit pAb | ABclonal | A19093 |
GAPDH Rabbit pAb | ABclonal | A19056 |
MRP1 Rabbit pAb | Abcam | ab260038 |
HIF1-α, hypoxia-inducible factor 1-α; HO-1, heme oxygenase-1; P-gp, p-glycoprotein; GAPDH, glyceraldehyde-3-phosphate dehydrogenase; MRP1, multi-drug resistant associated protein 1.
Wound healing assay
We used a marker pen to draw horizontal lines evenly behind the orifice plate with a ruler at intervals of approximately every 1 cm across the hole. Each hole contained at least five lines. A549 and H1650 cells were cultured to confluency in a microtiter plate and then a sterilized pipette tip was used to create a scratch perpendicular to the horizontal line on the back. The cells were washed with phosphate buffered saline (PBS) three times, and serum-free media was added. The cells were then incubated at 37 ℃ and 5% CO2 and subsequently photographed at 0, 6, 12, 24, and 48 h.
Transwell experiment
The cell invasion ability of the cells was investigated using Transwell assays. Matrigel was diluted into serum-free media (1:7.5) on ice and gently added to the upper chamber of a Transwell system, which was then incubated at 37 ℃ for 15 min. The cells were then diluted into a serum-free medium (2.5×104/mL) and added to the upper chamber, and a 500 µL medium containing 10% FBS was placed in the lower chamber of the Transwell. The plates were incubated at 37 ℃ for 24 h. Next, the cells were stained with crystal violet. A glass slide was then placed on an upright microscope and the Transwell was placed upside down on a microscope; images were subsequently taken and the cells were analyzed.
Annexin V-Allophycocyanin (APC) flow cytometry
The A549 and H1650 cells were grown to approximately 70% confluency and then treated with a buffer or cisplatin to induce apoptosis. The concentration of cisplatin was 80 µmol/L, and the action time was 24 hours. Next, the cells were stained with Annexin V-APC staining and then analyzed by flow cytometry. Data were analyzed using a t-test.
Statistical analyses
IBM SPSS Statistics (RRID: SCR_019096) (International Business Machines Corporation, USA) and GraphPad Prism (RRID:SCR_002798) (GraphPad Software Inc., San Diego, CA, USA) were used for statistical analysis. For the comparisons between two groups, the means of both samples were compared using the independent sample t-test. Univariate analysis of variance (ANOVA) was used for multi-group comparisons. The qRT-PCR experiments were run in triplicate, and imaging assays were run in quadruplicate. The qPCR data were analyzed by the 2−ΔΔCt method. Statistical significance, α, was set to 0.05. An F test was performed before the t-test to determine whether an equal or unequal variance assumption should be used.
Results
The expressions of HIF1-α, lncRNA H19, MRP1, and HO-1 were elevated under hypoxic conditions in tumor cells
MRP is a membrane transporter and a member of the ABC superfamily (ATP binding cassette transporter family, ABC transporter family), which has at least six members. MRP1 is the most closely related to tumor multidrug resistance. The overexpression of MRP1 in tumor tissues is one of the primary mechanisms of multidrug resistance in lung cancer cells. HO-1 is closely related to the occurrence, invasion, and metastasis of tumors, and its expression in lung cancer is elevated.
To explore the effect of hypoxia on drug resistance, we first examined the mRNA expression levels of HIF1-α, lncRNA H19, MRP1, and HO-1 under different oxygen concentrations using qRT-PCR. We found that the mRNA levels of HIF1-α, lncRNA H19 MRP1, and HO-1 in A549 and H1650 cells were significantly higher under hypoxic conditions than those under normoxic conditions (t-test, Figure 1, P<0.05).
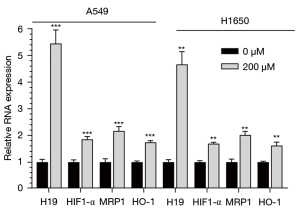
Cancer cell proliferation under normoxic and hypoxic conditions
Next, we evaluated the effect of a hypoxic microenvironment on the drug response of tumor cells. Compared with cells cultured under normoxic conditions, those cultured in hypoxic conditions were significantly more resistant to cisplatin (A549 cells, 19.14 vs. 35.86 µM; H1650 cells, 19.69 vs. 53.09 µM, respectively). The absorbance value at 490 nm and the inhibition rate of cisplatin in A549 and H1650 cells under normoxic and hypoxic conditions are shown in Figure 2 (P<0.05). The differences in absorbance values between cisplatin concentrations were statistically significant (t-test P<0.05, Table 3).
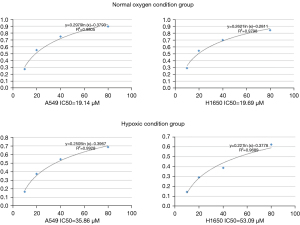
Table 3
Group | Cisplatin (µmol/L) | |||
---|---|---|---|---|
10 | 20 | 40 | 80 | |
Normoxia A549 | 1.79±0.18 | 1.15±0.10 | 0.69±0.05 | 0.35±0.10 |
Hypoxia A549 | 2.05±0.15 | 1.57±0.11** | 1.17±0.13** | 0.84±0.06** |
Normoxia H1650 | 1.74±0.12 | 1.16±0.11 | 0.80±0.16 | 0.46±0.08 |
Hypoxia H1650 | 2.10±0.07* | 1.76±0.07* | 1.53±0.09** | 0.98±0.08** |
Compared with the value of those under plus cisplatin group, *, P≤0.05; **, P≤0.01.
The expression levels of HIF1-α, MRP1, HO-1, and P-gp were elevated in A549 and H1650 cells under hypoxic conditions
P-gp, an important member of the ABC superfamily, can reduce the aggregation of chemotherapeutic drugs in tumor cells. P-gp may be one of the mechanisms of multidrug resistance in lung cancer (24). To explore the relationship between hypoxia and drug resistance of tumor cells, we evaluated the expression levels of HIF1-α, MRP1, HO-1, and P-gp in A549 and H1650 cell lines cultured under hypoxic conditions using western blot (Figure 3). The results showed that the levels of all examined proteins (HIF1-α, MRP1, HO-1, and P-gp) were significantly elevated under hypoxic conditions compared to those under normal culture conditions.
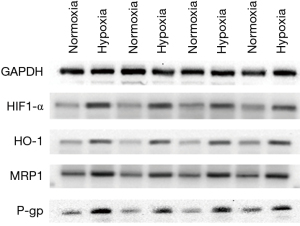
The migration and invasion of tumor cells were increased under hypoxic conditions
Next, we evaluated the migration ability of A549 and H1650 tumor cells using an OrisTM plate cell scratch test. Images were obtained at 0 and 12 h (Figure 4A). Quantification of the migrated cells showed that the migration ability of tumor cells was enhanced under hypoxic conditions (Figure 4B). A549 cell migration was significantly enhanced under hypoxia (one-way ANOVA, F=17.02, P=0.001); similar results were observed in the H1650 cell group (one-way ANOVA, F=35.45, P=0.001).
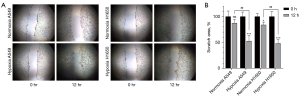
We also examined the invasion ability of tumor cells using Transwell assays (Figure 5A). Quantification of the results showed that tumor cell invasion was significantly enhanced in tumor cells cultured under hypoxic conditions (Figure 5B). The invasive ability of A549 cells was significantly enhanced under hypoxia (t-test, t=10.66, P<0.001); similar results were observed with H1650 cells (t-test, t=−11.34, P<0.001).
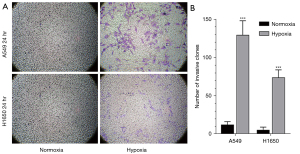
The apoptosis rate of cancer cells treated with cisplatin decreased under hypoxic conditions
A549 and H1650 cells were grown to approximately 70% confluence under different oxygen concentrations and then treated with a buffer or cisplatin. Apoptosis was evaluated using Annexin V-APC staining and flow cytometry (Figure 6A). The apoptosis rate of cancer cells cultured under hypoxic conditions and exposed to cisplatin was significantly decreased compared to cisplatin-treated cells cultured under normoxic conditions (Figure 6B). Compared with the untreated groups, A549 and H1650 cells treated with cisplatin showed markedly increased apoptosis under normoxic and hypoxic conditions.
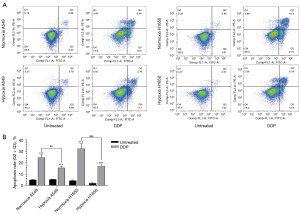
In the normoxia A549 groups, cells treated with cisplatin showed significantly increased apoptosis compared with untreated cells (t-test, t=−12.844, P<0.001); in the hypoxic A549 groups, cells treated with cisplatin showed notably increased apoptosis compared with untreated cells (t-test, t=−12.189, P<0.001); in the normoxia H1650 groups, cells treated with cisplatin showed significantly increased apoptosis compared with untreated cells (t=−11.008, P<0.001); and in the hypoxic H1650 groups, cells treated with cisplatin showed considerably increased apoptosis compared with untreated cells (t-test, t=−12.284, P<0.001).
The apoptosis rates of A549 and H1650 cells in the hypoxia groups treated with cisplatin were significantly lower than those in the normoxia groups (one-way ANOVA, A549 group F=116.181, P<0.001; H1650 group F=97.429, P<0.001). These results suggested that the tolerance of tumor cells to cisplatin is enhanced under hypoxic conditions.
Discussion
Our study showed that hypoxic culture conditions led to upregulated expression of lncRNA H19 in NSCLC cell lines. In addition, hypoxia promoted lung cancer cell proliferation, metastasis, and drug resistance. These findings suggest that lncRNA H19 may be related to the drug resistance of NSCLC. Future studies should examine the role of lncRNA H19 in tumor cell function.
The tumor microenvironment plays key roles in the production and development of cancer cells (5,6). Hypoxia is common in human tumors; the tumor microenvironment of lung cancer tumors is hypoxic and influences the processes of tumor development and invasion (25). Hypoxia promotes tumorigenesis and cancer cell proliferation through a variety of signaling pathways and induces the invasion and migration of cancer cells. Hypoxia also inactivates tumor cells, thereby enabling their escape from the effects of antitumor drugs, which causes resistance to chemotherapy and radiotherapy and leads to tumor recurrence (7,8,26). Hypoxia induces the expression of various genes that encode critical anti-apoptosis and cell proliferation factors, enabling cell survival and resistance to hypoxia and chemoradiotherapy (13,27). The HIF-α transcription factor, which is the major subunit involved in HIF activity, is the main regulator of the hypoxic cellular response. Reducing the expression of HIF1-α in cancer cells or inhibiting its function are potential treatments to limit the proliferation of cancer cells (14,15). LncRNA H19 promotes cancer cell proliferation and metastasis in several solid tumors, including lung, gastric, bladder, and ovarian cancers. LncRNA H19 also induces drug resistance in various cancers, including ovarian, breast, and liver cancers (18-20). In some tumors, hypoxia promotes increased lncRNA H19 expression (21,22).
The expression of lncRNA H19 in alveolar epithelial cells is significantly higher than that in normal lung cancer cells (28). We found that the expressions of lncRNA H19 and HIF1-α in A549 and H1650 cells cultured in a hypoxic environment were significantly higher than those in cells cultured in a normoxic environment. The expression levels of HIF1-α, MRP1, HO-1, and P-gp were markedly increased under hypoxic conditions. The HIF1-α result is consistent with the findings of Bao et al. (29). In recent years, tumor multidrug resistance has emerged continuously, and one of the reasons for MDR is the overexpression of ATP-binding cassette superfamily membrane transporters, including P-gp protein and MRP protein. Both P-gp and MRP1 belong to ATP-dependent drug pumps, and both can use the energy released by the hydrolysis of ATP to transport drugs to the outside of the cell. When P-gp is overexpressed, it increases the efflux of drugs through the action of the efflux pump, reduces the accumulation of drugs in cells, reduces the effect of drugs on cells, and makes tumor cells resistant (30,31). Apoptotic signaling pathways and/or inhibition of apoptosis signaling factors lead to drug resistance in tumor cells (32). MRP1 is one of the important members of MRP protein, and its mechanism of causing MDR is similar to that of P-gp. It can recognize and transport the conjugate formed by the combination of drug and GSH, and pump the drug out of the cell, thus leading to drug resistance (33). HO-1 is a microsomal catalytic enzyme, Tanaka S (34) and others found that HO-1 can inhibit the apoptosis of cancer cells through a variety of mechanisms, protect cancer cells from various oxidative stress damage, and promote tumors. development and transfer. Therefore, elevated expression of P-gp, MRP1 and HO-1 is associated with tumor resistance. However, our findings are the first to demonstrate the increased expression of MRP1, HO-1, and P-gp under hypoxic conditions in NSCLC.
We also found that the migration and invasion of A549 and H1650 cells were notably enhanced under hypoxic conditions. This is consistent with the observation that a hypoxic microenvironment can promote the production and development of NSCLC and increase the invasion and metastasis of NSCLC (9,35-37). Furthermore, we showed that the A549 and H1650 cells in a hypoxic environment were more resistant to cisplatin and that the apoptosis rates of these cell lines decreased significantly in a hypoxic environment. These results are consistent with the findings of previous studies (38,39).
Previous studies have demonstrated the oncogenic role of lncRNA H19. Despite the potential role of lncRNA19 in cancer cells, no study has yet explored the inhibition of lncRNA H19. In addition, there are currently no therapeutic drugs that significantly block the action of lncRNA H19. Further research should examine whether blocking the lncRNA H19 pathway can reverse tumor drug resistance. It may be possible to inhibit or even reverse lncRNA H19-mediated tumor drug resistance via regulation of the HIF pathway. In future studies, we plan to use PX-478, a HIF1-α inhibitor, to reduce HIF1-α and block downstream pathways to determine whether the inhibition of HIF1-α can reverse lncRNA H19-mediated drug resistance. Furthermore, the underlying mechanism of lncRNA H19 leading to drug resistance in NSCLC remains unknown and requires further exploration.
Limitations
This study had some limitations that should be noted. Only cell experiments were carried out; no histopathological or animal experiments were performed to verify the above experimental results. Therefore, more follow-up research is needed.
Conclusions
We found that hypoxic conditions promote the expression of lncRNA H19 in NSCLC cell lines and promote lung cancer proliferation, metastasis, and drug resistance.
Acknowledgments
Funding: This study was supported by 2019 Medical Science and Technology Plan Projects in Zhejiang Province, China, Grant/Award Number 2019KY771.
Footnote
Reporting Checklist: The authors have completed the MDAR reporting checklist. Available at https://tcr.amegroups.com/article/view/10.21037/tcr-22-1812/rc
Data Sharing Statement: Available at https://tcr.amegroups.com/article/view/10.21037/tcr-22-1812/dss
Conflicts of Interest: All authors have completed the ICMJE uniform disclosure form (available at https://tcr.amegroups.com/article/view/10.21037/tcr-22-1812/coif). The authors have no conflicts of interest to declare.
Ethical Statement: The authors are accountable for all aspects of the work in ensuring that questions related to the accuracy or integrity of any part of the work are appropriately investigated and resolved.
Open Access Statement: This is an Open Access article distributed in accordance with the Creative Commons Attribution-NonCommercial-NoDerivs 4.0 International License (CC BY-NC-ND 4.0), which permits the non-commercial replication and distribution of the article with the strict proviso that no changes or edits are made and the original work is properly cited (including links to both the formal publication through the relevant DOI and the license). See: https://creativecommons.org/licenses/by-nc-nd/4.0/.
References
- Siegel RL, Miller KD, Fuchs HE, et al. Cancer Statistics, 2021. CA Cancer J Clin 2021;71:7-33. [Crossref] [PubMed]
- Leidinger P, Keller A, Backes C, et al. MicroRNA expression changes after lung cancer resection: a follow-up study. RNA Biol 2012;9:900-10. [Crossref] [PubMed]
- Ettinger DS, Wood DE, Aisner DL, et al. NCCN Guidelines Insights: Non-Small Cell Lung Cancer, Version 2.2021. J Natl Compr Canc Netw 2021;19:254-66. [Crossref] [PubMed]
- Kapse-Mistry S, Govender T, Srivastava R, et al. Nanodrug delivery in reversing multidrug resistance in cancer cells. Front Pharmacol 2014;5:159. [PubMed]
- Meads MB, Gatenby RA, Dalton WS. Environment-mediated drug resistance: a major contributor to minimal residual disease. Nat Rev Cancer 2009;9:665-74. [Crossref] [PubMed]
- Sausville EA. The challenge of pathway and environment-mediated drug resistance. Cancer Metastasis Rev 2001;20:117-22. [Crossref] [PubMed]
- Triner D, Shah YM. Hypoxia-inducible factors: a central link between inflammation and cancer. J Clin Invest 2016;126:3689-98. [Crossref] [PubMed]
- Krishnamachary B, Berg-Dixon S, Kelly B, et al. Regulation of colon carcinoma cell invasion by hypoxia-inducible factor 1. Cancer Res 2003;63:1138-43. [PubMed]
- Thambi T, Park JH, Lee DS. Hypoxia-responsive nanocarriers for cancer imaging and therapy: recent approaches and future perspectives. Chem Commun (Camb) 2016;52:8492-500. [Crossref] [PubMed]
- Wan J, Wu W, Zhang R. Local recurrence of small cell lung cancer following radiofrequency ablation is induced by HIF-1α expression in the transition zone. Oncol Rep 2016;35:1297-308. [Crossref] [PubMed]
- Macklin PS, McAuliffe J, Pugh CW, et al. Hypoxia and HIF pathway in cancer and the placenta. Placenta 2017;56:8-13. [Crossref] [PubMed]
- Amelio I, Melino G. The p53 family and the hypoxia-inducible factors (HIFs): determinants of cancer progression. Trends Biochem Sci 2015;40:425-34. [Crossref] [PubMed]
- Swinson DE, Jones JL, Cox G, et al. Hypoxia-inducible factor-1 alpha in non small cell lung cancer: relation to growth factor, protease and apoptosis pathways. Int J Cancer 2004;111:43-50. [Crossref] [PubMed]
- Koh MY, Spivak-Kroizman T, Venturini S, et al. Molecular mechanisms for the activity of PX-478, an antitumor inhibitor of the hypoxia-inducible factor-1alpha. Mol Cancer Ther 2008;7:90-100. [Crossref] [PubMed]
- Welsh S, Williams R, Kirkpatrick L, et al. Antitumor activity and pharmacodynamic properties of PX-478, an inhibitor of hypoxia-inducible factor-1alpha. Mol Cancer Ther 2004;3:233-44. [Crossref] [PubMed]
- Osielska MA, Jagodziński PP. Long non-coding RNA as potential biomarkers in non-small-cell lung cancer: What do we know so far? Biomed Pharmacother 2018;101:322-33. [Crossref] [PubMed]
- Zhou J, Xu J, Zhang L, et al. Combined Single-Cell Profiling of lncRNAs and Functional Screening Reveals that H19 Is Pivotal for Embryonic Hematopoietic Stem Cell Development. Cell Stem Cell 2019;24:285-298.e5. [Crossref] [PubMed]
- Chen CL, Ip SM, Cheng D, et al. Loss of imprinting of the IGF-II and H19 genes in epithelial ovarian cancer. Clin Cancer Res 2000;6:474-9. [PubMed]
- Nakagawa H, Chadwick RB, Peltomaki P, et al. Loss of imprinting of the insulin-like growth factor II gene occurs by biallelic methylation in a core region of H19-associated CTCF-binding sites in colorectal cancer. Proc Natl Acad Sci U S A 2001;98:591-6. [Crossref] [PubMed]
- Nielsen MM, Tehler D, Vang S, et al. Identification of expressed and conserved human noncoding RNAs. RNA 2014;20:236-51. [Crossref] [PubMed]
- Matouk IJ, Mezan S, Mizrahi A, et al. The oncofetal H19 RNA connection: hypoxia, p53 and cancer. Biochim Biophys Acta 2010;1803:443-51. [Crossref] [PubMed]
- Wu W, Hu Q, Nie E, et al. Hypoxia induces H19 expression through direct and indirect Hif-1α activity, promoting oncogenic effects in glioblastoma. Sci Rep 2017;7:45029. [Crossref] [PubMed]
- Matouk IJ, Halle D, Gilon M, et al. The non-coding RNAs of the H19-IGF2 imprinted loci: a focus on biological roles and therapeutic potential in Lung Cancer. J Transl Med 2015;13:113. [Crossref] [PubMed]
- Jin JS, Chen SQ, Zhao YF. Expression of multidrug resistance-associated protein (MRP), p-glycoprotein (p-gp) in non-small cell lung cancer (NSCLC) and its clinical significance. Proceeding of Clinical Medicine 2010;19:904-5.
- Jing X, Yang F, Shao C, et al. Role of hypoxia in cancer therapy by regulating the tumor microenvironment. Mol Cancer 2019;18:157. [Crossref] [PubMed]
- Manoochehri Khoshinani H, Afshar S, Najafi R. Hypoxia: A Double-Edged Sword in Cancer Therapy. Cancer Invest 2016;34:536-45. [Crossref] [PubMed]
- Tao J, Yang G, Zhou W, et al. Targeting hypoxic tumor microenvironment in pancreatic cancer. J Hematol Oncol 2021;14:14. [Crossref] [PubMed]
- Xu Y, Lin J, Jin Y, et al. The miRNA hsa-miR-6515-3p potentially contributes to lncRNA H19-mediated-lung cancer metastasis. J Cell Biochem 2019;120:17413-21. [Crossref] [PubMed]
- Bao YX, Ge DJ, Geng Y, et al. Expression of miR-15a and HIF-1α in the condition of low oxygen. Journal of Modern Oncology 2015;23:1637-40.
- Li F, Zhou X, Zhou H, et al. Reducing Both Pgp Overexpression and Drug Efflux with Anti-Cancer Gold-Paclitaxel Nanoconjugates. PLoS One 2016;11:e0160042. [Crossref] [PubMed]
- Koziolová E, Chytil P, Etrych T, et al. Ability of polymer-bound P-glycoprotein inhibitor ritonavir to overcome multidrug resistance in various resistant neuroblastoma cell lines. Anticancer Drugs 2017;28:1126-30. [Crossref] [PubMed]
- Tainton KM, Smyth MJ, Jackson JT, et al. Mutational analysis of P-glycoprotein: suppression of caspase activation in the absence of ATP-dependent drug efflux. Cell Death Differ 2004;11:1028-37. [Crossref] [PubMed]
- Wu W, Chen M, Luo T, et al. ROS and GSH-responsive S-nitrosoglutathione functionalized polymeric nanoparticles to overcome multidrug resistance in cancer. Acta Biomater 2020;103:259-71. [Crossref] [PubMed]
- Tanaka S, Akaike T, Fang J, et al. Antiapoptotic effect of haem oxygenase-1 induced by nitric oxide in experimental solid tumour. Br J Cancer 2003;88:902-9. [Crossref] [PubMed]
- Zhang F, Duan S, Tsai Y, et al. Cisplatin treatment increases stemness through upregulation of hypoxia-inducible factors by interleukin-6 in non-small cell lung cancer. Cancer Sci 2016;107:746-54. [Crossref] [PubMed]
- Sun JC, He F, Yi W, et al. High expression of HIF-2α and its anti-radiotherapy effect in lung cancer stem cells. Genet Mol Res 2015;14:18110-20. [Crossref] [PubMed]
- Wohlkoenig C, Leithner K, Olschewski A, Olschewski H, Hrzenjak A. TR3 is involved in hypoxia-induced apoptosis resistance in lung cancer cells downstream of HIF-1α. Lung Cancer 2017;111:15-22. [Crossref] [PubMed]
- Zhu YN, Zhang L, Zhang Y, et al. Experimental study of chemoresistance of lung adenocarcinoma A549 cells to cisplatin under hypoxic environment. Journal of Modern Oncology 2017;25:3205-9.
- Xie Z, Dong HM, Wei YL, et al. The Influence of hypoxia microenvironment on metastasis induced by epithelial-mesenchymal transition. Chinese Journal of Respiratory and Critical Care Medicine 2016;15:465-9.
(English Language Editor: A. Kassem)