Knockdown of Nrf2 radiosensitizes glioma cells by inducing redox stress and apoptosis in hypoxia
Introduction
Gliomas are the most frequent primary tumors in the central nervous system in adults, with an estimated annual incidence of 6.6 per 100,000 individuals in the USA (1,2). During the last two decades, the management of gliomas is always challenging and tough, mainly because of the unconstrained growth, the infiltrative invasion, the high recurrence rate, and the strong resistance to treatment (3). As to glioblastoma multiforme (GBM), standard therapy consists of maximal safe resection followed by concurrent temozolomide chemotherapy and radiation therapy (RT), and then adjuvant temozolomide, with an overall survival of 27.2% at 2 years (4). The intrinsic or acquired radioresistance is a major obstacle to a favorable prognosis (5). Further efforts are still required to progressively elucidate the mechanism of the RT resistance.
As the backbone of glioma therapy, RT uses high-energy radiation to kill residual cancer cells after resection, inducing DNA damage and cytotoxicity by generating reactive oxygen species (ROS). The generation and accumulation of ROS in turn causes oxidative stress, damaged biological macromolecules, and leads to eventual cell death (6). Nrf2, as the master mediator of cellular adaptation to redox stress, belongs to the Keap1-Nrf2-ARE (antioxidant response element) signaling pathway. It upregulates ARE-containing genes, such as HO-1, NQO1, GCL, and GST (7). Therefore, Nrf2 plays a pivotal role in cellular responses to oxidative stress, and could help protect cells in normal tissues from harmful stimuli, such as inflammation, trauma, and cancer (8-10). Besides, the latest findings showed that constitutively high levels of Nrf2 promoted cancer formation and cell proliferation, and contributed to chemoresistance (11,12). It was found that activation of Nrf2 protected against ionizing radiation toxicity and conferred radioresistance in human lung cancer cells (13,14). Further investigation indicated that Nrf2 activation in lung and liver tumor models was associated with decreased levels of ROS and oxidative DNA damage (15). Moreover, it has also been established that Nrf2 was involved in the proliferation, migration, invasion, apoptosis, and angiogenesis of glioma cells (16-18).
In this study, we aimed to elucidate the mechanism of Nrf2 in the RT resistance of glioma in hypoxia. Our findings demonstrated that ionizing radiation activated the Nrf2 antioxidant response, and downregulation of Nrf2 increased the radiosensitivity of glioma cells under mimicking hypoxic conditions. We presented the following article in accordance with the MDAR checklist (available at https://tcr.amegroups.com/article/view/10.21037/tcr-22-1420/rc).
Methods
Patients and tissue samples
A consecutive series of 6 recurrent malignant glioma patients admitted at Jinling Hospital from 2003 to 2009 were included in the study, according to the ethical and legal standards. There were no gender or ethnicity restrictions on the recruitment. Histological diagnosis and grading of the tumors were performed following WHO criteria (World Health Organization, 2007) and they stayed the same grade after the two surgeries. All patients had received resections twice, with the first one followed by only RT, abandoning chemotherapy before the second surgery. The adjuvant chemotherapy after the first resection was fully discussed with all the patients, yet they abandoned it.
Cell culture, plasmid transfection, and establishment of stable ARE-driven reporter and Nrf2-knockdown cells
U251 (RRID: CVCL_0021) and U87 (RRID: CVCL_0022) cell lines of human glioma were obtained from the Type Culture Collection of the Chinese Academy of Sciences in Shanghai, China. Cells were maintained in Dulbecco’s modified Eagle’s medium (DMEM) (Gibco, Los Angeles, CA) supplemented with 10% fetal bovine serum (FBS) (Gibco) and 1% penicillin/streptomycin (Gibco), and were incubated at 37 ℃ in a 5% CO2 incubator in normoxia, or in a hypoxic chamber containing 5% CO2 and 1% O2.
The ARE-luciferase reporter plasmids were generated using the GV260-6×ARE vector (Gene-Pharma, Shanghai, China), which contained the 5’LTR-6×ARE-Minimal promoter-luc2P-IRES-PuroR-PolyA-3’LTR structure. The reporter plasmids were synthesized into the lentiviral particles by Gene-Pharma and named as AREgv. The Nrf2-specific short hairpin RNA (shRNA) was constructed by Gene-Pharma with the target sequence as GCAGTTCAATGAAGCTCAACT, and the new plasmid was named Nrf2-inhibited (Nrf2i) (19). Random sequence, TTCTCCGAACGTGTCACGT, was used as the negative control, which was named NC.
Cell transfection was performed in 6-well plates with lentiviral particles using Polybrene (Gene-Pharma) according to the instruction of the manufacturer. Transfections were sustained for 24 hours and followed by 24 hours of recovery in the complete medium.
For the selection of stable cells with target plasmids, cells were grown in the medium containing 1.5 µg/mL puromycin (Sigma-Aldrich, St. Louis, MO, USA) for up to 2 weeks. The stable AREgv clones were isolated and screened by measuring their basal and inducible luciferase activities obtained by treatment with t-BHQ (Sigma-Aldrich) as described below.
ARE-Luciferase reporter cell assays
The ARE activation after RT was tested by assessing the luciferase activity. Firstly, U251-AREgv and U87-AREgv cells were plated at 2×103 cells/well in 96-well plates in 100 µL media and incubated overnight before RT in hypoxia. Luciferase activity was assessed by Dual-Luciferase® Reporter Assay System (Molecular Devices M3, CA, USA). Normalization of data was done by cell number.
Measurement of ROS generation
The generation of ROS in glioma cells was measured by a fluorescence determination kit (Keygen Biotech, Jiangsu, China) following the manufacturer’s protocol. Cells were incubated at 37 ℃ in hypoxia with 10 µM of DCFH-DA for 20 minutes. Adherent cells harvested via trypsinization were washed three times to remove the dye and placed on ice before Fluorescence-Activated Cell Sorter (FACS) analysis on a FACScalibur (Becton Dickinson, San Jose, CA, USA).
Cell viability assay
Cell viability assay was carried out using a cell counting kit-8 (CCK-8) (Dojindo, Kumamoto, Japan) according to the manufacturer’s protocol. Briefly, cells were plated at 1×103 cells/well in 96-well plates overnight, and irradiated with 4 Gy/day for 4 days (3 Gy/min, high-frequency linear accelerator, Elekta Precise, Stockholm, Sweden). After incubation for 3 hours, 10 µL CCK-8 was added and cells were further incubated for 1 h. Then the absorbance was measured at 450 nm using the ELISA microplate reader (Bio-Rad, California, USA).
Total RNA extraction and PCR analysis
Total RNA was isolated from cells using TRIzol reagent (Invitrogen, CA, USA) following the manufacturer’s recommendations, and subjected to DNase (Promega, Madison, WI, USA) treatment. Reverse transcriptase reactions were then performed by incubating 400 ng of total RNA with the First-strand cDNA synthesis kit (Takara, Dalian, China). Spectrophotometer analysis and agarose gel electrophoresis were used to assess total RNA concentration and purity. The cDNA was collected and amplified immediately by using the following primers: for Nrf2, 5'-TCAGCGACGGAAAGAGTATGA-3' and 5'-CCACTGGTTTCTGACTGGATGT-3'; for NQO1, 5'-ATGGTCGGCAGAAGAGC-3' and 5'-GGAAATGATGGGATTGAAGT-3'; for HO-1, 5'-TCTCCGATGGGTCCTTACACTC-3' and 5'-GGCATAAAGCCCTACAGCAACT-3'; and for GAPDH, 5'-AGATCCCTCCAAAATCAAGTGG-3' and 5'-GGCAGAGATGATGACCCTTTT-3'. The amplification and data acquisition were implemented on a quantitative real-time polymerase chain reaction (PCR) system (Agilent, USA) using FastStart universal SYBR green master (Roche, Mannheim, Germany). Conditions were preset at 95 ℃ for 10 min, followed by 40 circles at 95 ℃ for 15 s and 60 ℃ for 1 min. Relative quantification of mRNA expression was determined using the 2−△△CT method. PCR amplification efficiency of each gene was established utilizing calibration curves. GAPDH was used as the internal reference gene. All the real-time PCR experiments were performed in accordance with the Minimum Information for publication of Quantitative Real-time PCR Experiments (20).
Western blot analysis
Cell lysates were prepared by extracting proteins with RIPA buffer centrifuged at 12,000 g for 15 min at 4 ℃. The concentration of proteins was measured by Coomassie Plus Protein Assay Reagent (Pierce, Illinois, USA). Total protein was separated by 10% sodium dodecyl sulfate-polyacrylamide gels electrophoresis using the Criterion System (Bio-Rad) and transferred to a nitrocellulose membrane (Millipore, Billerica, MA, USA). For immunoblotting, membranes were blocked with 5% skim milk for 2 hours at room temperature, and the following antibodies were used: 1:500 anti-Nrf2 (Abcam Cat#2178-1, RRID: AB_991780) and 1:1,000 anti-β-actin (Santa Cruz Biotechnology Cat#sc-517582, RRID: AB_2833259). After washing, membranes were incubated with secondary antibodies (Cell Signaling Technology Cat#7074, RRID: AB_2099233; Cat#7076, RRID: AB_330924) for 2 hours at room temperature. Protein bands were visualized with Chemiluminescent HRP Substrate (Millipore) and exposed to X-ray film (Fujifilm, Tokyo, Japan). Relative changes in protein expression were estimated from the mean pixel density and normalized to β-actin.
Measurement of total glutathione (GSH) contents
Glioma cells were grown in six-well plates for 24 hours, collected and lysed with protein detergent S solvent. The total GSH was determined by a commercially available Total Glutathione Assay Kit (Beyotime Institute of Biotechnology). All procedures complied with the manufacturer’s instructions. Protein concentration was estimated by Coomassie Plus Protein Assay Reagent (Pierce).
Immunohistochemical staining
For immunohistochemical analysis of the glioma tissues, 3-µm-thick serial sections were dewaxed and endogenous peroxidase was quenched with 3% H2O2 in methanol for 30 min. Before staining, nonspecific binding was blocked by incubation with 10% bovine serum albumin (BSA) in PBS at 37 ℃ for 1 h. Then all incubations with 1:50 anti-Nrf2 (Abcam Cat#2178-1, RRID: AB_991780) or 1:100 anti-HIF-1α (Novus Cat# NB100-105B, RRID: AB_1111438) antibody in PBS containing 1% BSA were carried out at 4 ℃ overnight (19). Sections were briefly washed and incubated at room temperature with the anti-rabbit antibody and avidin-biotin-peroxidase (Vector Laboratories Inc., Burlingame, CA, USA). The color was then developed by incubation with the diaminobenzidine solution (Dako Corporation, Carpinteria, CA, USA). Meyer’s hematoxylin (Sigma-Aldrich) was used to counterstain the nuclei. The hematoxylin and eosin (HE) staining was performed to identify the histological profiles of tumor cells. Occupied regions by tumor cells (percentage over trimmed tumor mass) were counted in the HE staining using Image J. The number of positive-staining cells was calculated from six representative staining fields per specimen under 200× magnifications. And the immunohistochemistry positive results for Nrf2 were recorded as previously described (19). Final immunoreactivity scores (IRS) were obtained by multiplying the percentage and the intensity score, and the mean value of the six fields was taken as the final result for each specimen.
Cell apoptosis analysis
Cell apoptosis was assessed by using a FITC-Annexin V apoptosis kit (Beyotime Institute of Biotechnology). Briefly, cells were harvested at a density of 5×105 cells/mL and then incubated with Annexin V-FITC and Propidium (PI) in dark for 15 min. At last, early and late apoptosis were detected on the flow cytometer (Becton Dickinson).
Ionizing radiation
Glioma cells were irradiated at room temperature in RS-2000 Pro Biological Irradiator (Resource, Buford, GA) at a dose rate of 2.13 Gy/min (maximum energy of 160 kV and 25 mA).
Statistical analysis
All the experiments were biologically repeated in triplicates independently with statistical data presented as mean ± SD. GraphPad Prism 8.3.0 was applied for statistical analysis. One-way ANOVA followed by Tukey’s multiple comparisons test was used to compare the levels of more than two groups. Data of two groups in Figure 1 were analyzed by paired, two-tailed Student’s t-test. P value <0.05 was considered statistically significant.
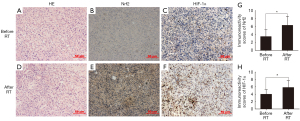
Ethical statement
The study was conducted in accordance with the Declaration of Helsinki (as revised in 2013). The study was approved by the Ethics Committee of Jinling Hospital (ID: 2016NZGKJ-029) and informed consent was taken from all the patients.
Results
High expression of Nrf2 in human malignant glioma tissues after RT
The protein levels of Nrf2 and HIF-1α were detected by immunohistochemical analysis in malignant glioma samples (with clinical information shown in Table S1) before and after RT. It was suggested that the staining intensity of Nrf2 was estimated mainly as weak or moderate and predominantly located in the cytoplasm in glioma cells before RT (Figure 1B). While the expression of Nrf2 was increased in the glioma tissues after exposure to irradiation (P=0.0144, Figure 1E,1G). Furthermore, Nrf2 immunoreactivity seemed to be also positive in the nuclei after RT (Figure 1E). As the main mediator of hypoxia, HIF-1α was mainly detected in the nuclei before RT (Figure 1C) and elevated after RT (P=0.0379, Figure 1F,1H). In brief, Nrf2 expression was enhanced significantly after exposure to RT in human recurrent glioma tissues with hypoxic conditions.
Irradiation increased ARE-reporter activity after 4 days, instead of within 24 hours in hypoxia
Inspired by the clinical phenomenon of Nrf2 activation, we constructed the ARE-luciferase reporter cell lines, U251-AREgv and U87-AREgv, to determine whether exposure to varying doses of ionizing radiation would activate the Nrf2-ARE pathway. The Nrf2 inducer, tert-butylhydroquinone (5 to 100 µM tBHQ), was used to confirm that the ARE reporter gene was functional, which showed a dose- and time-dependent increase in reporter activity by 24 hours (data not shown). Yet there was no significant increase in ARE luciferase after irradiation in the range 1 to 8 Gy at varying times from 2 to 24 hours, in the normoxia or the 1% O2 hypoxic condition (data not shown). Next, we found that ARE was activated by dose-dependent irradiation in the range from 2 to 8 Gy when assessed at about 4 days in both U251 and U87 cell lines (Figure 2A), which showed a delayed response to single doses. Then the following experiments were all carried out in the 1% O2 hypoxia. Clinically, RT doses are conventionally fractionated into a daily regimen. Therefore, fractionated irradiation was delivered, and ARE was activated by at least 4 daily fractions from 1 to 4 Gy per day, which showed a dose-dependent increase in luciferase activity too (Figure 2B). In both U251 and U87 cells, the protein level of Nrf2 was heightened effectively by a single dose of 8 Gy, as well as 4 daily fractions of 4 Gy (Figure 2C), which confirmed the ARE-reporter activation. Furthermore, the mRNA levels of Nrf2, and its representative downstream genes, HO-1 and NQO1, were significantly increased by the two irradiation modes mentioned above (Figure 2D,2E). The increase of these ARE-driven genes and protein expression indicated that irradiation induced a delayed activation of the ARE-Nrf2 pathway in hypoxia.
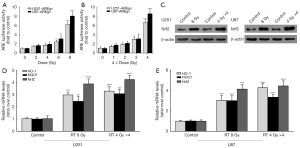
Establishment of stable Nrf2-knockdown cell lines
To establish the stable Nrf2 knockdown cells, we transfected the glioma cells with lentiviral-mediating plasmids encoding Nrf2-targeting shRNA, and then screened out the stable lines with puromycin. The down expression of Nrf2 was verified at both mRNA (both P<0.0001, Figure 3A and 3B) and protein (Figure 3C) levels in U251 and U87 cell lines. Furthermore, the mRNA levels of Nrf2 downstream genes, HO-1 and NQO1, were greatly depressed in the Nrf2i group of U251 and U87, compared with the wildtype (WT) and NC group (all P<0.01, Figure 3A,3B). And also, the function of Nrf2 was assessed by GSH content, which accorded with the reduced Nrf2 levels in both cell lines (P=0.0006 and 0.0003, for U251 and U87, respectively) (Figure 3D,3E).
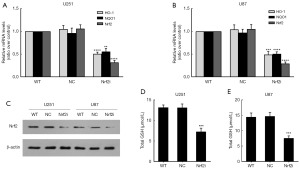
Downregulation of Nrf2 enhanced the ROS formation after RT in hypoxia
Afterward, the WT, NC, and stable Nrf2i groups of U251 and U87 cells were all exposed to 4 daily fractions of 4 Gy irradiation in hypoxia. It was suggested that the relative mRNA levels of HO-1 and NQO1 were highly elevated in the WT and NC group after RT (all P<0.01), yet depressed in the Nrf2i group after RT (all P<0.001) in U251 (Figure 4A) and U87 (Figure 4B). As Nrf2 pathway was known to play a pivotal role in oxidative stress and control the basal redox levels in cancer cells (21), the ROS levels were then examined in the stable knockdown cells with or without RT by FACS analysis in our study. It was suggested that the ROS level was higher in Nrf2i U87 and U251 cells (both P<0.05, Figure 4C,4D). Groups exposed to irradiation showed a dramatic increase in ROS in hypoxia. Specifically, induced by RT, the Nrf2i group also had a significantly higher level of ROS (all P<0.0001, Figure 4C,4D).
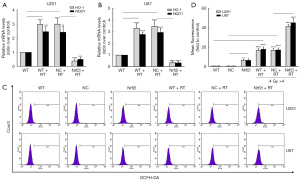
Knockdown of Nrf2 enhanced the RT effects on glioma cells in hypoxia
It is known that the high level of ROS results in significant damage to cell structures, including the glioma cells in hypoxia. Logically, we detected the apoptosis of U251 and U87 cells induced by irradiation in the FACS analysis, and lastly, assessed the cell viability by the CCK-8 assay. As expected, the apoptotic cells in the WT and NC group were significantly increased when exposed to 4 daily fractions of 4 Gy irradiation in hypoxia. While the stable knockdown of Nrf2 triggered much more apoptosis (both P<0.0001, Figure 5A,5B) and lower cell viability (P=0.0004 and 0.0058, for U251 and U87, respectively, Figure 5C) after RT. In a word, it was systematically verified that the RT effects were greatly enhanced by the knockdown of Nrf2 in glioma cells in hypoxia.
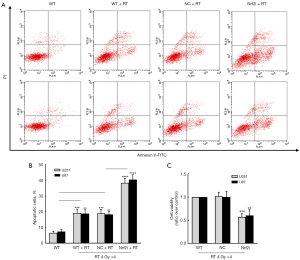
Discussion
As the intrinsic property of solid tumors, the importance of hypoxia in tumor progression and radioresistance has been valued (22). Hypoxia is considered as one of the microenvironmental factors associated with the malignant nature of glioma. In our previous study, the hypoxic conditions had been mimicked successfully by incubating cells in the hypoxic chamber containing 5% CO2 and 1% O2 (19). And it was demonstrated that Nrf2 could regulate glioma angiogenesis by imposing a blockade to HIF-1α-VEGF signaling in hypoxia. Likewise, we replicated the hypoxia model in vitro to mimic the hypoxic microenvironment of glioma throughout this study.
Recently, emerging studies have reported the deleterious effects of the Nrf2 signal in cancer cell biology, a high level of which promoted cancer formation and contributed to the chemoresistance of cancer cells (11,12,23). In our preliminary studies, Nrf2 has been proven to participate in the regulation of proliferation, apoptosis, migration, invasion, autophagy, and angiogenesis in glioma cells in hypoxia (16-19,24). It was observed that mutations were identified in the DGR or Kelch domain of the Keap1 protein and the DLG and ETGE motifs of Nrf2 in lung cancer cells, and Nrf2 was thus activated, eventually leading to the constitutive overexpressed Nrf2 and drug resistance (25-28). It was further found that Nrf2 could affect the prognosis of patients with lung cancers by inducing an elevated stress response to oxidative damage (29). A further study indicated that ionizing radiation activated ARE-dependent transcription in breast cancer cells, and the Nrf2-ARE pathway was important in maintaining resistance to irradiation under specific circumstances (30). Moreover, it was preliminarily shown that Nrf2 might enhance the resistivity to radiotherapy of specific human malignant cells including glioma (31-33). These findings led to the hypothesis that the antioxidant role of Nrf2 would be very important in the radioresistance of glioma cells. However, the role of Nrf2 in tumor radiosensitivity has not been fully understood and the mechanism behind remains unclear. So, our study further investigated the function of Nrf2 in the radiosensitivity of glioma cells, especially under hypoxic conditions. U87 and U251 were used in vitro in this study, as the representatives of GBM cells, and the results should be further verified in the patient-derived glioma stem cell lines.
It is known that irradiation could drive the expression of gene transcriptional programs, such as HO-1, a target gene of Nrf2 (34). Nrf2/HO-1 system was reported to protect against oxidative damage, modulation of apoptosis, regulation of inflammation, and contribution to angiogenesis, as an evolutionarily conserved mechanism (35). Several studies have also shown that overexpression of HO-1 is involved in the resistance of brain tumors to both chemotherapy and radiotherapy (36). It was reported that marked HO-1 expression was induced in pancreatic cancer cells after RT, and the targeted knockdown of HO-1 expression increased the radiosensitivity of cancer cells (34). Another report found that low doses of gamma-radiation induced nuclear accumulation of Nrf2 by 4 hours in the mouse macrophage cell line, probably through ERK1/2-dependent pathways (37). These results suggest that the negative effect of HO-1 in radiotherapy exists ubiquitously in tumors, but whether it also happens in GBM remains validated.
NQO1, a member of the NAD(P)H dehydrogenase (quinone) family, is another down-stream regulated gene of Nrf2. It reduces quinones to hydroquinones and results in the production of radical species. It is reported that the expression of NQO1 is enhanced in GBM (38). Overexpression of NQO1 also reduces ROS and increases cell proliferation in GBM (39). Furthermore, the NQO1 expression determines the radiosensitivity of triple-negative breast cancer cells (40). Yet it is still unknown whether NQO1 could regulate radiosensitivity in GBM.
While in this study, we have shown that both HO-1 and NQO1 are augmented after RT and could be positively regulated by Nrf2 in glioma cells. Also, reduction of Nrf2 enhanced the radiosensitivity of GBM cells by downregulation of HO-1 and NQO1. Summarily, our study suggested that HO-1 and NQO1 could be targets for ameliorating radiosensitivity of glioma cells, as the downstream molecules of Nrf2.
What’s more, irradiation was reported to induce a delayed activation of Nrf2 in multiple disease models, which may be cell type-dependent. In an oral mucositis model induced by fractionated irradiation, 19 days were taken to observe the increased Nrf2 expression (41). Similarly, Haton et al. found that irradiation-induced antioxidants, MnSOD and thioredoxin 2, expressed in the gut by 6 hours after abdominal exposure, waned by 4 days, and then a second level of induced antioxidant genes were expressed (42). It was also reported very recently that ionizing radiation can activate Nrf2 to increase ARE-dependent gene expression in breast cancer cells, but only after a significant time delay (30). This might be explained by the dynamic wound healing process, evolving from inflammation to tissue repair. It represented a transition from a pro-oxidant and pro-inflammatory phase to an antioxidant and anti-inflammatory phase with an appropriate delay, in which the Nrf2 pathway may participate. However, the protective process could restrict the radiotherapy effect of killing tumor cells.
In the previous studies, we have found that temozolomide and irradiation combined treatment could induce Nrf2 activation, which decreased chemoradiation sensitivity in human glioblastoma cells (43). In the current study, we put our eyes on the effect of RT on Nrf2 activation in human malignant glioma tissues. It was found that the IRS were increased and nuclear localization of Nrf2 was enhanced in the corresponding recurrent tumor tissues from the same patients undergoing RT. These evidences indicated the activation of the Nrf2-ARE pathway and implied the significance of Nrf2 in the radioresistance of glioma. Then, the vitro experiments indicated that it took 4 days to induce the delayed ARE-Nrf2 pathway activation after radiotherapy in hypoxia in glioma, which was radiation dose- and time-dependent and correlated with deferred ROS production in hypoxia. Furthermore, ROS production could climb continuously when Nrf2 is knocked down, which led to furthermore death of tumor cells through apoptosis. All of these suggested that the activated products of the Nrf2 pathway buffered redox changes generated by irradiation adequately. With Nrf2 knocked down, ROS could be produced steadily, leading to a stronger lethal effect on glioma.
Conclusions
In a word, this research found that activated Nrf2 might be an important factor for the radioresistance of tumor cells by protecting them from radiation-induced oxidative stress. Downregulation of Nrf2 could sensitize the lethal effect on GBM cells in vitro by enhancing oxidative stress and apoptosis in hypoxia, verifying the function of Nrf2, as an important target of radiotherapy in glioma.
Acknowledgments
The authors are grateful to the Translational Medicine Core Facilities in Medical School of Nanjing University. We would like to thank Professor Brad Smith for his help in polishing our paper.
Funding: This article was supported by
Footnote
Reporting Checklist: The authors have completed the MDAR checklist. Available at https://tcr.amegroups.com/article/view/10.21037/tcr-22-1420/rc
Data Sharing Statement: Available at https://tcr.amegroups.com/article/view/10.21037/tcr-22-1420/dss
Conflicts of Interest: All authors have completed the ICMJE uniform disclosure form (available at https://tcr.amegroups.com/article/view/10.21037/tcr-22-1420/coif). YJ reports grant (No. 81802513) from the National Natural Science Foundation of China. HDW reports Grant (No. 81672503) from the National Natural Science Foundation of China. XJJ reports Grant (No. BK20140731) from the Natural Science Foundation of Jiangsu Province. The other authors have no conflicts of interest to declare.
Ethical Statement: The authors are accountable for all aspects of the work in ensuring that questions related to the accuracy or integrity of any part of the work are appropriately investigated and resolved. The study was conducted in accordance with the Declaration of Helsinki (as revised in 2013). The study was approved by the Ethics Committee of Jinling Hospital (ID: 2016NZGKJ-029) and informed consent was taken from all the patients.
Open Access Statement: This is an Open Access article distributed in accordance with the Creative Commons Attribution-NonCommercial-NoDerivs 4.0 International License (CC BY-NC-ND 4.0), which permits the non-commercial replication and distribution of the article with the strict proviso that no changes or edits are made and the original work is properly cited (including links to both the formal publication through the relevant DOI and the license). See: https://creativecommons.org/licenses/by-nc-nd/4.0/.
References
- Wen PY, Kesari S. Malignant gliomas in adults. N Engl J Med 2008;359:492-507. [Crossref] [PubMed]
- Ostrom QT, Gittleman H, Fulop J, et al. CBTRUS Statistical Report: Primary Brain and Central Nervous System Tumors Diagnosed in the United States in 2008-2012. Neuro Oncol 2015;17:iv1-iv62. [Crossref] [PubMed]
- Reifenberger G, Wirsching HG, Knobbe-Thomsen CB, et al. Advances in the molecular genetics of gliomas - implications for classification and therapy. Nat Rev Clin Oncol 2017;14:434-52. [Crossref] [PubMed]
- Stupp R, Hegi ME, Mason WP, et al. Effects of radiotherapy with concomitant and adjuvant temozolomide versus radiotherapy alone on survival in glioblastoma in a randomised phase III study: 5-year analysis of the EORTC-NCIC trial. Lancet Oncol 2009;10:459-66. [Crossref] [PubMed]
- Meng W, Palmer JD, Siedow M, et al. Overcoming Radiation Resistance in Gliomas by Targeting Metabolism and DNA Repair Pathways. Int J Mol Sci 2022;23:2246. [Crossref] [PubMed]
- Hensley K, Floyd RA. Reactive oxygen species and protein oxidation in aging: a look back, a look ahead. Arch Biochem Biophys 2002;397:377-83. [Crossref] [PubMed]
- Shahcheraghi SH, Salemi F, Alam W, et al. The Role of NRF2/KEAP1 Pathway in Glioblastoma: Pharmacological Implications. Med Oncol 2022;39:91. [Crossref] [PubMed]
- Yan W, Wang HD, Feng XM, et al. The expression of NF-E2-related factor 2 in the rat brain after traumatic brain injury. J Trauma 2009;66:1431-5. [Crossref] [PubMed]
- Dinkova-Kostova AT, Liby KT, Stephenson KK, et al. Extremely potent triterpenoid inducers of the phase 2 response: correlations of protection against oxidant and inflammatory stress. Proc Natl Acad Sci U S A 2005;102:4584-9. [Crossref] [PubMed]
- Kwak MK, Itoh K, Yamamoto M, et al. Role of transcription factor Nrf2 in the induction of hepatic phase 2 and antioxidative enzymes in vivo by the cancer chemoprotective agent, 3H-1, 2-dimethiole-3-thione. Mol Med 2001;7:135-45. [Crossref] [PubMed]
- Yamadori T, Ishii Y, Homma S, et al. Molecular mechanisms for the regulation of Nrf2-mediated cell proliferation in non-small-cell lung cancers. Oncogene 2012;31:4768-77. [Crossref] [PubMed]
- Homma S, Ishii Y, Morishima Y, et al. Nrf2 enhances cell proliferation and resistance to anticancer drugs in human lung cancer. Clin Cancer Res 2009;15:3423-32. [Crossref] [PubMed]
- Lee S, Lim MJ, Kim MH, et al. An effective strategy for increasing the radiosensitivity of Human lung Cancer cells by blocking Nrf2-dependent antioxidant responses. Free Radic Biol Med 2012;53:807-16. [Crossref] [PubMed]
- Singh A, Bodas M, Wakabayashi N, et al. Gain of Nrf2 function in non-small-cell lung cancer cells confers radioresistance. Antioxid Redox Signal 2010;13:1627-37. [Crossref] [PubMed]
- Harris IS, DeNicola GM. The Complex Interplay between Antioxidants and ROS in Cancer. Trends Cell Biol 2020;30:440-51. [Crossref] [PubMed]
- Pan H, Wang H, Zhu L, et al. The involvement of Nrf2-ARE pathway in regulation of apoptosis in human glioblastoma cell U251. Neurol Res 2013;35:71-8. [Crossref] [PubMed]
- Ji XJ, Chen SH, Zhu L, et al. Knockdown of NF-E2-related factor 2 inhibits the proliferation and growth of U251MG human glioma cells in a mouse xenograft model. Oncol Rep 2013;30:157-64. [Crossref] [PubMed]
- Pan H, Wang H, Zhu L, et al. The role of Nrf2 in migration and invasion of human glioma cell U251. World Neurosurg 2013;80:363-70. [Crossref] [PubMed]
- Ji X, Wang H, Zhu J, et al. Knockdown of Nrf2 suppresses glioblastoma angiogenesis by inhibiting hypoxia-induced activation of HIF-1α. Int J Cancer 2014;135:574-84. [Crossref] [PubMed]
- Bustin SA, Benes V, Garson JA, et al. The MIQE guidelines: minimum information for publication of quantitative real-time PCR experiments. Clin Chem 2009;55:611-22. [Crossref] [PubMed]
- Menegon S, Columbano A, Giordano S. The Dual Roles of NRF2 in Cancer. Trends Mol Med 2016;22:578-93. [Crossref] [PubMed]
- Chan DA, Giaccia AJ. Hypoxia, gene expression, and metastasis. Cancer Metastasis Rev 2007;26:333-9. [Crossref] [PubMed]
- Tian Y, Wu K, Liu Q, et al. Modification of platinum sensitivity by KEAP1/NRF2 signals in non-small cell lung cancer. J Hematol Oncol 2016;9:83. [Crossref] [PubMed]
- Zhou Y, Wang HD, Zhu L, et al. Knockdown of Nrf2 enhances autophagy induced by temozolomide in U251 human glioma cell line. Oncol Rep 2013;29:394-400. [Crossref] [PubMed]
- Singh A, Misra V, Thimmulappa RK, et al. Dysfunctional KEAP1-NRF2 interaction in non-small-cell lung cancer. PLoS Med 2006;3:e420. [Crossref] [PubMed]
- Konstantinopoulos PA, Spentzos D, Fountzilas E, et al. Keap1 mutations and Nrf2 pathway activation in epithelial ovarian cancer. Cancer Res 2011;71:5081-9. [Crossref] [PubMed]
- Jeong Y, Hoang NT, Lovejoy A, et al. Role of KEAP1/NRF2 and TP53 Mutations in Lung Squamous Cell Carcinoma Development and Radiation Resistance. Cancer Discov 2017;7:86-101. [Crossref] [PubMed]
- Padmanabhan B, Tong KI, Ohta T, et al. Structural basis for defects of Keap1 activity provoked by its point mutations in lung cancer. Mol Cell 2006;21:689-700. [Crossref] [PubMed]
- Merikallio H, Pääkkö P, Kinnula VL, et al. Nuclear factor erythroid-derived 2-like 2 (Nrf2) and DJ1 are prognostic factors in lung cancer. Hum Pathol 2012;43:577-84. [Crossref] [PubMed]
- McDonald JT, Kim K, Norris AJ, et al. Ionizing radiation activates the Nrf2 antioxidant response. Cancer Res 2010;70:8886-95. [Crossref] [PubMed]
- Sharma PK, Varshney R. 2-Deoxy-D-glucose and 6-aminonicotinamide-mediated Nrf2 down regulation leads to radiosensitization of malignant cells via abrogation of GSH-mediated defense. Free Radic Res 2012;46:1446-57. [Crossref] [PubMed]
- Da C, Pu J, Liu Z, et al. HACE1-mediated NRF2 activation causes enhanced malignant phenotypes and decreased radiosensitivity of glioma cells. Signal Transduct Target Ther 2021;6:399. [Crossref] [PubMed]
- Yang W, Shen Y, Wei J, et al. MicroRNA-153/Nrf-2/GPx1 pathway regulates radiosensitivity and stemness of glioma stem cells via reactive oxygen species. Oncotarget 2015;6:22006-27. [Crossref] [PubMed]
- Berberat PO, Dambrauskas Z, Gulbinas A, et al. Inhibition of heme oxygenase-1 increases responsiveness of pancreatic cancer cells to anticancer treatment. Clin Cancer Res 2005;11:3790-8. [Crossref] [PubMed]
- Loboda A, Damulewicz M, Pyza E, et al. Role of Nrf2/HO-1 system in development, oxidative stress response and diseases: an evolutionarily conserved mechanism. Cell Mol Life Sci 2016;73:3221-47. [Crossref] [PubMed]
- Sferrazzo G, Di Rosa M, Barone E, et al. Heme Oxygenase-1 in Central Nervous System Malignancies. J Clin Med 2020;9:1562. [Crossref] [PubMed]
- Tsukimoto M, Tamaishi N, Homma T, et al. Low-dose gamma-ray irradiation induces translocation of Nrf2 into nuclear in mouse macrophage RAW264.7 cells. J Radiat Res 2010;51:349-53. [Crossref] [PubMed]
- Zhong B, Yu J, Hou Y, et al. A novel strategy for glioblastoma treatment by induction of noptosis, an NQO1-dependent necrosis. Free Radic Biol Med 2021;166:104-15. [Crossref] [PubMed]
- Luo S, Lei K, Xiang D, et al. NQO1 Is Regulated by PTEN in Glioblastoma, Mediating Cell Proliferation and Oxidative Stress. Oxid Med Cell Longev 2018;2018:9146528. [Crossref] [PubMed]
- Lin LC, Lee HT, Chien PJ, et al. NAD(P)H:quinone oxidoreductase 1 determines radiosensitivity of triple negative breast cancer cells and is controlled by long non-coding RNA NEAT1. Int J Med Sci 2020;17:2214-24. [Crossref] [PubMed]
- Ara G, Watkins BA, Zhong H, et al. Velafermin (rhFGF-20) reduces the severity and duration of hamster cheek pouch mucositis induced by fractionated radiation. Int J Radiat Biol 2008;84:401-12. [Crossref] [PubMed]
- Haton C, François A, Vandamme M, et al. Imbalance of the antioxidant network of mouse small intestinal mucosa after radiation exposure. Radiat Res 2007;167:445-53. [Crossref] [PubMed]
- Cong ZX, Wang HD, Zhou Y, et al. Temozolomide and irradiation combined treatment-induced Nrf2 activation increases chemoradiation sensitivity in human glioblastoma cells. J Neurooncol 2014;116:41-8. [Crossref] [PubMed]