TFF3 promotes clonogenic survival of colorectal cancer cells through upregulation of EP4 via activation of STAT3
Highlight box
Key findings
• TFF3 is a novel regulator of EP4 expression, and STAT3 signaling is responsible for TFF3-induced EP4 expression.
What is known and what is new?
• TFF3 is involved in CRC progression and intestinal mucosal repair. TFF3 promotes survival, proliferation, invasion, and metastasis, and inhibits apoptosis of CRC cells.
• In this study, we found that TFF3 promotes clonogenic survival of CRC cells via upregulating EP4 expression.
What is the implication, and what should change now?
• Blocking TFF3-induced EP4 expression may be a promising strategy for CRC treatment.
Introduction
Colorectal cancer (CRC) is the world’s second most deadly cancer, which accounts for 10% of all annually diagnosed cancers and cancer-related deaths worldwide (1,2). Proliferation is an important part of cancer development and progression. Over the past few years, numerous studies committed to decoding the mystery of sustained proliferation of cancer cells (3,4), however, the underlying molecular mechanisms are considered more complicated than previously imagined and are far from clear.
TFF3 is one of the trefoil factor family of proteins, which are highly conserved during evolution and are heat, acid, and enzyme resistant (5). TFF3 contains 59 amino acid residues and a single three-loop trefoil domain, which consists of 42 residues and three disulfide bonds (Cys11-Cys37, Cys21-Cys36 and Cys31-Cys48) resulting in the characteristic trefoil structure (5). TFF3 is expressed in the epithelial mucosal layer of the small intestine, colon, hypothalamus, pituitary, thyroid gland, lower respiratory tract, cervix, salivary gland and uterus (5). TFF3 expression is dysregulated in various cancer tissues, including colorectal (6,7), cervical (8), prostate (9), gastric (10,11), hepatocellular (12), and mammary carcinomas (13,14). Previous studies showed that TFF3 regulates cancer cell proliferation (15-17), however, the underlying mechanisms are far from clear.
Single cells to survive and reproduce to form colonies is a key ability for tumor cells, which is interpreted as a trait of cancer cells with tumor-initiating capabilities (18). Here, we found that TFF3 promotes clonogenic survival of CRC cells and explored the underlying mechanisms. We present this article in accordance with the MDAR reporting checklist (available at https://tcr.amegroups.com/article/view/10.21037/tcr-22-2552/rc).
Methods
Reagents
PGE2 was obtained from Tocris Bioscience (Minneapolis, MN, USA). L-902688 and ONO-AE3-208 were obtained from MedChemExpress (Princeton, NJ, USA). Niclosamide was obtained from Selleck Chemicals (Houston, TX, USA). Anti-TFF3 antibody (sc-398651) and anti-EP4 antibody (sc-55596) were obtained from Santa Cruz Biotechnology (Santa Cruz, CA, USA). Anti-tubulin antibody (66031-1-Ig) was obtained from Proteintech (Wuhan, China). Anti-STAT3 antibody (12640) and anti-p-STAT3 antibody (9145) were obtained from Cell Signaling Technology (Danvers, MA, USA). Goat anti-mouse IgG (H+L) antibody (A-11001, Alexa Fluor 488 conjugate) and Goat anti-Rabbit IgG (H+L) antibody (A32732, Alexa Fluor Plus 555 conjugate) were obtained from Thermo Fisher Scientific (Waltham, MA, USA).
Cell lines
The HCT-8 (Manassas, VA, USA), SW620 (Manassas, VA, USA), and HCT116 (Manassas, VA, USA) CRC cell lines were purchased from American Type Culture Collection (ATCC) and cultured in RPMI 1640 medium supplemented with 10% fetal bovine serum. HEK293T cells were obtained from ATCC and cultured in Dulbecco’s modified Eagle’s medium supplemented with 10% fetal bovine serum. The TFF3 knockout cell line SW620KO was generated using the CRISPR/Cas9 system. All-in-one plasmid lentiCRISPR v2 was obtained from Addgene (Watertown, MA, USA). On-target sequence was 5'-ATGTCACCCCCAAGGAGTGC-3'. HEK293T cells were used to produce lentivirus and the viral supernatant was aliquoted and stored at −80 ℃. SW620 cells were seeded at 5×105 cells/2 mL in antibiotic free RPMI 1640 medium. Twenty-four hours later, 100 µL viral supernatant was added to the 2 mL of media on the cells and gently rocked plate to mix. At 48 hours after infection, cells were screened using puromycin for 6 days at a final concentration of 2 µg/mL. Single cell-derived clones were obtained by limiting dilution and edited single clones were verified by western blotting. HCT-8 cells overexpressing TFF3 were generated by transfecting HCT-8 cells with TFF3/pcDNA3.1(+). Positive cells were screened using G418 at a final concentration of 100 µg/mL. A stable cell pool was used for biological assays. All cells were cultured at 37 ℃ and with 5% CO2.
Human tissues
Surgically resected CRC tissues were collected from the Department of Gastrointestinal Surgery, Xijing Hospital. All tissue specimens were frozen at −80 ℃ or formalin-fixed. HE staining was performed and two experienced pathologists reviewed the tissue sections. All human individuals provided written informed consent. The study was approved by the Xijing Hospital Ethics Committee (No. KY20213194-1). The study was conducted in accordance with the Declaration of Helsinki (as revised in 2013).
Clonogenic assay
Clonogenic assays were performed as previously described (19). The cells were cultured for about 15 days until visible clones emerged. After being washed twice with phosphate buffered saline (PBS), the cells were fixed with 2% paraformaldehyde at 25 ℃ for 10 min and stained with 0.2% crystal violet at 25 ℃ for 30 min. The plates were washed three times with PBS to remove excess dye. The cell clones in each well were counted.
RNA interference with small interfering RNA (siRNA)
Specific siRNA and scramble control siRNAs were obtained from GenePharma (Shanghai, China). A mixture of two individual siRNAs, each aimed at a different region of the STAT3 mRNA, was used for silencing STAT3. Cells were seeded in 6-well plates and transfected with 20 nM siRNAs using Lipofectamine 2000 (Invitrogen, Waltham, MA, USA) according to the manufacturer’s instructions. The siRNA sequences were as follows: siSTAT3-1: 5'-CCACUUUGGUGUUUCAUAA-3'; siSTAT3-2: 5'-GAGCUGCAAACAACUAUAC-3'; scramble siRNA: 5'-UUCUCCGAACGUGUCACGU-3'.
Western blotting
Tissues or cell pellets were lysed using cold RIPA buffer (Beyotime, Shanghai, China) containing protease inhibitors (Roche, Basel, Switzerland). Protein concentration was measured using a BCA kit (Beyotime). Equal amounts of protein were denatured in 5× Laemmli buffer (Beyotime), separated by 12% or 15% SDS-PAGE (for TFF3), and transferred to polyvinylidene fluoride membranes (Millipore, Boston, MA, USA). The membranes were blocked with 5% nonfat milk in PBST at 25 ℃ for 40 min. Then membranes were incubated with primary antibodies at 4 ℃ overnight. After being washed with PBST for four times, the membranes were incubated with secondary antibodies at 25 ℃ for 50 min. Immunoblots were developed using an ECL kit (Beyotime).
Reverse transcription quantitative polymerase chain reaction (qPCR)
Total RNA was extracted using TRIzol reagent (OMEGA Bio-Tek, Norcross, GA, USA) according to the manufacturer’s instructions. Reverse transcription was performed using a PrimeScript RT Reagent Kit (Takara Bio Inc., Shiga, Japan). All primers were synthesized by Tsingke Biotechnology (Beijing, China). The sequences of primers were as follows: PTGER4 sense, 5'-CCGGCGGTGATGTTCATCTT-3'; antisense, 5'-CCCACATACCAGCGTGTAGAA-3'; GAPDH sense, 5'-GCACCGTCAAGGCTGAGAAC-3'; antisense, 5'-TGGTGAAGACGCCAGTGGA-3'. Real-time PCR was performed using the TB Green Premix Ex Taq™ KIT (Takara Bio Inc.) on an Agilent Mx3005P, and the data were analyzed using the MxPro-Mx3005P software.
Luciferase reporter assay
The pGL3-Basic reporter vector containing the promoter of PTGER4 was constructed by GenScript (Nanjing, China). The luciferase construct and pRL-TK (Beyotime) were co-transfected into the CRC cells. The cells were cultured for 48 hours and assayed using the Dual-Luciferase® Reporter Assay System (Promega, Madison, WI, USA) according to the manufacturer’s instructions. The relative luciferase activity was calculated by dividing results from the Firefly luciferase assay over the Renilla luciferase assay. The following equation was used to determine the normalized fold change in luciferase activity between test groups:
Each construct was compared to the luciferase activity of construct pGL3-Basic (an empty vector). The normalized fold changes in luciferase activity from each experiment were averaged together, and the statistical significance determined.
Immunofluorescence staining
Immunofluorescence staining was performed as previously described (20). Briefly, cells were inoculated into cell culture dishes (NEST, Wuxi, China). After being washed with PBS, the cells were fixed with 2% paraformaldehyde at 25 ℃ for 10 min, permeabilized with 0.1% Triton X-100 at 25 ℃ for 2 min, and blocked with 1% bovine serum albumin at 25 ℃ for 30 min. The cells were then incubated with the indicated primary antibodies at 25 ℃ for 90 min, washed three times with PBS, and incubated with the appropriate secondary antibodies at 25 ℃ for 30 min. Cell nuclei were stained with DAPI (Vector Labs, California, USA). Cells were visualized using an A1R-A1 confocal laser microscope system (Nikon, Tokyo, Japan).
Immunohistochemistry (IHC)
Tissues were fixed in 10% formalin and embedded in paraffin. Antigen demasking was performed using 10 mmol/L sodium citrate buffer. 3% H2O2 was used to block endogenous peroxidase activity. Goat serum was used to block nonspecific background staining. The sections were incubated at 4 ℃ overnight with primary antibodies, washed three times with PBS, and then incubated with biotinylated secondary antibodies at 25 ℃ for 40 min. Visualization was performed using the DAB horseradish peroxidase color development kit (ZSGB-BIO, Beijing, China). The IHC scores were determined by two senior pathologists according to the proportion and intensity of positive cells: 0 (no staining), 1 (any percentage with weak intensity), 2 (30–50% with strong intensity), and 3 (>50% with strong intensity).
Statistical analysis
Quantitative results are presented as the mean ± standard deviation. Student’s t-test was used to compare the differences between two samples. One-way ANOVA was used to compare the means of more than two groups. Spearman’s R was used to determine the correlation between the expression levels of genes or proteins. All reported P values were two-tailed, and P<0.05 was considered significant.
Results
Enhanced TFF3 promotes clonogenic survival of CRC cells
We first set out to assess the expression of TFF3 in human CRC tissues. As shown in Figure 1A, we found that the expression of TFF3 in CRC cells is higher than that in normal colonic epithelial cells, which is consistent with the previous studies (6,7,21). To determine the role of TFF3 in the clonogenic survival of CRC cells, we generated TFF3 knockout SW620 cells (SW620KO, Figure 1B) and found that SW620KO cells showed decreased clonogenic survival ability compared to the control cells (Figure 1C). We also restored TFF3 expression in SW620KO cells and found that recovery expression of TFF3 in SW620KO cells (Figure 1D) rescued the clonogenic survival ability of SW620KO cells (Figure 1E). To further validate this observation, we overexpressed TFF3 in HCT-8 cells, which have no endogenous TFF3 expression (Figure 1F). As expected, HCT-8 cells overexpressing TFF3 (HCT-8-TFF3) showed enhanced clonogenic survival ability compared to the control cells (Figure 1G). All these results suggest that elevated TFF3 contributes to the clonogenic survival of CRC cells.
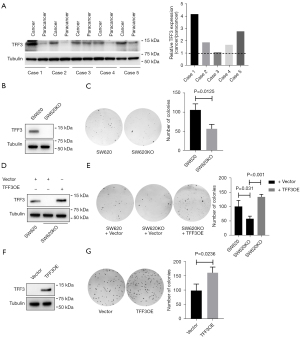
PGE2-EP4 signaling contributes to TFF3-mediated clonogenic survival of CRC cells
As previous studies demonstrated that PGE2-EP4 signaling facilitates clonogenic survival of cancer cells (22), and TFF3 promotes PTGS2 expression and PGE2 production in CRC cells (6), we examined the involvement of PGE2-EP4 signaling in TFF3-enhanced clonogenic survival of CRC cells. As shown in Figure 2A, we treated TFF3 knockout cell line SW620KO with PGE2 or L-902688, a highly potent agonist of EP4, and found that PGE2, as well as L-902688, reversed the effects of TFF3 knockout on the clonogenic survival of SW620 cells. Next, we treated HCT-8 cells overexpressing TFF3 with ONO-AE3-208, an inhibitor of EP4, and found that EP4 inhibition attenuated the increase in clonogenic survival induced by TFF3 overexpression (Figure 2B). All these results suggest a potential role of PGE2-EP4 signaling in TFF3-spured clonogenic survival of CRC cells.
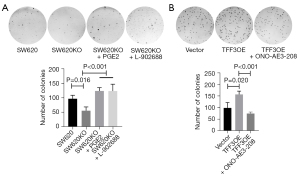
TFF3 promotes EP4 expression
EP4 is the major receptor for PGE2 on CRC cells (6), we determined the effects of TFF3 on EP4 expression. As shown in Figure 3A,3B, we found that EP4 expression was decreased in SW620KO cells compared to control cells, at both mRNA and protein levels. As expected, overexpression of TFF3 in HCT-8 cells resulted in increased EP4 expression (Figure 3C,3D). Gene expression analysis using Gene Expression Omnibus (GEO) and The Cancer Genome Atlas (TCGA) data showed that TFF3 and EP4 expression levels were highly correlated (Figure 3E-3G).
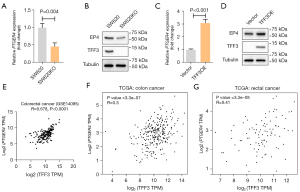
TFF3 transcriptionally enhances EP4 expression
To understand more about how TFF3 promotes EP4 expression, we explored the underlying mechanisms more closely. We constructed an EP4 promoter luciferase reporter (Figure 4A) and found that TFF3 knockout decreased EP4 reporter activity (Figure 4B). As expected, overexpression of TFF3 in HCT-8 cells increased EP4 reporter activity (Figure 4C). Next, we constructed a series of 5'-truncated versions of the EP4 promoter and found that the promoter region between −437 and +1 was indispensable for TFF3-induced transcription of PTGER4 in HCT-8 cells (Figure 4D).
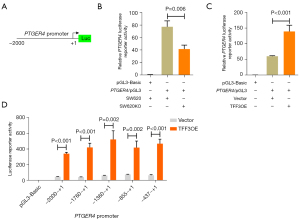
STAT3 mediates TFF3-induced EP4 expression
As TFF3 was reported to activate several intracellular pathways including STAT3 (6,8), we verified these observations and found that overexpression of TFF3 in HCT-8 cells resulted in increased STAT3 phosphorylation (Figure 5A) and nuclear localization (Figure 5B). As expected, SW620 cells showed reduced STAT3 phosphorylation (Figure 5C) and nuclear localization (Figure 5D).
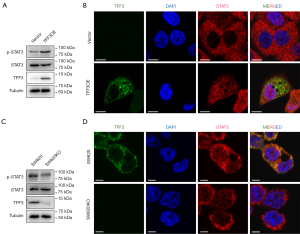
To explore how TFF3 transcriptionally enhances EP4 expression, we analyzed the promoter region between -437 and +1 and found a STAT3 binding site (Figure 6A). Overexpression of STAT3 resulted in increased reporter activity (Figure 6B). Mutation of the STAT3 binding site (Figure 6C) decreased reporter activity (Figure 6D,6E). We also found that TFF3-stimulated EP4 transcription could be blocked by the STAT3 inhibitor niclosamide in a dose-dependent manner (Figure 6F). Consistently, TFF3-induced EP4 protein expression could be reduced by the STAT3 inhibitor niclosamide (Figure 6G) or STAT3 siRNA (Figure 6H). We also confirmed these results in SW620 cells and found that STAT3 inhibition (Figure 6I) and STAT3 silence (Figure 6J) led to decreased EP4 expression. As we previously revealed that TFF3 activates STAT3 signaling via interacting with CD147 (6), we detected EP4 expression in CD147 knockout cells and found that deficiency of CD147 resulted in EP4 downregulation (Figure 6K). These results suggest that TFF3 stimulates EP4 expression mainly via STAT3 signaling.
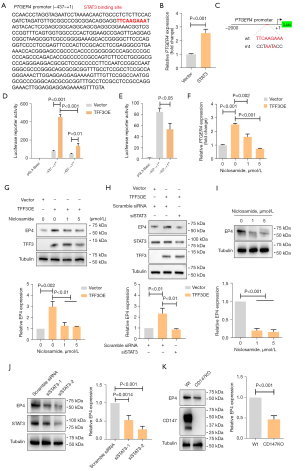
TFF3 correlates with EP4 in human CRC tissues
To determine whether TFF3 regulates EP4 expression in human CRC, we assessed TFF3 and EP4 expression in human CRC tissues by IHC. As shown in Figure 7A, TFF3 expression was closely correlated with EP4 expression. These results suggest that TFF3 promotes EP4 expression in CRC.
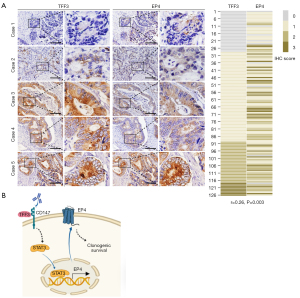
Discussion
PGE2 can be generated by many cells including cancer cells and is involved in several tumor-promoting aspects such as cell proliferation (23,24) and invasion (24,25) through binding to E-type prostanoids (EP) receptors, namely EP1, EP2, EP3 and EP4 receptors. Among the EP receptors, the Gs-protein-coupled EP4 receptor is commonly upregulated in cancer and can be considered as the most important involved in tumorigenic mechanisms (26), which contributes to the pathology of many human malignancies, including those of the colon, breast, prostate, ovary, and lung (27). Elevated EP4 expression in CRC leads to increased cyclic adenosine monophosphate (cAMP) levels contributing to resistance to apoptosis and anchorage-independent growth (28), which is blocked by EP4 inhibitor ONO-AE3-208 (29). Tumor cell colony formation and AKT phosphorylation were significantly inhibited when cells were treated with EP4 receptor-specific antagonist (22). In accordance with these observations, in the present study, we found that PGE2-EP4 signaling promotes clonogenic survival of CRC cells, supporting EP4 as a potential therapeutic target for CRC prevention and treatment.
EP4 receptor protein expression was increased in CRC (100%) as well as adenomas (36%) when compared with normal colonic epithelium (28). However, the causes of EP4 receptor dysregulation in cancer are poorly understood. Kambe et al. reported that specificity protein 1 (Sp-1) binds to the region −197 to −160 of the EP4 promoter to promote its expression (30). EP4 expression is enhanced by c-Myc, which binds to Sp-1 under low cellular density conditions, but is downregulated under high cellular density conditions via HIF-1α, which may prevent c-Myc and Sp-1 from DNA-binding in normal colorectal epithelial cells (31). Oh et al. found that human papillomavirus E5 protein induces EP4 expression in cAMP response element (CRE)-dependent pathway in cervical cancer cells, which is inhibited by an EP4 antagonist, inhibitor of cAMP-dependent protein kinase or phosphatidylinositol 3-kinase, and CRE decoy (32). Transforming growth factor β2 promotes transcription of PTGER4 in transformed macrophage (33). IL10 receptor signal leads to STAT3/SHIP1 dependent expression of EP4 receptor in macrophages (34). In the present study, we revealed that TFF3 is a novel regulator of EP4 expression, and STAT3 signaling is responsible for TFF3-induced EP4 expression (Figure 7B). We previously reported that TFF3 directly interacts with CD147 and induces activation of STAT3 signaling, PTGS2 expression, and CRC progression (6). Therefore, TFF3 stimulates PGE2 signaling at two levels, enhancing PTGS2-mediated PGE2 production and its receptor EP4 expression.
We acknowledge several limitations in the present study. First, we did not investigate the contribution of EP4 to TFF3-enhanced clonogenic survival of CRC cells using in vivo models. Second, given that TFF3 stimulates PGE2 production and EP4 expression, the magnitude of the respective contribution to TFF3-induced PGE2 signaling activation has to be further studied.
Conclusions
This study outlines the regulatory effect of TFF3 on EP4 expression, which is mediated by STAT3 signaling.
Acknowledgments
Funding: This work was supported by
Footnote
Reporting Checklist: The authors have completed the MDAR reporting checklist. Available at https://tcr.amegroups.com/article/view/10.21037/tcr-22-2552/rc
Data Sharing Statement: Available at https://tcr.amegroups.com/article/view/10.21037/tcr-22-2552/dss
Peer Review File: Available at https://tcr.amegroups.com/article/view/10.21037/tcr-22-2552/prf
Conflicts of Interest: All authors have completed the ICMJE uniform disclosure form (available at https://tcr.amegroups.com/article/view/10.21037/tcr-22-2552/coif). The authors have no conflicts of interest to declare.
Ethical Statement: The authors are accountable for all aspects of the work in ensuring that questions related to the accuracy or integrity of any part of the work are appropriately investigated and resolved. The study was approved by the Xijing Hospital Ethics Committee (No. KY20213194-1). The study was conducted in accordance with the Declaration of Helsinki (as revised in 2013). All human individuals provided written informed consent.
Open Access Statement: This is an Open Access article distributed in accordance with the Creative Commons Attribution-NonCommercial-NoDerivs 4.0 International License (CC BY-NC-ND 4.0), which permits the non-commercial replication and distribution of the article with the strict proviso that no changes or edits are made and the original work is properly cited (including links to both the formal publication through the relevant DOI and the license). See: https://creativecommons.org/licenses/by-nc-nd/4.0/.
References
- Qiu H, Cao S, Xu R. Cancer incidence, mortality, and burden in China: a time-trend analysis and comparison with the United States and United Kingdom based on the global epidemiological data released in 2020. Cancer Commun (Lond) 2021;41:1037-48. [Crossref] [PubMed]
- Sung H, Ferlay J, Siegel RL, et al. Global Cancer Statistics 2020: GLOBOCAN Estimates of Incidence and Mortality Worldwide for 36 Cancers in 185 Countries. CA Cancer J Clin 2021;71:209-49. [Crossref] [PubMed]
- Dey P, Kimmelman AC, DePinho RA. Metabolic Codependencies in the Tumor Microenvironment. Cancer Discov 2021;11:1067-81. [Crossref] [PubMed]
- Bonacci T, Emanuele MJ. Dissenting degradation: Deubiquitinases in cell cycle and cancer. Semin Cancer Biol 2020;67:145-58. [Crossref] [PubMed]
- Braga Emidio N, Brierley SM, Schroeder CI, et al. Structure, Function, and Therapeutic Potential of the Trefoil Factor Family in the Gastrointestinal Tract. ACS Pharmacol Transl Sci 2020;3:583-97. [Crossref] [PubMed]
- Cui HY, Wang SJ, Song F, et al. CD147 receptor is essential for TFF3-mediated signaling regulating colorectal cancer progression. Signal Transduct Target Ther 2021;6:268. [Crossref] [PubMed]
- Yusufu A, Shayimu P, Tuerdi R, et al. TFF3 and TFF1 expression levels are elevated in colorectal cancer and promote the malignant behavior of colon cancer by activating the EMT process. Int J Oncol 2019;55:789-804. [Crossref] [PubMed]
- Yuan Z, Chen D, Chen X, et al. Overexpression of trefoil factor 3 (TFF3) contributes to the malignant progression in cervical cancer cells. Cancer Cell Int 2017;17:7. [Crossref] [PubMed]
- Liu J, Kim SY, Shin S, et al. Overexpression of TFF3 is involved in prostate carcinogenesis via blocking mitochondria-mediated apoptosis. Exp Mol Med 2018;50:1-11. [Crossref] [PubMed]
- Taniguchi Y, Kurokawa Y, Takahashi T, et al. Prognostic Value of Trefoil Factor 3 Expression in Patients with Gastric Cancer. World J Surg 2018;42:3997-4004. [Crossref] [PubMed]
- Kirikoshi H, Katoh M. Expression of TFF1, TFF2 and TFF3 in gastric cancer. Int J Oncol 2002;21:655-9. [Crossref] [PubMed]
- You ML, Chen YJ, Chong QY, et al. Trefoil factor 3 mediation of oncogenicity and chemoresistance in hepatocellular carcinoma is AKT-BCL-2 dependent. Oncotarget 2017;8:39323-44. [Crossref] [PubMed]
- Kannan N, Kang J, Kong X, et al. Trefoil factor 3 is oncogenic and mediates anti-estrogen resistance in human mammary carcinoma. Neoplasia 2010;12:1041-53. [Crossref] [PubMed]
- May FE, Westley BR. TFF3 is a valuable predictive biomarker of endocrine response in metastatic breast cancer. Endocr Relat Cancer 2015;22:465-79. [Crossref] [PubMed]
- Cheng F, Wang X, Chiou YS, et al. Trefoil factor 3 promotes pancreatic carcinoma progression via WNT pathway activation mediated by enhanced WNT ligand expression. Cell Death Dis 2022;13:265. [Crossref] [PubMed]
- Liu M, Xiao Y. Trefoil factor 3 silencing can inhibit the proliferation and apoptosis of lung cancer cells. J BUON 2021;26:1842-9. [PubMed]
- Zhang M, Wang B, Chong QY, et al. A novel small-molecule inhibitor of trefoil factor 3 (TFF3) potentiates MEK1/2 inhibition in lung adenocarcinoma. Oncogenesis 2019;8:65. [Crossref] [PubMed]
- Liu M, Yang J, Xu B, et al. Tumor metastasis: Mechanistic insights and therapeutic interventions. MedComm (2020) 2021;2:587-617. [PubMed]
- Lai XR, Wang CL, Qin FZ. The mechanism of LncRNA01977 in lung adenocarcinoma through the SDF-1/CXCR4 pathway. Transl Cancer Res 2022;11:475-87. [Crossref] [PubMed]
- Nan G, Zhao SH, Wang T, et al. CD147 supports paclitaxel resistance via interacting with RanBP1. Oncogene 2022;41:983-96. [Crossref] [PubMed]
- Zhang Z. Trefoil factor 3 knock-down prevents autophagy-related gene 12 elevation in colon adenocarcinoma. J Histotechnol 2019;42:169-76. [Crossref] [PubMed]
- Yang L, Huang Y, Porta R, et al. Host and direct antitumor effects and profound reduction in tumor metastasis with selective EP4 receptor antagonism. Cancer Res 2006;66:9665-72. [Crossref] [PubMed]
- Li Y, Shi J, Qi S, et al. IL-33 facilitates proliferation of colorectal cancer dependent on COX2/PGE(2). J Exp Clin Cancer Res 2018;37:196. [Crossref] [PubMed]
- Sun P, Quan JC, Wang S, et al. lncRNA-PACER upregulates COX-2 and PGE2 through the NF-κB pathway to promote the proliferation and invasion of colorectal-cancer cells. Gastroenterol Rep (Oxf) 2020;9:257-68. [Crossref] [PubMed]
- Wilson DJ, DuBois RN. Role of Prostaglandin E2 in the Progression of Gastrointestinal Cancer. Cancer Prev Res (Phila) 2022;15:355-63. [Crossref] [PubMed]
- Karpisheh V, Joshi N, Zekiy AO, et al. EP4 receptor as a novel promising therapeutic target in colon cancer. Pathol Res Pract 2020;216:153247. [Crossref] [PubMed]
- Ching MM, Reader J, Fulton AM. Eicosanoids in Cancer: Prostaglandin E(2) Receptor 4 in Cancer Therapeutics and Immunotherapy. Front Pharmacol 2020;11:819. [Crossref] [PubMed]
- Chell SD, Witherden IR, Dobson RR, et al. Increased EP4 receptor expression in colorectal cancer progression promotes cell growth and anchorage independence. Cancer Res 2006;66:3106-13. [Crossref] [PubMed]
- Hawcroft G, Ko CW, Hull MA. Prostaglandin E2-EP4 receptor signalling promotes tumorigenic behaviour of HT-29 human colorectal cancer cells. Oncogene 2007;26:3006-19. [Crossref] [PubMed]
- Kambe A, Iguchi G, Moon Y, et al. Regulation of EP4 expression via the Sp-1 transcription factor: inhibition of expression by anti-cancer agents. Biochim Biophys Acta 2008;1783:1211-9. [Crossref] [PubMed]
- Seira N, Yamagata K, Fukushima K, et al. Cellular density-dependent increases in HIF-1α compete with c-Myc to down-regulate human EP4 receptor promoter activity through Sp-1-binding region. Pharmacol Res Perspect 2018;6:e00441. [Crossref] [PubMed]
- Oh JM, Kim SH, Lee YI, et al. Human papillomavirus E5 protein induces expression of the EP4 subtype of prostaglandin E2 receptor in cyclic AMP response element-dependent pathways in cervical cancer cells. Carcinogenesis 2009;30:141-9. [Crossref] [PubMed]
- Haidar M, Echebli N, Ding Y, et al. Transforming growth factor β2 promotes transcription of COX2 and EP4, leading to a prostaglandin E2-driven autostimulatory loop that enhances virulence of Theileria annulata-transformed macrophages. Infect Immun 2015;83:1869-80. [Crossref] [PubMed]
- Samiea A, Yoon JSJ, Cheung ST, et al. Interleukin-10 contributes to PGE2 signalling through upregulation of EP4 via SHIP1 and STAT3. PLoS One 2020;15:e0230427. [Crossref] [PubMed]