Study on the value of multi-dimensional conformal radiotherapy and functional imaging in tumor bioimaging
Introduction
Modern radiotherapy plays an important role in the treatment of cancer. New radiotherapy methods and various disciplines are continuously emerging in an increasingly active field (1). With the rapid development of advanced technologies such as image guidance, adaptive radiotherapy, artificial intelligence (AI) integration, heavy particle therapy, and flash ultra-high dose rate, modern radiotherapy presents multiple features (2-5): the diversity and multi-mode capacity of radiotherapy data, the high precision, rapidity, real-time assessment, and functionality of radiotherapy technology, and the multidisciplinary integration and AI of radiotherapy technology (6). The application of AI in the intelligent and automatic delineation of tumor radiotherapy target areas and organs at risk, the establishment of a radiotherapy planning model, and the establishment of a remote intelligent quality control system are well underway (7). The multi-dimensional, multi-modal, and unique characteristics of big data of modern radiotherapy, as well as the new service mode based on “internet + radiotherapy”, “artificial intelligence radiotherapy”, and “shared radiotherapy”, promote the progress and development of radiotherapy technology towards the direction of digitalization, automation, and intelligence (8-10). Recent advances in cancer detection, diagnosis, and treatment have prompted the need for new types of imaging. Achieving this development will require improved imaging and radiotherapy techniques, vast advances in cancer knowledge at the molecular level, and the integration of multiple disciplines. Where current medical imaging mainly shows anatomical structures, new imaging types should provide biological and functional data, such as positron emission tomography (PET) scans that display metabolic signals using the radioisotope f-labeled fluorodeoxyglucose (FDG). Functional metabolic data can be studied from magnetic resonance imaging and spectroscopy (MRI/MRS) (11). Further development of noninvasive molecular imaging can specifically express tumor genotypes and phenotypes. Considering that “new” imaging techniques display a wide spectrum of signals, it has been suggested that such images (as opposed to actual anatomy) be referred to as “biological” images. Biological imaging is extensive, including metabolic, biochemical, physiological, and functional imaging, as well as genotypes and phenotypes of molecules currently under study. For radiation therapy, radiobiological images show signals that influence factors such as radiosensitivity and treatment outcome (e.g., tumor hypoxia) (12).
Functional imaging is an imaging method that uses radionuclides or radionuclide markers as tracers to reflect the biochemical process of the body through its uptake and metabolism by body cells, or uses functional imaging software to reflect the changes of body functions. Functional imaging mainly includes PET, single photon emission computed tomography (SPECT), and MRS, among others (13). It can not only provide images of tissue and cell metabolism, proliferation, blood supply, hypoxic state, and even gene phenotype, but also provide early diagnosis of tumors, analysis of tumor process, follow-up, and prediction of curative effect, making it a reality to study the changes of microenvironment in vivo and understand the development of tumors non-invasively. Molecular biology and clinical medicine have lacking a “bridge”, but functional imaging technology can organically connect this gap (14-16).
Radiotherapy is one of the most important treatment methods for malignant tumors. Seventy percent of patients with malignant tumors require radiotherapy at different stages of treatment, of which 70 percent are radical radiotherapy, and 70 percent of patients who receive radical radiotherapy are eventually cured. Radiotherapy contributes 40% of curable malignancies. High quality imaging information is the basis of precision radiotherapy for tumor tissue. The development of medical imaging technology makes the relationship between tumor and normal tissue clearer, the delineation of radiotherapy target more accurate, and the judgment of radiotherapy efficacy and injury clearer. Image guidance techniques include anatomical image guidance techniques [electronic portal imaging device (EPID), cone beam computed tomography (CBCT), ultrasound, magnetic resonance imaging (MRI), et al.] and functional image guidance techniques [PET-CT, hypoxic imaging, epidermal growth factor receptor (EGFR) imaging, perfusion imaging, etc.]. The development of anatomical image guidance technology is increasingly mature, and the functional image guidance technology is currently in full swing, which is the hot spot and direction of future development. At present, CBCT is usually used in image guidance technology. In this way, it is sometimes difficult to fully show the difference between tumor tissue and normal tissue, even more difficult to distinguish between nerve, muscle and other soft tissue structures.
Radiotherapy (hereinafter referred to as radiotherapy) plays an important role in tumor treatment. About 80% of tumor patients need radiotherapy. Radiotherapy not only has positive phase killing effect on tumor, but also has negative phase damage effect on immune function, causing the readjust of immune functional cells in patients.
At present, surgery, radiotherapy, and chemotherapy are the main treatment methods for brain metastases (BMs). With the continuous improvement of tumor treatment methods and the relative extension of survival time of tumor patients, the incidence of BMs is also increasing and is currently about 20–35%, which is the main cause of death and disability of tumor patients at present. Whole brain radiotherapy is often used in the clinical treatment of BMs and is regarded as the standard treatment (17). Combined with radiosurgery, it can effectively prolong the survival period. With the development of clinical research, the application of conformal radiotherapy in clinical practice has improved and advancements have been made in dose distribution (18). However, there are shortcomings in radiation accuracy, which can also affect normal tissues. It has been suggested that image-guided whole-brain intensity-modulated radiotherapy combined with dose-enhancing therapy [multi-dimensional conformal radiotherapy (MD-CRT)] can effectively improve the therapeutic effect for patients with BMs. In this study, 150 patients were selected to explore the effects of image-guided (MD-CRT) for BM. We present the following article in accordance with the MDAR reporting checklist (available at https://tcr.amegroups.com/article/view/10.21037/tcr-22-2005/rc).
Methods
General information
A total of 150 patients with BMs were retrospectively selected as the study participants from December 2020 to December 2021. The male to female ratio of participants was 84:66; the youngest was 37 years old, and the oldest was 61 years old, with an average of 51.64±4.17 years old. There were 114 cases of lung cancer, 27 cases of breast cancer, and 9 cases of ovarian cancer. The study was conducted in accordance with the Declaration of Helsinki (as revised in 2013). The study was approved by ethics board of the First Affiliated Hospital of Xi’an Jiaotong University (No. 20220024) and individual consent for this retrospective analysis was waived.
Inclusion and exclusion criteria
The inclusion criteria included the availability of complete patient information; diagnosis by imaging and clinical symptoms including dizziness, headache, oblique mouth, vomiting, lethargy, and other symptoms; that the patient was cognitively normal; and all were familiar with the study and volunteered to participate. The exclusion criteria included mental abnormality; other cerebrovascular diseases; and lack of cooperation with the investigators.
Research methods
All participants were treated with image-guided MD-CRT combined with increased dosage for brain metastasis: patients were instructed to maintain the supine position, the head was fixed with thermoplastic cranium, an enhanced computed tomography (CT) scan was performed from the cranial top to the lower margin of the mandible, and treatment plans were formulated according to the actual situation. Guiding correct position and the implementation of megavoltage cone-beam computed tomography (MV-CBCT) positioning, through the image acquisition, the window corresponding to the sagittal plane, coronal, and transverse section was conducted with reference to the image to compare and adjust alignment, and control any direction error of more than 2 mm. The error was also controlled regarding accelerator transmission and treatment positioning for calibration, and an image results summary was generated to attain a rough sketch of the target tumor volume. Based on the size of the clinical target volume, 3 mm was placed outward as the planned target volume, and the distance from the epidermis was about 4 mm. Normal tissues, including spinal cord, optic chiasma, optic nerve, and brain stem, were outlined under CT guidance to ensure that the acceptable prescription dose was 95% of the target volume. The volume prescription dose of the gross tumor was set as follows: Dt 4,994–6,990 cGy for 22–30 times every 4.4–6.0 weeks. The prescribed dose of clinical target volume was set as follows: Dt 3,994–5,000 cGy every 4.4–6.0 weeks, 22–30 times. We limited the dose received by normal organs, and controlled the dose of optic chiasma, optic nerve, and brain stem below 50 Gy, and controlled the dose of the spinal cord below 40 Gy.
Observational index
The short-term treatment effect of the patients was evaluated, the dose received by irradiation and the incidence of adverse reactions were counted, and all patients were followed up to evaluate the long-term efficacy. Short-term treatment effects comprised disease progression, stability, partial response (PR), and complete response (CR), and was evaluated strictly in accordance with the new Response Evaluation Criteria in Solid Tumors (RECIST) version 1.1 (https://ctep.cancer.gov/protocoldevelopment/docs/recist_guideline.pdf). The number of patients with CR and PR was equal to the efficacy of treatment.
Statistical analysis
A bilateral unpaired t-test or Mann-Whitney U test was used to analyze the statistical significance of differences between groups. All data were presented as the mean ± standard deviation of at least three independent trials. A P value <0.05 was considered statistically significant.
Results
Treatment effect analysis of all participants
Among the 150 patients, 24 cases were in CR, 72 cases were in PR, 36 cases were in a stable condition, and 18 cases were in deterioration (Table 1).
Table 1
Sectionalization | Cases | Percentage, % |
---|---|---|
CR | 24 | 16.00 |
PR | 72 | 48.00 |
Stable condition | 36 | 24.00 |
Deterioration | 18 | 12.00 |
Total | 150 | 100.00 |
CR, complete response; PR, partial response.
Analysis of treatment
All participants were followed up, the survival was 62.37±1.24 months, 96 cases (64.00%) survived, 54 cases (36.00%) had died. The radiation dose of tumor volume ranged from 58 to 69 Gy, with an average dose of 62.09±3.94 Gy. In terms of adverse reactions, brain edema occurred in 57 patients, accounting for 38.00%.
Functional imaging analysis
The low frequencies usually contain the main components of the source image, whereas the high frequencies retain more detail of the source image. In view of the limitations of traditional fusion strategies, the vGG-19 model (MATLAB; MathWorks, Natick, MA, USA) is used. Finally, the low frequency coefficient is a fusion of the source image and the weight map (Figure 1).
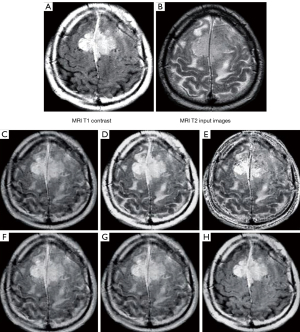
Image fusion feature
Multimodal medical image fusion plays an extremely important role in human brain structure, lesion localization, disease diagnosis and treatment planning. Medical images have different image modes, which can be divided into anatomical images and functional images. Anatomical images include computed tomography images, magnetic resonance images, and ultrasound images, which provide details of the body’s morphological anatomy. This may lose the edge and texture features of the image, but adds two diagonal gradient changes to the regular method, which can be used to stimulate datacenter-ready secure control module (DC-SCM) analysis (Figure 2).
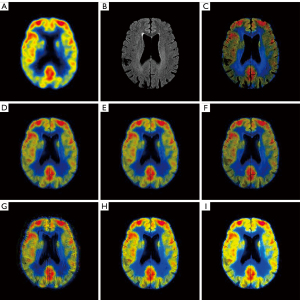
Multimodal medical image fusion
Multimodal medical image fusion is an important method to fuse the complementary features of different imaging methods into a single fused image. Clinical choice of different fusion images still needs to be a doctor visually inspected to complete its own experience, the lack of evaluation standard of medical image fusion, the existing objective quality evaluation method was not designed for medical image fusion, when evaluating multimodal fusion image has great limitations, and difficult to into the design and optimization of image fusion. Therefore, the study of multimodal medical image fusion and quality evaluation has important clinical application value (Figure 3).
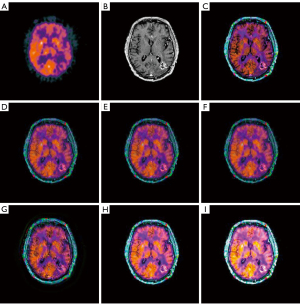
The role of functional imaging techniques in multidimensional radiotherapy
Image fusion can be used to detect the skull base, blood vessels, and recurrent targets that are difficult to delineate in conventional MR and CT imaging. Fusion of multiple images on CT is very important for craniocerebral tumors, especially during radiotherapy, and it can improve therapeutic efficacy and reduce complications. Follow-up examinations using image fusion techniques are also helpful in clinical practice. Assessment of intracranial tumor margin recurrence may reduce the incidence of adverse events associated with surgical revision. In addition, the study of vascular occlusion sequence may hint at the influence of radiotherapy on arteriovenous malformation (AVM), which may help clinicians to adjust the radiotherapy dose and develop a more effective radiotherapy regimen (Figure 4).
The role of biofunctional imaging in precision radiotherapy of tumors
Accurate target detection is critical for radiosurgery, neurosurgery, and low-fractional radiotherapy because treatment outcomes and complication rates are related to the accuracy of target definition. In tumors at the base of the skull and around the visual pathway, an accurate anatomical assessment of the cranial nerves is important to avoid adverse effects on these structures adjacent to the lesion. The structures obtained from MR heavy T2 images were analyzed in three dimensions and fused with CT slices to evaluate fine structures in radiosurgery and microsurgery. In vascular lesions, angiography is critical for assessing the entire structure from shunt, blood flow, and bleeding risk factors. However, the angiogram did not show the exact parts of the brain and surrounding structures. True image fusion of axial plane angiography, MR images, and CT is ideal for precise target definition (Figure 5).
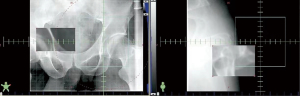
Discussion
As one of the main means of tumor treatment, radiation therapy is required by 70% of tumor patients at different stages of tumor disease (19). After one hundred years of development, radiotherapy equipment has incorporated deep therapy equipment from a single function, such as cobalt 60 development, to the mainstream of linear accelerator and mass child, heavy ion accelerator, and other advanced equipment. The technology has also advanced from the traditional two-dimensional radiotherapy evolution to modern three-dimensional four dimensions, even in the intensity modulation, image guided, stereotactic leap development (20). Modern radiotherapy is widely used in early-, middle-, locally-advanced, and even advanced tumor patients, the tumor effect is better, and the normal tissue damage is lighter, greatly improving the gain ratio of radiotherapy. The rapid development of radiotherapy technology has engendered a large number of images and treatment data (21). There is an urgent need for big data analysis to improve the quality of radiotherapy clinical decision-making, improve the work efficiency of all aspects of radiotherapy, and optimize the quality control of the whole process of radiotherapy (22). Therefore, the application of AI in the field of radiotherapy has emerged at the right moment. Modern radiotherapy is a highly complex treatment mode, including radiotherapy equipment, radiotherapy technology, radiotherapy processing, radiotherapy quality control, radiotherapy supervision and follow-up, and the complexity of radiotherapy safety protection (23).
Imaging and other biological indicators play an important role in the diagnosis and treatment of various tumors. With the development of radiotherapy technology, especially the extensive application of precise radiotherapy represented by intensity-modulated radiotherapy (IMRT) in clinical practice, it is increasingly required to sketch the curve of equal dose distribution (24-27). However, traditional anatomical imaging technology has its limitations in the selection and delineation of radiation fields and anatomical imaging combined with functional imaging technology (28). In particular, the application of some imaging agents that can reveal aspects of biological metabolism provides a tool for target delineation. It is common to use CT or PET in radiotherapy planning, but in the long run, the delineation of a target area should be combined with multiple imaging methods including CT, MRI, SPECT, PET, and so on, so as to more truly and comprehensively reflect the anatomical and functional metabolism status of tumor and normal tissues, and achieve dynamic observation (29). So far, tumor imaging has been dominated by CT. Currently, FDG-PET is the most mature and widely used functional imaging technology. It is believed that functional imaging will become an indispensable part of radiotherapy planning with its mature clinical application.
The high precision and speed of modern radiotherapy have higher requirements on the real-time of modern radiotherapy (30-32). In the era of image-guided radiotherapy, one of the major concerns is whether radiation can be “seen” in the target area, and can be adjusted according to the change of the target area. Due to technical limitations, CBCT or an electron field imaging system cannot realize the target movement and anatomical changes of patients during treatment. Therefore, the real-time monitoring system of targets, including adaptive radiotherapy, ExacTrac X-ray (Brainlab, Munich, Germany), 4D-CT and respiratory gating, came into being, which greatly improved the real-time performance of radiotherapy (33). Clinically, PET-CT is mainly used in tumor, brain and heart diseases, which can achieve early detection and diagnosis.
Taking tumors as an example, PET-CT can detect early tumors and metastatic lesions mainly because tumor cells have active metabolism and strong proliferation ability, and their metabolic level is significantly different from that of normal cells. In the process of examination, 18F-FDG tracer (imaging agent) is injected to make tumor cells emit obvious bright points under the instrument. With the naked eye, you can see tumor cells that are particularly metabolically active, and then you can find early tumors. Of course, in addition to malignant tumors, some inflammatory tissues, infection, tuberculosis focus, etc., there will also be metabolic exuberant bright spot, need to pay attention to the difference.
The functional expansion of modern radiotherapy is mainly reflected in the gradual application of functional imaging in the delineation of targets and the formulation of a radiotherapy plan. Although MRI/MRS can obtain physiological function imaging, CT can obtain anatomical structure, and PET can obtain tumor in vivo information by molecular metabolic imaging fusion technology, integrated imaging, structure, and in vivo information to delineate the active area of high metabolism in the target volume, which is called the biological target area. From this, new concepts and theories such as biological intensity modulated radiation therapy (B-IMRT) were derived (34). The B-IMRT is based on the biological target, which takes into account the sensitivity differences between the tumor and normal tissues, and the effects of these factors can be demonstrated by advanced imaging techniques, especially molecular imaging techniques (35). The core significance of the biological target area lies in the adjustment of the intensity of radiation in the biological target area, so that the tumor cells with active metabolism can receive a high dose of radiation and construct accurate dose control and engraving.
Acknowledgments
Funding: None.
Footnote
Reporting Checklist: The authors have completed the MDAR reporting checklist. Available at https://tcr.amegroups.com/article/view/10.21037/tcr-22-2005/rc
Data Sharing Statement: Available at https://tcr.amegroups.com/article/view/10.21037/tcr-22-2005/dss
Conflicts of Interest: All authors have completed the ICMJE uniform disclosure form (available at https://tcr.amegroups.com/article/view/10.21037/tcr-22-2005/coif). The authors have no conflicts of interest to declare.
Ethical Statement: The authors are accountable for all aspects of the work in ensuring that questions related to the accuracy or integrity of any part of the work are appropriately investigated and resolved. The study was conducted in accordance with the Declaration of Helsinki (as revised in 2013). The study was approved by ethics board of the First Affiliated Hospital of Xi’an Jiaotong University (No. 20220024) and individual consent for this retrospective analysis was waived
Open Access Statement: This is an Open Access article distributed in accordance with the Creative Commons Attribution-NonCommercial-NoDerivs 4.0 International License (CC BY-NC-ND 4.0), which permits the non-commercial replication and distribution of the article with the strict proviso that no changes or edits are made and the original work is properly cited (including links to both the formal publication through the relevant DOI and the license). See: https://creativecommons.org/licenses/by-nc-nd/4.0/.
References
- Xiao L, Xia XG, Wang YP. Exact and Robust Reconstructions of Integer Vectors Based on Multidimensional Chinese Remainder Theorem (MD-CRT). IEEE Trans Signal Process 2020;68:5349-64. [Crossref] [PubMed]
- Abshire D, Lang MK. The Evolution of Radiation Therapy in Treating Cancer. Semin Oncol Nurs 2018;34:151-7. [Crossref] [PubMed]
- Grewal AS, Jones J, Lin A. Palliative Radiation Therapy for Head and Neck Cancers. Int J Radiat Oncol Biol Phys 2019;105:254-66. [Crossref] [PubMed]
- Palmer JD, Gamez ME, Ranta K, et al. Radiation therapy strategies for skull-base malignancies. J Neurooncol 2020;150:445-62. [Crossref] [PubMed]
- Alfouzan AF. Radiation therapy in head and neck cancer. Saudi Med J 2021;42:247-54. [Crossref] [PubMed]
- Shah C, Bauer-Nilsen K, McNulty RH, et al. Novel radiation therapy approaches for breast cancer treatment. Semin Oncol 2020;47:209-16. [Crossref] [PubMed]
- Lee VH, Yang L, Jiang Y, et al. Radiation Therapy for Thoracic Malignancies. Hematol Oncol Clin North Am 2020;34:109-25. [Crossref] [PubMed]
- Opie C, Shwe KM. Improvements in radiation therapy services in Myanmar: 2012-2020. J Med Imaging Radiat Oncol 2021;65:445-53. [Crossref] [PubMed]
- Gardner SJ, Kim J, Chetty IJ. Modern Radiation Therapy Planning and Delivery. Hematol Oncol Clin North Am 2019;33:947-62. [Crossref] [PubMed]
- Reyngold M, Parikh P, Crane CH. Ablative radiation therapy for locally advanced pancreatic cancer: techniques and results. Radiat Oncol 2019;14:95. [Crossref] [PubMed]
- Glide-Hurst CK, Lee P, Yock AD, et al. Adaptive Radiation Therapy (ART) Strategies and Technical Considerations: A State of the ART Review From NRG Oncology. Int J Radiat Oncol Biol Phys 2021;109:1054-75. [Crossref] [PubMed]
- Freislederer P, Kügele M, Öllers M, et al. Recent advanced in Surface Guided Radiation Therapy. Radiat Oncol 2020;15:187. Erratum in: Radiat Oncol 2020;15:244. [Crossref] [PubMed]
- Hata M. Radiation therapy for elderly patients with uterine cervical cancer: feasibility of curative treatment. Int J Gynecol Cancer 2019;29:622-9. [Crossref] [PubMed]
- Reichstein DA, Brock AL. Radiation therapy for uveal melanoma: a review of treatment methods available in 2021. Curr Opin Ophthalmol 2021;32:183-90.
- Abi Jaoude J, Kouzy R, Nguyen ND, et al. Radiation therapy for patients with locally advanced pancreatic cancer: Evolving techniques and treatment strategies. Curr Probl Cancer 2020;44:100607. [Crossref] [PubMed]
- Liu J, Li J, Liu T, et al. Graded Image Generation Using Stratified CycleGAN. Med Image Comput Comput Assist Interv 2020;12262:760-9.
- Ji J, Zhong B, Ma KK. Image Interpolation Using Multi-scale Attention-aware Inception Network. Network. IEEE Trans Image Process 2020; Epub ahead of print. [Crossref] [PubMed]
- Broers N, Busch NA. The effect of intrinsic image memorability on recollection and familiarity. Mem Cognit 2021;49:998-1018. [Crossref] [PubMed]
- Taneja S, Barbee DL, Rea AJ, et al. CBCT image quality QA: Establishing a quantitative program. J Appl Clin Med Phys 2020;21:215-25. [Crossref] [PubMed]
- Yu N, Hu X, Song B, et al. Topic-Oriented Image Captioning Based on Order-Embedding. IEEE Trans Image Process 2019;28:2743-54. [Crossref] [PubMed]
- Zheng B, Zhang J, Sun G, et al. Fully Learnable Model for Task-Driven Image Compressed Sensing. Sensors (Basel) 2021;21:4662. [Crossref] [PubMed]
- Wang G, Shi Q, Shao Y, et al. DIBR-Synthesized Image Quality Assessment With Texture and Depth Information. Front Neurosci 2021;15:761610. [Crossref] [PubMed]
- Sharp J, Bouton D, Anabell L, et al. Image Distortion in Biplanar Slot Scanning: Part 2 Technology-specific Factors. J Pediatr Orthop 2020;40:587-91. [Crossref] [PubMed]
- Teng D, Xia S, Hu S, et al. miR-887-3p Inhibits the Progression of Colorectal Cancer via Downregulating DNMT1 Expression and Regulating P53 Expression. Comput Intell Neurosci 2022;2022:7179733. [Crossref] [PubMed]
- Chen Y, Wang J, Zhang X, et al. Correlation between apparent diffusion coefficient and pathological characteristics of patients with invasive breast cancer. Ann Transl Med 2021;9:143. [Crossref] [PubMed]
- Qi A, Li Y, Yan S, et al. Effect of postoperative chemotherapy on blood glucose and lipid metabolism in patients with invasive breast cancer. Gland Surg 2021;10:1470-7. [Crossref] [PubMed]
- Radomska KJ, Coulpier F, Gresset A, et al. Cellular Origin, Tumor Progression, and Pathogenic Mechanisms of Cutaneous Neurofibromas Revealed by Mice with Nf1 Knockout in Boundary Cap Cells. Cancer Discov 2019;9:130-47. [Crossref] [PubMed]
- Mo J, Anastasaki C, Chen Z, et al. Humanized neurofibroma model from induced pluripotent stem cells delineates tumor pathogenesis and developmental origins. J Clin Invest 2021;131:e139807. [Crossref] [PubMed]
- Goh GH, Petersson F. Plexiform Fibrohistiocytic Tumor Presenting as a Central Neck Mass Clinically Mimicking a Thyroglossal Duct Cyst: An Unusual Case Reported with Histo-cytopathologic Correlation and a Review of the Cytopathology Literature. Head Neck Pathol 2020;14:262-7. [Crossref] [PubMed]
- Iwata E, Orosz Z, Teh J, et al. Neuroendocrine Tumor Arising in a Tailgut Cyst: A Rare Presacral Tumor. Int J Surg Pathol 2019;27:336-42. [Crossref] [PubMed]
- Agarwal R, Sinha D, Tomar R, et al. Primary malignant peripheral nerve sheath tumor of the breast. Breast J 2019;25:742-5. [Crossref] [PubMed]
- Tritto V, Ferrari L, Esposito S, et al. Non-Coding RNA and Tumor Development in Neurofibromatosis Type 1: ANRIL Rs2151280 Is Associated with Optic Glioma Development and a Mild Phenotype in Neurofibromatosis Type 1 Patients. Genes (Basel) 2019;10:892. [Crossref] [PubMed]
- Yoshida K, Miyoshi T, Murakami T. Multicystic peritoneal tumor in two layer hens. J Vet Med Sci 2020;82:294-8. [Crossref] [PubMed]
- Winter N, Dohrn MF, Wittlinger J, et al. Role of high-resolution ultrasound in detection and monitoring of peripheral nerve tumor burden in neurofibromatosis in children. Childs Nerv Syst 2020;36:2427-32. [Crossref] [PubMed]
- Well L, Döbel K, Kluwe L, et al. Genotype-phenotype correlation in neurofibromatosis type-1: NF1 whole gene deletions lead to high tumor-burden and increased tumor-growth. PLoS Genet 2021;17:e1009517. [Crossref] [PubMed]
(English Language Editor: J. Jones)