Research on the oncogenic role of the house-keeping gene GAPDH in human tumors
Highlight box
Key findings
• GAPDH may be a promising biomarker for pan-cancer diagnosis.
What is known and what is new?
• Normally, GAPDH is used as an internal reference gene to quantify DNA, RNA, and proteins, but it is unstable at some pathological stages, including cancer and aging;
• GAPDH overexpression is found in the majority of TCGA tumors. High levels of GAPDH are associated with poor survival.
What is the implication, and what should change now?
• GAPDH has a potential role in cancer progression in human beings. RNA or protein analyses using GAPDH are not compatible with most cancer research.
Introduction
There is an increasing demand for new pan-cancer markers in order to better understand the mechanism and clinical application of tumorigenesis process. Many publicly funded bioinformatics databases, like The Cancer Genome Atlas (TCGA), could provide an outstanding convenience for people to analyze the expression and related functional genomics data of the interested gene in a variety of cancers (1-3).
Glyceraldehyde-3-phosphate dehydrogenase (GAPDH), which plays a role in glycolysis, has been identified as an internal reference gene to quantitate DNA, RNA and proteins in usual biological experiments (4,5). An important reason is that GAPDH is highly and constantly expressed in almost all tissues. However, A study of GAPDH protein in mouse had discovered a new side (6). It might take part in several additional nuclear functions such as nitrosylation of nuclear proteins and the regulation of mRNA stability due to its nitrosylase activity. Subsequently, more and more studies have found that GAPDH is unstable in some pathological stages including aging and cancer (7,8). As a glycolytic enzyme, GAPDH is a significant player in cancer energy metabolism to reflect the high metabolic state of cancer, which may be further related to cancer proliferation and invasion.
Therefore, we would carry out the pan-cancer analysis to explore the expression profile of GAPDH and several other modules including survival status, gene regulation and related cellular pathways in various tumor types through the datasets provided by TCGA project and corresponding databases. This comprehensive work would be helpful for us to reveal the potential application value and molecular mechanism of GAPDH in the progression of cancers in human beings. We present the following article in accordance with the REMARK reporting checklist (available at https://tcr.amegroups.com/article/view/10.21037/tcr-22-1972/rc).
Methods
Analysis of GAPDH gene expression
The study was conducted in accordance with the Declaration of Helsinki (as revised in 2013).
Tumor IMmune Estimation Resource 2 (TIMER2) database (http://timer.cistrome.org/) was used to compare an expression of GAPDH mRNA in tumors and nearby normal tissues across all tumors from TCGA (9).
Gene Expression Profiling Interactive Analysis 2 (GEPIA2) database (http://gepia2.cancer-pku.cn) was used to profile the expression of GAPDH mRNA between tumors and matched TCGA normal and Genotype-Tissue Expression (GTEx) data (10).
University of ALabama at Birmingham CANcer (UALCAN) database (http://ualcan.path.uab.edu/analysis-prot.html) was used to collect the expression of GAPDH protein in primary and normal tissues using data from Clinical Proteomic Tumor Analysis Consortium (CPTAC) dataset (11).
The Human Protein Atlas (HPA) database (http://www.proteinatlas.org/) was used to download the immunohistochemistry (IHC) images of GAPDH in normal tissues and four tumors, including ovarian serous cystadenocarcinoma (OV), kidney renal clear cell carcinoma (KIRC), lung adenocarcinoma (LUAD) and pancreatic adenocarcinoma (PAAD).
Analyses of survival prospects
The above GEPIA2 database was used to get the information about the overall survival (OS) data, disease-free survival (DFS) data and survival map of GAPDH throng TCGA tumors.
Analyses of genetic changes
The cBioPortal database (https://www.cbioportal.org/) was used to obtain the genetic alteration data of GAPDH in tumors, consisting of gene mutation, change frequency, mRNA expression and so on (12).
Analyzing immune infiltration
We analyzed TIMER2 data to determine if GAPDH expression correlates with immune infiltration in TCGA tumors. Fibroblasts, neutrophils and endothelial cells associated with cancer were selected for further analysis.
Analyses of genes related to GAPDH gene
Search Tool for Recurring Instances of Neighbouring Genes (STRING) website (https://string-db.org/) was used to collect no more than 50 interactors of GAPDH protein based on experiments (13).
The GEPIA2 database was again used to collect the top 100 GAPDH-correlated genes across all the TCGA tumors. For a few genes of interest, we conducted a paired Pearson correlation analysis to GAPDH.
The R language software (Clusterprofiler package, version 3.14.3) was used to conduct the enriched Gene Ontology (GO) and Kyoto Encyclopedia of Genes and Genomes (KEGG) pathway of the above two sets of GAPDH binding and interacted genes.
Statistical analysis
We used Spearman correlation analysis as the statistical approach. And P<0.05 was considered meaningful.
Results
GAPDH gene expression increases in the majority of TCGA tumors
Using the TIMER2, we compared GAPDH expression in the TCGA repository among tumors and normal tissues adjacent to them. Figure 1A shows that GAPDH is expressed at a higher level in almost all tumor tissues like bladder urothelial carcinoma (BLCA) and lung squamous cell carcinoma (LUSC) than in tissues that serve as controls. Incredibly, only one tumor type, prostate adenocarcinoma (PRAD), did not exhibit differential expression.
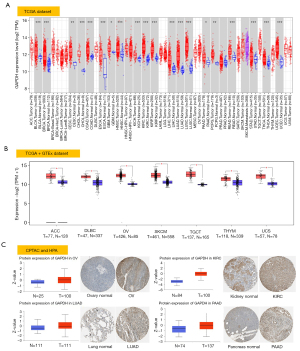
In cases in which the TCGA does not have any data on normal tissues, further analysis of GAPDH expression was conducted in tumors using GTEx data. For adrenocortical carcinoma (ACC), lymphoid neoplasm diffuse large B-cell lymphoma (DLBC), OV, skin cutaneous melanoma (SKCM), testicular germ cell tumors (TGCT), thymoma (THYM) and uterine carcinosarcoma (UCS), we found higher expression in tumor tissues (Figure 1B, P<0.05). Tumors like acute myeloid leukemia (LAML) or Brain lower grade glioma (LGG) did not show a significant difference in expression. As a whole, we found that human tumors expressed a high level of GAPDH.
Apart from transcription, we evaluated GAPDH at the protein level using the CPTAC proteome dataset. Tumor tissues from OV, KIRC, LUAD and PAAD were significantly more likely to express GAPDH than normal tissues (Figure 1C, P<0.05). Also, we analyzed IHC results obtained from HPA and compared them to data from CPTAC. The results of the analyses of the two databases were in agreement. The IHC staining of normal ovary, kidney, lung and pancreas tissues was low or medium, while the staining of tumor tissues was medium or strong (Figure 1C).
Survival analysis data of GAPDH gene
Next, we were interested in determining whether GAPDH expression correlated with prognosis and OS. Based on GAPDH expression levels in cancer cases, we divided the cases into high and low groups, and then TCGA and GEO datasets were used to investigate the relationship between GAPDH expression and prognosis among different tumor types. It has been found that tumors expressing high levels of GAPDH have poor OS. This is true for cervical squamous cell carcinoma and endocervical adenocarcinoma (CESC) (P=0.0022), glioblastoma multiforme (GBM) (P=0.023), LGG (P=1.7e−05), liver hepatocellular carcinoma (LIHC) (P=2.1e−05), LUAD (P=3e−04) and mesothelioma (MESO) (P=0.00061) (Figure 2A). Further, Figure 2B shows the association between the GAPDH gene expression and the DFS rates in KIRC (P=0.039), kidney renal papillary cell carcinoma (KIRP) (P=0.0089), LGG (P=0.003), MESO (P=0.036), PAAD (P=0.0081), sarcoma (SARC) (P=0.038) and THYM (P=0.026).
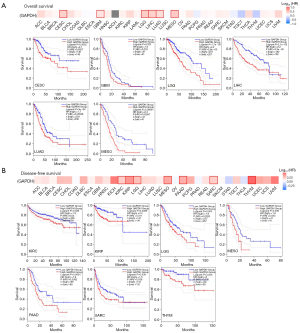
Genetic alteration analysis data
As a consequence of genetic alterations in human cancers, we would like to analyze how the genetic changes in GAPDH are reflected in tumors. The GAPDH gene is altered in 2.1% (231/10,967) of queried TCGA tumor samples, and the classification of genetic alterations was shown in Figure 3A. Further, a high frequency of GAPDH alteration (>6%) was found in seminoma where “amplification” is the primary type (Figure 3B). Additionally, to find out whether the highest degree of “amplification” of GAPDH is related to mRNA expression, we systematically studied and correlated these in Seminoma. Compared with samples without copy number changes in GAPDH, those with GAPDH alterations had an increased mRNA expression (Figure 3C).
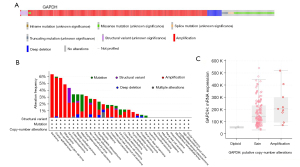
Immune infiltration analysis data
Because GAPDH participates in the regulation of nitrosylation and nuclear functions, alterations in expression levels of GAPDH or mutations in the gene may alter how immune cells respond to tumors. Therefore, we examined the correlation between various levels of endothelial and immune cell infiltration and GAPDH expression in a variety of tumor types studied in the TCGA using TIMER, XCELL, QUANTISEQ and so on. It is interesting to note that expression of GAPDH negatively correlates with the estimated infiltration of cancer-associated fibroblasts (CAFs) in the breast invasive carcinoma (BRCA), SARC and THYM. Also, HNSC neutrophil cell infiltration and GAPDH expression exhibited negative correlations. For BLCA, BRCA, colon adenocarcinoma (COAD), esophageal carcinoma (ESCA), KIRC, LUAD, LUSC, PAAD, SARC, stomach adenocarcinoma (STAD), THYM and UCS as well, GAPDH expression and endothelial infiltration showed negative correlations, as well as a positive correlation in THCA tumors (Figure 4).
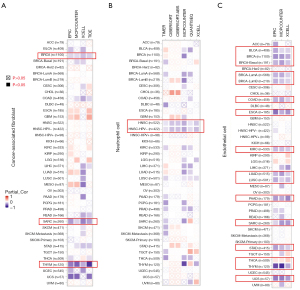
Enrichment analysis of GAPDH gene-related partners
Finally, to determine the molecular mechanism for how the GAPDH gene affects tumorigenesis and development, a series of pathway enrichment analyses were carried out based on the proteins that interact with GAPDH and the GAPDH expression correlation genes. With the STRING tool, we obtained a total of 50 experimentally detected GAPDH-binding proteins. Figure 5A shows the interaction network of these 50 proteins. Then, GEPIA2 combined the data of all TCGA tumors, allowing us to acquire genes ranking in the top 100 that correlate with GAPDH expression. A positive correlation existed between GAPDH expression and that of triosephosphate isomerase 1 (TPI1) (R=0.80), enolase 1 (ENO1) (R=0.57), prohibitin 2 (PHB2) (R=0.56) and pyruvate kinase M1/2 (PKM) (R=0.53) (Figure 5B). And the heatmaps indicated that GAPDH correlated strongly with the four genes listed above in most cancer types (Figure 5C).
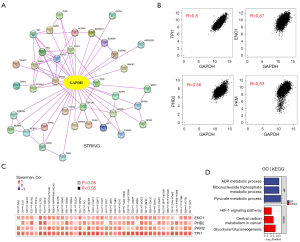
After combining the two datasets, we performed GO and KEGG enrichment analyses, which identified “Pyruvate metabolic process” and “Glycolysis/gluconeogenesis” on the list of top hits (Figure 5D), indicating that these pathways might be involved in GAPDH-induced cancer.
Discussion
The GAPDH gene on chromosome 12 encodes a single mRNA species that results in the production of a polypeptide comprising 335 amino acids, as a homo tetramer made up of four identical subunits of 37 kDa. Even though a lot of companies use GAPDH for internal control, it is expressed differently in several kinds of human cells (14). Levels of GAPDH are also reported to be unusually elevated in some types of cancer in humans (15,16).
In addition to its glycolytic function, GAPDH is involved in numerous cellular functions, including DNA repair and apoptosis (17,18). Hence, the GAPDH deregulation in cancers suggests that this enzyme has inconsistent roles in determining cell fate. However, it remains unclear whether GAPDH plays a role in certain types or common pathways involved in the development of tumors. Therefore, we conducted a pan-cancer analysis of GAPDH. According to the TCGA data, we examined GAPDH gene expression in 33 different tumors. A systematic collection of protein data, as well as molecular and genetic characteristics, was also conducted using other databases.
Based on our results, GAPDH expression levels in 27 tumor tissues are higher than those in the control tissues, regardless of the presence of PRAD, LAML, MESO, SARC and uveal melanoma (UVM). The similar expression levels of GAPDH in different tumor types may be due to common underlying functions and mechanisms. At the same time, we also compared the expression level of GAPDH total protein and found a higher level of GAPDH protein in the tumor tissues of OV, KIRC, LUAD and PAAD patients than in their corresponding control tissues. This difference suggests that GAPDH might not be suitable as an internal reference gene for several cancer research. Furthermore, we found that overexpression of GAPDH generally predicted a poor prognosis for patients with cancers expressing high levels of GAPDH, such as LGG and MESO. In MESO, a high GAPDH level was related to poor OS (P=0.00061) and DFS (P=0.036). GAPDH was rarely reported to be associated with MESO. The results of our study may lead to a prospective clinical biomarker for the prediction of survival rates among patients with MESO.
Evidence is growing that cancer metabolism not only has a critical role to play in cancer signaling for maintenance of tumors and survival, but also contributes to regulating the antitumor immune response by affecting both metabolites and immune cells (19,20). In this study, there is statistically significant evidence of negative or positive comparison of GAPDH expression and CAF, neutrophil, endothelial counts in a few types of tumors, which indicate that GAPDH would be of great significance in tumor immunity. Besides, the hyperoxia signaling pathway is involved in aggressive tumor behavior (21), and the GAPDH gene contains a hypoxia response element with a hypoxia inducible factor-1 (HIF-1) transcription factor binding site. By activating HIF-1, the expression of enzymes involved in glycolysis, such as GAPDH, could be enhanced by p-AKT in cancer cells of the colon, kidney and liver (22-24). Corresponding to this, our analysis of KEGG pathways identified “Glycolysis/gluconeogenesis” and “HIF-1 signaling pathway” as the enriched hits.
Conclusions
As a result of our analysis, we found a statistically significant link between GAPDH expression, clinical outcome and immune cell infiltration for a variety of tumors, providing a variety of viewpoints regarding GAPDH’s role in tumorigenesis.
Acknowledgments
Funding: This work was supported by
Footnote
Reporting Checklist: The authors have completed the REMARK reporting checklist. Available at https://tcr.amegroups.com/article/view/10.21037/tcr-22-1972/rc
Conflicts of Interest: All authors have completed the ICMJE uniform disclosure form (available at https://tcr.amegroups.com/article/view/10.21037/tcr-22-1972/coif). The authors have no conflicts of interest to declare.
Ethical Statement: The authors are accountable for all aspects of the work in ensuring that questions related to the accuracy or integrity of any part of the work are appropriately investigated and resolved. The study was conducted in accordance with the Declaration of Helsinki (as revised in 2013).
Open Access Statement: This is an Open Access article distributed in accordance with the Creative Commons Attribution-NonCommercial-NoDerivs 4.0 International License (CC BY-NC-ND 4.0), which permits the non-commercial replication and distribution of the article with the strict proviso that no changes or edits are made and the original work is properly cited (including links to both the formal publication through the relevant DOI and the license). See: https://creativecommons.org/licenses/by-nc-nd/4.0/.
References
- Wang Z, Jensen MA, Zenklusen JC. A Practical Guide to The Cancer Genome Atlas (TCGA). Methods Mol Biol 2016;1418:111-41. [Crossref] [PubMed]
- Chen W, Liu D, Wang G, et al. Screening diagnostic markers for acute myeloid leukemia based on bioinformatics analysis. Transl Cancer Res 2022;11:1722-9. [Crossref] [PubMed]
- Li Y, Gu J, Xu F, et al. Transcriptomic and functional network features of lung squamous cell carcinoma through integrative analysis of GEO and TCGA data. Sci Rep 2018;8:15834. [Crossref] [PubMed]
- Zhang JY, Zhang F, Hong CQ, et al. Critical protein GAPDH and its regulatory mechanisms in cancer cells. Cancer Biol Med 2015;12:10-22. [PubMed]
- Wisnieski F, Calcagno DQ, Leal MF, et al. Reference genes for quantitative RT-PCR data in gastric tissues and cell lines. World J Gastroenterol 2013;19:7121-8. [Crossref] [PubMed]
- Ci S, Xia W, Liang W, et al. Src-mediated phosphorylation of GAPDH regulates its nuclear localization and cellular response to DNA damage. FASEB J 2020;34:10443-61. [Crossref] [PubMed]
- Yang M, Yan J, Wu A, et al. Alterations of housekeeping proteins in human aged and diseased hearts. Pflugers Arch 2021;473:351-62. [Crossref] [PubMed]
- Butera G, Mullappilly N, Masetto F, et al. Regulation of Autophagy by Nuclear GAPDH and Its Aggregates in Cancer and Neurodegenerative Disorders. Int J Mol Sci 2019;20:2062. [Crossref] [PubMed]
- Li T, Fan J, Wang B, et al. TIMER: A Web Server for Comprehensive Analysis of Tumor-Infiltrating Immune Cells. Cancer Res 2017;77:e108-10. [Crossref] [PubMed]
- Tang Z, Kang B, Li C, et al. GEPIA2: an enhanced web server for large-scale expression profiling and interactive analysis. Nucleic Acids Res 2019;47:W556-60. [Crossref] [PubMed]
- Chandrashekar DS, Bashel B, Balasubramanya SAH, et al. UALCAN: A Portal for Facilitating Tumor Subgroup Gene Expression and Survival Analyses. Neoplasia 2017;19:649-58. [Crossref] [PubMed]
- Wu P, Heins ZJ, Muller JT, et al. Integration and Analysis of CPTAC Proteomics Data in the Context of Cancer Genomics in the cBioPortal. Mol Cell Proteomics 2019;18:1893-8. [Crossref] [PubMed]
- Szklarczyk D, Gable AL, Nastou KC, et al. The STRING database in 2021: customizable protein-protein networks, and functional characterization of user-uploaded gene/measurement sets. Nucleic Acids Res 2021;49:D605-12. [Crossref] [PubMed]
- Colombe AS, Gerbaud P, Benitah JP, et al. Housekeeping Proteins Exhibit a High Level of Expression Variability Within Control Group and Between Ischemic Human Heart Biopsies. J Am Heart Assoc 2022;11:e026292. [Crossref] [PubMed]
- Guo Y, Li Q, Ren W, et al. Quantitative Proteomics Reveals Down-Regulated Glycolysis/Gluconeogenesis in the Large-Duct Type Intrahepatic Cholangiocarcinoma. J Proteome Res 2022;21:2504-14. [Crossref] [PubMed]
- Sirover MA. Pleiotropic effects of moonlighting glyceraldehyde-3-phosphate dehydrogenase (GAPDH) in cancer progression, invasiveness, and metastases. Cancer Metastasis Rev 2018;37:665-76. [Crossref] [PubMed]
- Kosova AA, Khodyreva SN, Lavrik OI. Role of Glyceraldehyde-3-Phosphate Dehydrogenase (GAPDH) in DNA Repair. Biochemistry (Mosc) 2017;82:643-54. [Crossref] [PubMed]
- Ping Z, Fan H, Wen C, et al. GAPDH siRNA Regulates SH-SY5Y Cell Apoptosis Induced by Exogenous α-Synuclein Protein. Neuroscience 2021;469:91-102. [Crossref] [PubMed]
- Xia L, Oyang L, Lin J, et al. The cancer metabolic reprogramming and immune response. Mol Cancer 2021;20:28. [Crossref] [PubMed]
- Corrado M, Pearce EL. Targeting memory T cell metabolism to improve immunity. J Clin Invest 2022;132:e148546. [Crossref] [PubMed]
- Ristescu AI, Tiron CE, Tiron A, et al. Exploring Hyperoxia Effects in Cancer-From Perioperative Clinical Data to Potential Molecular Mechanisms. Biomedicines 2021;9:1213. [Crossref] [PubMed]
- Sun LT, Zhang LY, Shan FY, et al. Jiedu Sangen decoction inhibits chemoresistance to 5-fluorouracil of colorectal cancer cells by suppressing glycolysis via PI3K/AKT/HIF-1α signaling pathway. Chin J Nat Med 2021;19:143-52. [Crossref] [PubMed]
- Zoa A, Yang Y, Huang W, et al. High expression of hypoxia-inducible factor 1-alpha predicts poor prognosis in pancreatic ductal adenocarcinoma: a meta-analysis and database validation protocol. Transl Cancer Res 2022;11:3080-91. [Crossref] [PubMed]
- Lee SH, Golinska M, Griffiths JR. HIF-1-Independent Mechanisms Regulating Metabolic Adaptation in Hypoxic Cancer Cells. Cells 2021;10:2371. [Crossref] [PubMed]