Inhibition of autophagy by 3-methyladenine promotes migration and invasion of colon cancer cells through epithelial mesenchymal transformation
Introduction
Colon cancer is one of the most common malignancies and is the third leading cause of cancer-related deaths worldwide (1,2). Despite significant progress in diagnosis and treatment, cancer metastasis remains the main cause of death. Cancer metastasis can be achieved by activating the epithelial mesenchymal transformation (EMT) process. EMT is characterized by the loss of tight junction protein E-cadherin, the expression of mesenchymal markers such as vimentin, and an increase in cell motility, which drives the cascade of invasion and metastasis (3-6).
Autophagy is an evolutionarily conserved catabolic process that maintains the stability of the intracellular environment (7). In tumors, autophagy plays different roles in different cells and tissues. It can promote or inhibit the development of tumors (8,9). Microtubule-associated protein light chain 3B (MAPLC3B, hereafter referred to as LC3B) is a marker of the autophagy pathway. When autophagy is induced, LC3B-I is transformed into LC3B-II, and the ratio of LC3B-II/LC3B-I is related to the number of autophagosomes (10). In addition, autophagy plays a major role in the degradation of selective autophagy receptors and the signal regulatory protein SQSTM1/p62 (hereafter referred to as p62). Therefore, the conversion of LC3B-I into LC3B-II and the selective degradation of p62 are widely used as markers for monitoring autophagic activity (10).
There is much evidence demonstrating that inhibition of autophagy can improve cancer treatment. Early clinical trials have shown that lysosomal inhibitors, such as hydroxychloroquine, have a good anticancer effect by inhibiting autophagy (11), however, studies have found that inhibition of autophagy may have adverse effects in cancer treatment (11). It is also possible that different inhibitors exert different effects. 3-methyladenine (3-MA) can inhibit phosphatidylinositol 3-kinase (PI3K) activity and prevent the formation of autophagosomes and autophagic vacuoles (10). The effects of inhibition of autophagy by 3-MA on the EMT and metastasis of colon cancer cells are unclear.
The transcription factor Twist1 is a basic helix-loop-helix protein and is a core regulator of early embryonic morphogenesis and cancer development and metastasis (12,13). Twist1 results in loss of adhesion in epithelial cells by downregulating the expression of E-cadherin, thereby promoting EMT and cancer metastasis (3-6,12,13). There is currently a paucity of data on the effects of autophagy on Twist1 in colon cancer. This investigation examined the effects of inhibition of autophagy by 3-MA on Twist1 expression and cancer cell invasion in colon cancer cell lines, so as to provide theoretical support for potential colon cancer treatments. We present the following article in accordance with the MDAR reporting checklist (available at https://tcr.amegroups.com/article/view/10.21037/tcr-22-1736/rc).
Methods
Tissue samples
A total of 56 paraffin-embedded colon cancer samples were obtained from the Department of Pathology at The Second Hospital of Tianjin Medical University. Clinical, demographic data, and pathology, including age, gender, primary site, metastatic site, and lymph node involvement, were collated from electronic medical records. Histologically, there were 42 cases of low-grade tumor and 14 cases of high-grade tumor. This study was approved by the Ethics Committee of The Second Hospital of Tianjin Medical University (No. KY2022K184) and conducted in accordance with the Declaration of Helsinki (as revised in 2013). Individual consent for this retrospective analysis was waived.
Immunohistochemical staining
Sections (4 µm thick) of routine formalin-fixed and paraffin-embedded tissues were deparaffinized, rehydrated, and subjected to antigen retrieval. Immunohistochemical staining was performed according to the manufacturer’s recommendations with a fully automated system (Leica Biosystems, Bond-Max, Germany). Sections were stained with an anti-Twist1 antibody (25465-1-AP, Proteintech, USA). Appropriate positive and negative controls were used.
Cell culture and treatment
The human colon cancer cell lines LOVO and SW620 were obtained from the American Type Culture Collection (ATCC, USA). Cells were cultured and passaged according to standard protocols. Cells were cultured in RPMI 1640 medium (Gibco, Grand Island, NY, USA) containing 10% fetal bovine serum (Gibco) at 37 ℃ in a humidified incubator (SANYO, Tokyo, Japan) with 5% CO2. For the in vitro experiments, cells were co-cultured with the autophagy inhibitor 3-MA (5 mM; MCE, USA) for 0, 24, and 48 hours. We had blank group and 3-MA group. Subsequently, the cells were prepared for the blank group and 3-MA group.
Wound healing assay
Colon cells treated with or without 3-MA were collected and seeded in 6-well plates. When the cells reached approximately 80% confluency, the bottom of the well was scratched uniformly using sterile pipette tips. After scratching, the cells were washed three times with phosphate buffered saline (PBS) and observed under light microscopy at 0, 24, and 48 hours to assess the width of the scratch line.
Transwell assays
Cells were seeded in Matrigel Invasion Chambers (EMD Millipore, Billerica, MA, USA) in a 24-well culture plate. After incubation for 24 or 48 hours at 37 ℃ in an atmosphere with 5% CO2, noninvading cells within the insert chamber were removed, and the upper layer of the transwell was briefly wiped with a cotton swab. The bottom of the membrane was fixed with 4% paraformaldehyde for 30 minutes, stained with 0.1% crystal violet for 10 minutes, and then imaged. The invading cells on the membrane were counted under a bright-field microscope (Zeiss, Oberkochen, Germany).
Quantitative reverse transcription polymerase chain reaction (qRT-PCR) analysis
Total RNA was extracted from cells using TRIzol reagents (Invitrogen, MA, USA) according to the manufacturer’s protocol. The Takara Reverse Transcription Kit (Takara, Japan) was used to synthesize the cDNA. A SYBR Premix ExTaq kit (Takara, Japan) and Thermal Cycler DiceTM Real Time System III (Takara, Japan) were used for RT-PCR. Expression levels in each sample were normalized to the expression level of β-actin. The 2−ΔΔCt method was applied to analyze mRNA expression with β-actin as the normalization control. The following primer sequences were used: Twist1 forward 5'TCTACCAGGTCCTCC AGAGC3'; Twist1 reverse 5'CTCCATCCTCCAGACCGAGA3'; β-actin forward 5'CGTGACATTAAGGAGAAGCTG3'; β-actin reverse 5'CTAGAA GCATTTGCGGTGGAC3'.
Western Blot analysis
Cells were homogenized with RIPA lysis buffer (Sigma, MA, USA) to extract the protein content and the concentration was detected using the BCA Protein Quantitative Kit (Beyotime, Beijing, China). Protein samples were diluted in 5× sodium dodecyl sulfate (SDS) sample loading buffer. Equal amounts of protein (10 µg) in a 10 µL volume were separated on 10% SDS-polyacrylamide gel electrophoresis (PAGE) gels and transferred to polyvinylidene difluoride (PVDF) membranes (Merck Millipore, MA, USA). Membranes were incubated overnight at 4 ℃ with primary antibodies specific for E-cadherin (EP6, Zsbio, China), vimentin (EP21, Zsbio, China), p62 (18420-1-AP, Proteintech, USA), Twist1 (25465-1-AP, Proteintech, USA), light chain 3 (LC3; 18420-1-AP, Proteintech, USA), and glyceraldehyde 3-phosphate dehydrogenase (GAPDH) (ab53573, Abcam, UK). Thereafter, membranes were incubated with horseradish peroxidase-conjugated secondary antibodies (Zsbio, Beijing, China) at 25 ℃ for 1.5 hours. Antibody solutions were diluted in 5% milk in Tris-buffered saline/Tween (TBS/T) buffer. Specific protein bands were visualized using an ECL substrate kit (Merck Millipore, MA, USA). The ImageJ software was used to determine the relative expression of the target protein to GAPDH.
Immunofluorescence staining
For immunofluorescence staining, cells were cultured on glass coverslips (24-well plates). The cells were washed with PBS, fixed with 4% paraformaldehyde for 20 minutes, and permeabilized with 0.2% Triton X-100 in PBS for 4 minutes. Subsequently, the cells were incubated overnight at 4 ℃ with the appropriate primary antibodies diluted in PBS containing 1% bovine serum albumin (BSA). The cells were washed 3 times and then incubated for 45 minutes with FITC-conjugated donkey anti-rabbit secondary antibodies diluted in the same buffer. Imaging was performed using a fluorescence microscope (Olympus, Japan).
Gene Expression Profiling Interactive Analysis (GEPIA)
The GEPIA platform (http://gepia2.cancer-pku.cn/#index) is an interactive web server for cancer expression profile data which integrates gene expression profile data from The Cancer Genome Atlas (TCGA) and Genotype-Tissue Expression (GTEX) projects. Data related to the transcriptional expression of Twist1 in colon cancer tissues and normal tissues were downloaded from GEPIA.
Statistical analysis
Statistical analyses were performed using GraphPad Prism 7.0 software (La Jolla, CA, USA). Student’s t-tests were performed on samples that passed the Shapiro-Wilk normality test and variance homogeneity test. Nonparametric Wilcoxon signed-rank tests were performed for samples that did not exhibit a normal distribution or variance unequal. Data are presented as the mean ± standard deviation (SD). A P value <0.05 was considered statistically significant. All experiments were technically repeated 3 times, and the results were taken as the average.
Results
Inhibition of autophagy in colon cancer cells by 3-MA
Western blotting analysis showed that LOVO and SW480 cells treated with 3-MA had significantly decreased ratios of LC3B-II/LC3B-I and significantly elevated levels of p62 compared to untreated cells (Figure 1A). Immunofluorescence staining showed much more p62 accumulation in cells treated with 3-MA (Figure 1B), indicating that 3-MA effectively inhibited autophagy.
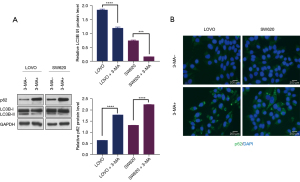
Inhibition of autophagy by 3-MA promotes the migration and invasion of colon cancer cells
To investigate whether the inhibition of autophagy by 3-MA has an effect on the function of colon cancer cells, the migration and invasion abilities of colon cancer cells before and after 3-MA treatment were investigated. Wound healing assays showed that cells treated with 3-MA migrated at a significantly faster rate in the leading edge of the scratch compared with untreated control cells (Figure 2A). Similarly, transwell assays showed that the number of cells per field markedly increased after 3-MA treatment (Figure 2B). This suggested that inhibition of autophagy by 3-MA promoted the migration and invasion of colon cancer cells.
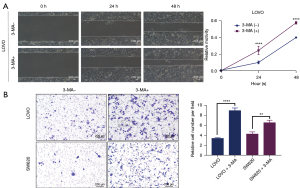
Inhibition of autophagy by 3-MA increases vimentin expression and decreases E-cadherin expression in colon cancer cells
To further investigate whether the inhibition of autophagy by 3-MA triggered the EMT process, the expression of EMT-related proteins was evaluated. Western blotting analysis showed that the expression of epithelial marker E-cadherin was significantly decreased in colon cancer cells treated with 3-MA compared with untreated cells (Figure 3A). However, the expression of the mesenchymal marker vimentin was markedly upregulated in colon cancer cells treated with 3-MA (Figure 3B). These results suggested that autophagy inhibition changed the colon cancer cell traits and induced EMT.
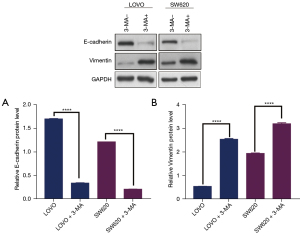
The expression of Twist1 in tumor tissues and normal tissues of colon cancer
To clarify the mechanisms involved in the regulation of EMT by inhibiting autophagy, the expression of Twist1 in colon cancer tissue and cells was examined. The GEPIA platform was used to analyze the expression of Twist1 in tumor tissues and normal tissues of colon cancer patients. The results showed that the expression of Twistl increased slightly in tumor tissues, but there was no significant difference between tumor tissues and normal tissues (Figure 4A). Furthermore, immunohistochemistry staining revealed that Twist1 was expressed in both normal and colon cancer tissues, and the staining range and intensity were comparable (Figure 4B). These results are consistent with the GEPIA analysis.
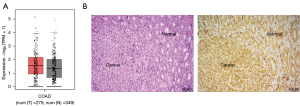
Inhibition of autophagy by 3-MA increases Twist1 expression in colon cancer cells
In vitro studies revealed that the mRNA (Figure 5A) and protein (Figure 5B) expression of Twist1 increased significantly in response to autophagy inhibition. The upregulation of Twist1 expression occurred in parallel with a decrease in E-cadherin expression and an increase in vimentin expression. Immunofluorescence staining showed higher Twist1 expression in cells treated with 3-MA compared to untreated cells (Figure 5C), suggesting that inhibition of autophagy promoted EMT by upregulating the expression of Twist1.
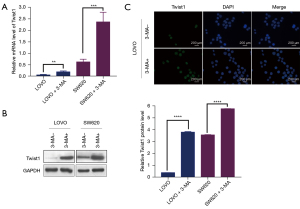
Discussion
This study demonstrated that inhibition of autophagy by 3-MA induced EMT in colon cancer cells. The role of autophagy in EMT has been widely reported, but the results are controversial. Several studies have shown that autophagy promotes EMT. For example, Alizadeh et al. (14) demonstrated that autophagy positive regulation of transforming growth factor (TGF)-β1 induced EMT in non-small cell lung cancer cell lines. Similarly, Dash et al. (15) reported that autophagy promoted EMT and metastasis by regulating the level of reactive oxygen species in hepatocellular carcinoma cells. However, recent investigations on different types of cancer have reported otherwise. Qiang et al. (16) demonstrated that autophagy mediates p62-dependent Twist1 degradation and promotes cell proliferation and migration in autophagy-related gene (ATG) knockout mouse embryonic fibroblasts and human squamous cell carcinoma cells. In addition, autophagy-dependent SNAI1 degradation inhibits EMT and metastasis of cancer cells in lung cancer and cervical cancer cell lines (17). Taken together, these studies suggest that the role of autophagy in EMT may have tissue heterogeneity, or produce different effects under different conditions.
The results of the few studies examining the role of autophagy in EMT of colon cancer cells remain controversial. For example, suppression of beclin-1 (a key molecule involved in autophagy activation) expression through gene knockout significantly reduced EMT and invasive behaviors in colon cancer cells (18). However, according to an updated report by Islam Khan et al. (19), RAMS11 promotes colorectal cancer through mTOR-dependent inhibition of autophagy and promotion of EMT. In this study, we found that inhibition of autophagy by 3-MA decreased the expression of E-cadherin, increased the expression of vimentin, and promoted the migration and invasion of colon cancer cells. These results suggested that there is a close correlation between autophagy and cell phenotype or the EMT process in colon cancer. In addition, inhibition of autophagy resulted in p62 accumulation and upregulation of the EMT-related transcription factor Twist1. Studies have shown that p62 contains domains that interact with many signal activators (20). P62 accumulated during autophagy deficiency blocks the degradation of Twist1 through the interaction between its ubiquitin related domain and Twist1, and then regulates the expression of E-cadherin, induces EMT, and promotes tumor metastasis (21,22). Based on our data, we speculate that the EMT process of colon cancer might follow this regulatory pathway.
It is noteworthy that there was no significant difference in the expression of Twist1 in normal tissues and tumor tissues of colon cancer patients as analyzed using data from the GEPIA database. The Twist1 protein is an unstable protein regulated by the ubiquitin proteasome system through F-box protein and E3 ubiquitin ligase (23). It is degraded by autophagy and other pathways, such as the proteasome system, and can change dynamically in vivo. In addition, the expression and cellular localization of Twist1 drives the resultant downregulation or loss of E-cadherin expression, and for Twist1 to function as an activator and/or repressor of the transcription of a target gene, efficient nuclear localization is essential (24). Further research is required to clarify the intracellular localization of Twist1 in colon cancer tissue, so as to understand its functional role.
There is much evidence in the literature and clinical trials showing that autophagy inhibitors combined with chemotherapy or targeted therapy will benefit cancer patients (11,25). This study demonstrated that inhibition of autophagy by 3-MA promoted the migration and invasion of colon cancer cells, suggesting that inhibition of autophagy may have adverse effects on colon cancer, at least under certain conditions. In addition, Twist1 is a key downstream regulator of p62. It is speculated that targeting p62-mediated Twist1 stabilization may be a promising cancer prevention and treatment strategy.
In conclusion, this investigation demonstrated that inhibition of autophagy upregulates the expression of Twist1 in colon cancer cells and promotes cancer cell migration and invasion through EMT. Furthermore, inhibition of autophagy may have adverse effects on colon cancer.
Acknowledgments
Funding: This work was supported by a grant from the Scientific Research Project of Tianjin of China (No. ZC20028).
Footnote
Reporting Checklist: The authors have completed the MDAR reporting checklist. Available at https://tcr.amegroups.com/article/view/10.21037/tcr-22-1736/rc
Data Sharing Statement: Available at https://tcr.amegroups.com/article/view/10.21037/tcr-22-1736/dss
Conflicts of Interest: All authors have completed the ICMJE uniform disclosure form (available at https://tcr.amegroups.com/article/view/10.21037/tcr-22-1736/coif). The authors have no conflicts of interest to declare.
Ethical Statement: The authors are accountable for all aspects of the work in ensuring that questions related to the accuracy or integrity of any part of the work are appropriately investigated and resolved. The study was conducted in accordance with the Declaration of Helsinki (as revised in 2013). The study was approved by the Ethics Committee of The Second Hospital of Tianjin Medical University (No. KY2022K184) and individual consent for this retrospective analysis was waived.
Open Access Statement: This is an Open Access article distributed in accordance with the Creative Commons Attribution-NonCommercial-NoDerivs 4.0 International License (CC BY-NC-ND 4.0), which permits the non-commercial replication and distribution of the article with the strict proviso that no changes or edits are made and the original work is properly cited (including links to both the formal publication through the relevant DOI and the license). See: https://creativecommons.org/licenses/by-nc-nd/4.0/.
References
- Chen W, Zheng R, Baade PD, et al. Cancer statistics in China, 2015. CA Cancer J Clin 2016;66:115-32. [Crossref] [PubMed]
- Siegel RL, Miller KD, Goding Sauer A, et al. Colorectal cancer statistics, 2020. CA Cancer J Clin 2020;70:145-64. [Crossref] [PubMed]
- Mittal V. Epithelial Mesenchymal Transition in Tumor Metastasis. Annu Rev Pathol 2018;13:395-412. [Crossref] [PubMed]
- Nieto MA, Huang RY, Jackson RA, et al. EMT: 2016. Cell 2016;166:21-45. [Crossref] [PubMed]
- Yang J, Antin P, Berx G, et al. Guidelines and definitions for research on epithelial-mesenchymal transition. Nat Rev Mol Cell Biol 2020;21:341-52. [Crossref] [PubMed]
- Tam WL, Weinberg RA. The epigenetics of epithelial-mesenchymal plasticity in cancer. Nat Med 2013;19:1438-49. [Crossref] [PubMed]
- Zachari M, Ganley IG. The mammalian ULK1 complex and autophagy initiation. Essays Biochem 2017;61:585-96. [Crossref] [PubMed]
- Levy JMM, Towers CG, Thorburn A. Targeting autophagy in cancer. Nat Rev Cancer 2017;17:528-42. [Crossref] [PubMed]
- Galluzzi L, Pietrocola F, Bravo-San Pedro JM, et al. Autophagy in malignant transformation and cancer progression. EMBO J 2015;34:856-80. [Crossref] [PubMed]
- Klionsky DJ, Abdelmohsen K, Abe A, et al. Guidelines for the use and interpretation of assays for monitoring autophagy (3rd edition). Autophagy 2016;12:1-222.
- Amaravadi RK, Kimmelman AC, Debnath J. Targeting Autophagy in Cancer: Recent Advances and Future Directions. Cancer Discov 2019;9:1167-81. [Crossref] [PubMed]
- Qin Q, Xu Y, He T, et al. Normal and disease-related biological functions of Twist1 and underlying molecular mechanisms. Cell Res 2012;22:90-106. [Crossref] [PubMed]
- Yang J, Mani SA, Donaher JL, et al. Twist, a master regulator of morphogenesis, plays an essential role in tumor metastasis. Cell 2004;117:927-39. [Crossref] [PubMed]
- Alizadeh J, Glogowska A, Thliveris J, et al. Autophagy modulates transforming growth factor beta 1 induced epithelial to mesenchymal transition in non-small cell lung cancer cells. Biochim Biophys Acta Mol Cell Res 2018;1865:749-68. [Crossref] [PubMed]
- Dash S, Sarashetti PM, Rajashekar B, et al. TGF-β2-induced EMT is dampened by inhibition of autophagy and TNF-α treatment. Oncotarget 2018;9:6433-49. [Crossref] [PubMed]
- Qiang L, Zhao B, Ming M, et al. Regulation of cell proliferation and migration by p62 through stabilization of Twist1. Proc Natl Acad Sci U S A 2014;111:9241-6. [Crossref] [PubMed]
- Zada S, Hwang JS, Ahmed M, et al. Control of the Epithelial-to-Mesenchymal Transition and Cancer Metastasis by Autophagy-Dependent SNAI1 Degradation. Cells 2019;8:129. [Crossref] [PubMed]
- Shen H, Yin L, Deng G, et al. Knockdown of Beclin-1 impairs epithelial-mesenchymal transition of colon cancer cells. J Cell Biochem 2018;119:7022-31. [Crossref] [PubMed]
- Islam Khan MZ, Law HKW. RAMS11 promotes CRC through mTOR-dependent inhibition of autophagy, suppression of apoptosis, and promotion of epithelial-mesenchymal transition. Cancer Cell Int 2021;21:321. [Crossref] [PubMed]
- Komatsu M, Kageyama S, Ichimura Y. p62/SQSTM1/A170: physiology and pathology. Pharmacol Res 2012;66:457-62. [Crossref] [PubMed]
- Bertrand M, Petit V, Jain A, et al. SQSTM1/p62 regulates the expression of junctional proteins through epithelial-mesenchymal transition factors. Cell Cycle 2015;14:364-74. [Crossref] [PubMed]
- Karreth F, Tuveson DA. Twist induces an epithelial-mesenchymal transition to facilitate tumor metastasis. Cancer Biol Ther 2004;3:1058-9. [Crossref] [PubMed]
- Lander R, Nordin K, LaBonne C. The F-box protein Ppa is a common regulator of core EMT factors Twist, Snail, Slug, and Sip1. J Cell Biol 2011;194:17-25. [Crossref] [PubMed]
- Schwoebel ED, Moore MS. The control of gene expression by regulated nuclear transport. Essays Biochem 2000;36:105-13. [Crossref] [PubMed]
- Chude CI, Amaravadi RK. Targeting Autophagy in Cancer: Update on Clinical Trials and Novel Inhibitors. Int J Mol Sci 2017;18:1279. [Crossref] [PubMed]
(English Language Editor: J. Teoh)