Bioactivity of recombinant humanized monoclonal antibody against HER2 in-vivo and in-vitro and its mechanism of action in ovarian cancer
Highlight box
Key findings
• The antibody-dependent cellular cytotoxicity (ADCC) effect mediated by anti-epidermal growth factor receptor 2 (HER2) humanized immunoglobulin G monoclonal antibody (rhHER2-mAb) was investigated, and a mechanism of action through the effective binding of rhHER2-mAb to the HER2 antigen on the cell surface was identified.
What is known and what is new?
• Rh-HER2 humanized recombinant antibody is always used in breast cancer and its application in ovarian cancer is limited. The traditional method to obtain antibodies is to construct stable cell lines and the transient gene expression (TGE) technology is worth more discussion.
• Here, verification in a mouse model also showed that the antibody effectively inhibited ovarian cancer HER2 high-expressing cell lines in vitro and in vivo. And we obtain the humanized monoclonal antibody using transient gene expression technology.
What is the implication, and what should change now?
• The results provide technical support for the future development of HER2-targeted drugs and evidence for humanized monoclonal antibody development using transient gene expression in human embryonic kidney 293F cells.
Introduction
Human epidermal growth factor receptor 2 (HER2), a transmembrane protein with tyrosine protein kinase activity and a molecular weight of 18,500, belongs to the epidermal growth factor receptor (EGFR) family. HER2 has been shown to be overexpressed on the surface of various cancer cells, including colon, gastric, breast, and ovarian cancer cells (1,2). A study has shown the essential role of HER2 in processes such as tumor cell proliferation, transformation, and invasion, and reported that in addition to being a prognostic indicator, HER2 is also an important therapeutic target for precision treatment (3). HER2 gene can promote angiogenesis and increase vascular permeability. Abnormal activation of HER2 gene may lead to tumor formation. Herceptin (trastuzumab) is a humanized monoclonal antibody targeting the extracellular domain of HER2 protein that works by downregulating HER2 receptor expression and blocking ligand-mediated cell signal transduction. Herceptin has been widely used clinically to treat metastatic breast cancer in which the HER2 protein is overexpressed (4,5). Nowadays pertuzumab, trastuzumab plus docetaxel has become the standard treatment against HER2-positive MBC as first-line therapy, T-DM1 (Kadcyla) may be an alternative (6). With the rapid development of targeted therapy, the safety and efficacy of trastuzumab in ovarian cancer have been extensively studied (5).
Ovarian cancer is a common gynecologic malignancy characterized by high mortality and a lack of effective modalities for early diagnosis and the treatment of tumor metastasis. HER2 is a receptor tyrosine kinase (RTK), which plays a vital role in the regulation of proliferation, immigration and differentiation of ovarian cancer, and HER2 is overexpressed in 14% to 42.9% ovarian cancer cases. It is reported that trastuzumab can dose-dependently inhibit the tumor growth of ovarian cancer with HER2 overexpressed and prolong mice survival period (7). Thus, it is of great importance that innovative treatment modalities and novel therapies for ovarian cancer be developed.
Transient gene expression (TGE), a well-established technology for rapid protein production, can facilitate the rapid production of proteins with lower costs, a shorter production period, and less dependence on stable transfection cell banks than traditional stable gene expression approaches. TGE can also enable the rapid production of recombinant proteins in the development and manufacturing of biomacromolecule drugs, such as those used in pre-clinical compound screening, toxicology testing, and early clinical drug production. In the application of TGE, a number of factors affect the yield of recombinant monoclonal antibodies, including the host cell type, culture method, expression vector, molecular structure, transfection method, and the corresponding upstream and downstream processes (8). Among others, human embryonic kidney 293 (HEK293) cells are commonly used mammalian host cells in protein production, which can be used in large-scale culture. Protein products can be folded correctly and post-translationally modified, thus providing high transfection efficiency and yield (9,10). Previous studies have tested the consistency of transient transfection technology and stable transfection expression products and found that different batches of products produced by different technology platforms are consistent in terms of their affinity, secondary structure, thermal stability, and glycosylation modification, which has provided a theoretical basis for the application of TGE in pre-clinical drug development (11-13).
HER2 targeted therapeutics, such as monoclonal antibodies (e.g., trastuzumab and pertuzumab), and tyrosine kinase inhibitors (TKIs) (e.g., lapatinib), have shown significant clinical benefits for patients with early-stage and metastatic HER2-positive breast cancers. However, a large proportion of patients with HER2-positive breast cancer have shown de novo or acquired resistance to trastuzumab (14,15). As the most common type of cancer in women, breast cancer and ovarian cancer faces a significant challenge for drug discovery due to its heterogenous nature, complex molecular subtypes and pathologies. Patients who have experienced metastasis are still facing limited treatment options. Monoclonal antibody (mAb) against HER2-positive breast cancer has developed. The facing challenge make antibody drug conjugates (ADCs) as an emerging class of drugs used in targeted therapies especial for solid tumors, because of their highly selective binding moiety of mAb and highly cytotoxic warheads with defined mechanisms of action. More than ten ADCs have been approved by the Food and Drug Administration (FDA), three of which are for breast cancer indication namely HER2-targeting Kadcyla and ENHERTU, and TROP2-targeting Trodelvy, highlighting the clinical potential of this therapeutic modality for breast cancer treatment (16).
In this study, the light- and heavy-chain expression vectors of rhHER2-mAb were built based on the HEK293f cell TGE system. By optimizing the co-transfection system, rhHER2-mAb was purified and its affinity and antibody-dependent cellular cytotoxicity (ADCC) efficacy were further investigated. The in-vivo and in-vitro experiments in this study have laid a foundation for further research on the bioactivity of rhHER2-mAb and other novel antibodies. We present the following article in accordance with the ARRIVE reporting checklist (available at https://tcr.amegroups.com/article/view/10.21037/tcr-23-432/rc).
Methods
Materials
Escherichia coli (DH5α) was prepared in our laboratory and the eukaryotic expression plasmid (pCDNA3.4) was uesd for this study. The HER2 antibody light chain (LC) and heavy chain (HC) were inserted into the plasmid coding region. The SK-OV-3, OVCAR-3, and A-2780 cells were preserved in our laboratory, and non-obese diabetic/severe combined immunodeficiency (NOD/SCID) (Beijing Vital River Laboratory Animal Technology Co., Ltd., China) mice were fed and kept in the laboratory.
Devices and reagents
DNA and protein gel electrophoresis devices and a gel imaging analysis system were purchased from Shanghai Tianneng Technology, China. The reagents purchased and used in this study included the following: AKTA start fast liquid system (GE Healthcare, USA); DNA polymerase and restriction endonucleases (Takara, Japan); the homologous recombination kit (Nanjing Novazan Biotechnology, China); the DNA gel recovery kit and non-endotoxic mass extraction kit (Axygen, USA); anti-human HER2 antibody (mouse anti-human monoclonal antibody, BD, USA), peroxidase affinipure donkey anti-human immunoglobulin G (IgG1) (H + L) antibody (Jackson, USA); trastuzumab (Herceptin, Roche, USA); electrochemiluminescence luminous chromogenic solution (Suzhou Xinsaimei Biotech, China); 25-kDa linear polyethyleneimine (PEI; Ployscience, USA); freestyle 293 medium, phenol red-free Roswell Park Memorial Institute Medium (RPMI) 1640, McCoy’s 5A Media, L-15 medium, Dulbecco’s Modified Eagle Medium (DMEM), trypsin, fetal bovine serum (FBS), and pancreatin (Gibco, USA and Jinuo Biomedical Technology, China); FBS (BI, Israel); and rProtein affinity chromatography column packing (Yisheng Biotechnology, China). The sequencing and primer synthesis were completed with the help of Shanghai Sangon Biotechnology, China.
Construction of the rhHER2-mAb expression vector
The variable region sequence obtained by screening the laboratory phage library was spliced and cloned into the pCDNA3.4 plasmid to construct the rhHER2-mAb expression vector. The primers were designed according to the gene sequences shown in Table 1. The Nhe I and Hind III restriction sites that were homologous to the sequences of the expression vector pCDNA 3.4 were introduced at the 5'-end of the primer. Overlap polymerase chain reaction (PCR) was used to splice the gene fragments of the light and HCs (17). The LC was obtained by overlap PCR using primers 1, 2, 3, and 4, while the HC was obtained by primers 1, 5, 6, and 7. The pCDNA3.4 plasmid double-digested with Nhe I and Hind III endonuclease were cut and recovered and connected to the overlap PCR product using one step cloning kit. The cloning product was then transformed into Escherichia coli DH5α. The positive clones were selected for culture and sent for sequencing, and the mutation-free clones were selected for the mass sampling of the non-endotoxic plasmid.
Table 1
Primer | Sequence (5'-3') |
---|---|
HER2 LC/HC signal peptide | Forward: CGAACCCTT |
HER2 LC signal peptide | Reverse: CGCTGGGCCCCTTCGTCTTGATCTCCACCTTGGT |
HER2 LC | Forward: ACCAAGGTGGAGATCAAGACGAAGGGGCCCAGCG |
Reverse: GATCGAACCCTT |
|
HER2 HC signal peptide | Reverse: CGCTGGGCCCCTTCGTGCTGCTCACGGTGACGAGG |
HER2 HC | Forward: CCTCGTCACCGTGAGCAGCACGAAGGGGCCCAGCG |
Reverse: GATCGAACCCTT |
The underline means the restriction sites. rhHER2-mAb, recombinant anti-HER2 humanized immunoglobulin G monoclonal antibody; LC, light chain; HC, heavy chain.
TGE of rhHER2-mAb
Optimization of the DNA/PEI ratio
The heavy- and light-chain plasmids were extracted using an endotoxin-free plasmid extraction kit before filtration with a 0.22-µm filter; the HEK293F cells were inoculated into the Freestyle 293 medium and placed in a constant temperature shaker at 37 ℃, 5% carbon dioxide (CO2) and shaken at 125 rpm. The cell density and viability were maintained at [1–2]×106 cells/mL and above 95%. The cells were centrifuged 24 h before transfection and resuspended in fresh medium before being expanded to a density of 0.6×106 cells/mL. On the day of transfection, the cell concentration was measured before the identification of the cell types. The cell density was then adjusted to [1–1.2]×106 cells/mL, and 0.6 mL of the cells was added to a 12-well plate. Based on the predetermined condition that 0.6 µg of plasmid was needed to transfect every 106 cells, the light/heavy-chain plasmids were matched at a mass ratio of 1:1, and DNA and polyethyleneimine (PEI) were mixed at mass ratios of 1:1, 1:2, 1:3, 1:4 or 1:5, respectively and inoculated at room temperature for 25 min before being added to the cell suspension to continue culturing. The supernatant of the cell fermentation broth was collected by centrifugation 48 h later for the subsequent cell identifications.
Meanwhile, the green fluorescent protein and control plasmids were mixed with PEI using the same gradient ratios for transfection. After 48 h, 1×106 cells were collected from each group, centrifuged at 300 ×g at 4 ℃ for 5 min, washed twice with 1 mL of 2% FBS–phosphate buffered saline (PBS) before being resuspended at 100 µL, and passed through a flow cytometer. The transfection efficiency was detected by the fluorescein isothiocyanate channel.
Optimization of LC/HC ratios
Western blotting was used to verify whether the rhHER2-mAb was correctly assembled. Samples were isolated by 10% sodium dodecyl-sulfate polyacrylamide gel electrophoresis (SDS-PAGE) and transferred to a polyvinylidene fluoride (PVDF) membrane before incubation overnight at 4 ℃ with Tris-buffered saline (TBS) containing 5% skimmed milk powder. The next day, the PVDF membrane was incubated with anti-human IgG (H + L) secondary antibody before being rinsed 3 times with TBS for 5 min each time. The western blotting films were then developed. The effects of the DNA/PEI and LC/HC plasmid ratios on the transient expression of rhHER2-mAb were compared.
Purification and identification of rhHER2-mAb
All the purification reagents were filtered with a 0.22-µm membrane, and the cell fermentation broth supernatant was subsequently filtered through a 0.45-µm membrane. The specific processes of rProteinA affinity chromatography were as follows: the AKTA system was rinsed with 20% ethanol and ultrapure water, and the column was connected to position 1 of the AKTA purifier. Binding buffer (pH 7.2, 20 mM of phosphate buffer, and 150 mM of sodium chloride was used to equilibrate the column, and the sample was loaded at a speed of 1 mL/min. After loading, the column was equilibrated again using the binding buffer before the non-specific impurities were removed by using the wash buffer (pH 5.0, and 100 mM of citrate buffer). The protein was eluted with elution buffer (pH 3.0, and 100 mmol/L of citric acid buffer). The eluted sample was then adjusted to a neutral pH with neutralization buffer (pH 8.5, and 1 mol/L of trihydroxymethyl aminomethane hydrochloride buffer), and the column was then washed with 0.5 M of NaOH and 20% ethanol. The obtained product antibody was dialyzed into PBS (pH 7.2), filtered and sterilized, and stored in a −80 ℃ refrigerator.
Affinity detection of rhHER2-mAb
The affinity of rhHER2-mAb was determined by bio-layer interferometry (BLI) using the OctetRed96 system under the following protocol: The streptomycin-affinity (SA) sensor was pre-soaked in PBS buffer for 15 min, and the program was set for antibody affinity detection (18). The SA sensor was then infiltrated into the equilibrium solution (0.02% tween20 in phosphate buffered solution containing 0.05% albumin from bovine serum) for 180 s as the benchmark. The sensor was infiltrated into the solidification solution (5 µg/mL of biotin-labeled HER2 protein) for 600 s. After the signal was stable, the sensor was soaked in the fresh equilibrium solution for 180 s, before being soaked in solutions with rhHER2-mAb concentrations of 50, 25, 12.5, 6.25, or 3.125 nM, and the PBS control, for 450 s. After the signal was stable, the sensor was immersed in PBS and dissociated for 1,800 s. The above experiments were carried out at 30 ℃ with a rotating speed of 1,000 r/min, and the PBS (pH 7.4) used in all steps was from the same batch. The data analysis was performed using the OctetRed96 (USA, Fortebio) analysis software.
Biological activity of rhHER2-mAb
Macoy’5A + 10% FBS, RPMI 1640 + 10% FBS, and DMEM + 10% FBS complete medium were each prepared to culture the SK-OV-3, OVCAR-3, and A2780 cells, respectively, in a 5% CO2 incubator at 37 ℃. The cell surface expression level of HER2 on our panel of cancer cells was tested using flow cytometry.
Lactate dehydrogenase (LDH) cytotoxicity assays were used to detect the ADCC level mediated by rhHER2-mAb, in which LDH was quantitatively measured by the CytoTox 96® Cytotoxicity Detection Kit (CTB163, Promega). In accordance with the manufacturer’s instructions, the experimental and control groups, including the effector cell spontaneous LDH release, target cell spontaneous LDH release, target cell maximum LDH release, volume correction control, and culture medium-only control groups, were set, with 3 replicates per group. Three strains of SK-OV-3, OVCAR-3 and A2780 cells in the growth phase were collected, centrifuged at 1,000 rpm, resuspended in RPMI 1640 + 5% inactivated serum, sampled and counted, before being subsequently plated in 96-well cell culture plates at a density of 8,000 cells/well at 100 µL per well. A490 was detected by a multifunctional microplate reader after sequentially adding the LDH matrix liquid with stop solution in accordance with the manufacturer’s instructions. The cytotoxicity ratios were calculated according to the formula (percent cytotoxicity = 100 × Experimental LDH Release OD490/Maximum LDH Release OD490), the data were processed by GraphPad Prism 5 (USA), and a 4-parameter analysis was adopted to fit a sigmoidal dose-dependent curve.
rhHER2-mAb pharmacokinetics and anti-tumor animal experiments
Animal experiments were performed under a project license (No. 20191512) granted by ethics board of the First Affiliated Hospital of Naval Military Medical University, in compliance with the First Affiliated Hospital of Naval Military Medical University guidelines for the care and use of animals. A protocol was prepared before the study without registration. The blood samples were collected separately and diluted 2,000-fold for quantitative determination as per enzyme-linked immunosorbent assay (ELISA) standard procedures. Specifically, goat anti-human IgG-kappa specific antibodies were incubated overnight in a 96-well high-affinity protein binding plate. rhHER2-mAB was captured using anti-human IgG-kappa antibody, and the signal was then amplified with goat anti-human FcHRP antibody. Tetramethylbenzidine solution was subsequently added to measure absorbance (OD450 absorption). The pharmacokinetic (PK) parameters were analyzed using a non-compartmental analysis model.
The anti-tumor efficacy evaluation of the rhHER2-mAb mice were performed as follows: 15 female NOD/SCID mice (aged 6–8 weeks old) (Beijing Vital River Laboratory Animal Technology Co., Ltd., China) were randomly divided into the following 3 groups: (I) the experimental rhHER2 group; (II) the Herceptin group; and (III) the PBS group. Each mouse underwent subcutaneous implantation (on the right flank area) of 100 µL of peripheral blood mononuclear cell (PBMC) and SK-OV-3 cells with an E/T ratio of 1:4. For each mouse, 5×106 SK-OV-3 cells were mixed with 2×106 inactivated PBMCs in a volume of 100 µL. On the 2nd day, the mice in each group were injected in situ with 1 mg/kg of rhHER2, Herceptin, and PBS as blank controls, and then underwent weekly treatments via the in-situ injection of rhHER2-mAb at 1 mg/kg doses. The tumor size was measured with a vernier caliper every 4 days.
Statistical analysis
Statistical comparisons were carried out by unpaired two-tailed t-test. Family-wise significance level (alpha) was 0.05. All analyses were carried out in GraphPad Prism 8.
Results
Construction of the rhHER2-mAb expression vector
The eukaryotic expression vector pCDNA3.4 was designed and built in this study using the TGE system with the HEK293F mammalian cell as the host cell (Figure 1A,1B). HHER2-mAb LC or HC gene fragments were inserted into the pCDNA3.4 plasmid. The 0.8%–agarose gel electrophoresis results showed that the LC and HC sizes were 708 and 1,413 bp, respectively (Figure 1C). The final sequencing results confirmed that the expression vectors (i.e., pCDNA3.4-anti-Her2 LC and pCDNA3.4-anti-Her2 HC) had been successfully constructed.
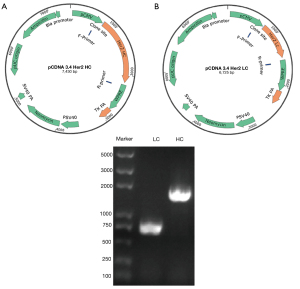
Optimization of rhHER2-mAb TGE
The TGE conditions, such as the DNA/PEI and LC/HC plasmid ratios, were optimized using HEK293F as the host cell and pCDNA3.4 as the eukaryotic expression plasmid. The HC and LC plasmids were mixed with PEI using different gradient ratios for the transfection (Table 2). The results showed that the transfection efficiencies of the DNA/PEI ratios from 1:1 to 1:5 were 5.02%, 12.38%, 35.99%, 90.22%, and 92.34%, respectively (Figure 2A), which suggested that the transfection efficiency exceeded 90% when the DNA/PEI ratios were 1:4 and 1:5. In terms of the cost, a DNA/PEI ratio of 1:4 was set for the subsequent transfection steps with a transfection efficiency of 90.22%. The effects of the DNA/PEI and LC/HC plasmid ratios on the transient expression of rhHER2-mAb were compared. The western blotting results showed that the protein yield was higher when the DNA/PEI ratio was 1:4 and the LC/HC ratio was 2:1. The optimal ratios were then adopted for the subsequent large-scale expression of rhHER2-mAb (Figure 2B,2C). Thus, based on the aforementioned transfection results, the HEK293F cells were transfected at a LC/HC ratio of 2:1 and a DNA/PEI ratio of 1:4 for the subsequent experiments.
Table 2
Parameter | LC/HC ratio | |||||||||
---|---|---|---|---|---|---|---|---|---|---|
5:1 | 4:1 | 3:1 | 3:2 | 2:1 | 1:1 | 1:4 | 2:3 | 1:3 | 1:2 | |
LC, % | 83.4 | 80 | 75 | 60 | 66.6 | 50 | 20 | 40 | 25 | 33.3 |
HC, % | 16.6 | 20 | 25 | 40 | 33.3 | 50 | 80 | 60 | 75 | 66.6 |
Total | 100 | 100 | 100 | 100 | 100 | 100 | 100 | 100 | 100 | 100 |
rhHER2-mAb, recombinant anti-HER2 humanized immunoglobulin G monoclonal antibody; LC, light chain; HC, heavy chain.
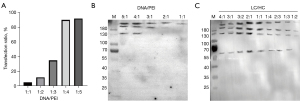
Purification and identification of rhHER2-mAb
rProteinA has high specificity and affinity against the fragment crystallizable (Fc) segment at the stable region of the HC, and good versatility for antibody purification. The rProtein A affinity chromatography results are shown in Figure 3A. The upper column was cleaned with citric acid washing solution (pH 5.0) and replacement solution (pH 3.0) for purified products with no impurities eluted. rhHER2-mAb can be eluted on a large scale using the replacement solution, which presents with a unified peak pattern. Finally, the unintended products were eluted using NaOH. The SDS-PAGE results showed that the molecular weight of the rhHER2 antibody was >180 kDa, and the bands of interest for the reduced sample was clear. The molecular weights of the heavy and LCs were measured at 55 and 25 kDa, respectively (Figure 3B). The purified sample was dialyzed, concentrated, and underwent protein quantification. The protein concentration was determined to be 7.3824 mg/mL, with a yield of about 102.45 mg/L.
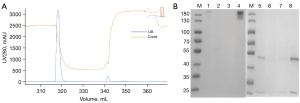
Affinity detection of rhHER2-mAb
The affinity of rhHER2-mAb was determined by BLI using the OctetRed96 (USA, Fortebio) system. After subtracting the data of the PBS control group from the that of the experimental group, the binding and dissociation curve was depicted (Table 3). The binding rate constant (kon) was calculated as 2.69×105 ms−1, and the dissociation rate constant (koff) was 9.43×10−7 s−1. The equilibrium dissociation constant [i.e., the antibody affinity (KD)] was 2.35×10−13 M with an R2 of 0.9538.
Table 3
Affinity of rhHER2-mAb | Value |
---|---|
kon | 2.69×105 ms−1 |
koff | 9.43×10−7 s−1 |
KD | 2.35×10−13 M |
R2 | 0.9538 |
Biological activity of rhHER2-mAb in vitro
In terms of HER2 antigen overexpression on the cell surface, the SK-OV-3, OVCAR-3, and A2780 cell lines were selected as the cells of interest for the investigation of rhHER2-mAb bioactivity (19). The flow cytometry results showed that the cell surface expression level of Her2 was SK-OV-3 > OVCAR3 > A2780 (Figure 4A). The LDH results showed that rhHER2-mAb exerted ADCC against all 3 cell lines at a PBMC ratio of 1:50, and the half-maximal inhibitory concentration (IC50) values of rhHER2-mAb against the SK-OV-3, OVCAR-3, and A2780 cell lines were 6.06, 96.49, and 21.60 ng/mL, respectively (Figure 4B).
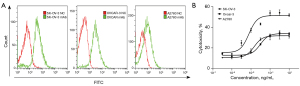
rhHER2-mAb pharmacokinetics and anti-tumor animal experiments
The PK parameters were analyzed using a non-compartmental analysis model, and the half-life of rhHER2-mAb in vivo was found to be approximately 14 days (Figure 5A). The anti-tumor efficacy of rhHER2-mAb was evaluated in the xenograft NOD/SCID mouse model. We compared the rhHER2-mAb, Herceptin, and PBS groups after 60 days, and the results showed that rhHER2-mAb and Herceptin inhibited the growth of SK-OV-3 tumors. rhHER2-mAb and Herceptin were further compared, and the results showed that while both protected the mice from death, rhHER2-mAB was significantly more effective at inhibiting the growth of SK-OV-3 tumors after 60 days. Additionally, observations of subcutaneously denuded tumors revealed that the tumor shape and size of the mice in the PBS group were more irregular and larger, respectively (Figure 5B-5D).
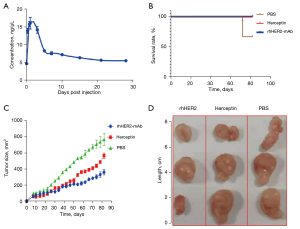
Discussion
Currently, monoclonal antibodies are a mainstay in the biotherapeutic drug market for cancers (20). In the production of recombinant protein drugs, exogenous genes are introduced into host cells and trigger natural selection with screening markers. Exogenous genes are fully integrated into the host genome, and monoclonal cells with stable expression of the desired target genes are ultimately selected (21). The entire acquisition process of stable cells usually takes >6 months. Conversely, TGE technology does not involve the integration of target genes into the host cell genome, and thus avoids the lengthy selection and screening process. However, the expression of the foreign gene is also lost with the division of the host cell. Generally, the entire TGE process takes only a few weeks from the construction of the expression plasmid to the acquisition of the target product, and thus the time and costs of TGE are reduced compared to those of stable transformation (22,23).
The main transfection reagent used in TGE is PEI, which can adsorb to the cell membrane surface of the cells by forming positively charged complexes with DNA. However, serum-free media often contain some components, such as heparin and dextran sulfate, to prevent cell aggregation, which can hinder the formation of positive complexes between PEI and DNA, and thus affects the transfection efficiency of PEI, as extra steps are required, such as culture media exchanges, in large-scale transient infection processes. Various media that supports both high-density cell culture and PEI transformation have been developed, including Freestyle medium from Invitrogen (12338001, USA), which have greatly facilitated the scalability of TGE. In this study, we further explored and selected Freestyle 293 medium and 293F cells, and the pCDNA3.4 expression vector, optimized the PEI-mediated transfection efficiency (1:4) and the co-transfection system with different plasmid ratios (LC/HC =2:1), and ultimately achieved the goal of efficient target protein production.
The affinity of antibodies refers to the binding strength of the antigen-binding cluster of an antibody to the epitope of an antigen (19). Methods for the detection of affinity mainly include ELISA, solid-phase radioimmunoassay, equilibrium dialysis, and Octect and Biacore assays (24). With bio-layer interferometry, the affinity of antibodies can be determined using the Fortebio Octet instrument, and quantitative changes in the number of molecules on the sensor surface and related data on the concentration and kinetics can be obtained. In this study, the Octect assays were used to determine the KD value of rhHER2-mAb (2.35 pM), which is a monoclonal antibody with a high affinity and binding rate that are comparable to those of marketed drugs. In comparing the in-vitro activity of different cells, we found that rhHER2-mAb exerted a killing effect against SK-OV-3 at a low concentration with an IC50 of 6.06 ng/mL.
A study has shown that HER2 siRNA could be a potential drug for the treatment of HER2 positive cancer. Virus-based siRNA and shRNA strategies can effectively solve the problem of siRNA system delivery in vivo, these concerns remain major issues which make it use in clinical regarding immunogenic response and insertional mutagenesis (25). Some studies have shown that co-delivery of HER2 siRNA and monoclonal antibody enhanced the effect especially for tumors that are not sensitive to monoclonal antibody therapy (26,27).
Xenograft NOD/SCID mouse models can be used to evaluate the anti-tumor bioactivity of antibodies (28,29). These mice have severely compromised immunodeficiency without functional T and B lymphocytes; however, the appearance and body weight of SCID mice do not differ to those of normal mice despite their severely atrophied thymus and peripheral lymphatic tissues. This mouse model has had broad-spectrum usage in cancer immunotherapy (30,31). Compared to other obtained commercialized anti-HER2 antibodies, our antibody show higher affinity and better activity in vivo and vitro on ovarian cancer. And here, we used a TGE method to produce our antibodies which allows us to quickly obtain a large number of antibodies. This study evaluated the anti-tumor bioactivity of rhHER2-mAb using a NOD/SCID mouse model and found that the purified rhHER2-mAb after transient expression effectively inhibited the growth of tumors compared to the control group and more effectively killed tumors in an ovarian cancer mouse model compared to Herceptin.
Conclusions
In this study, the ADCC effect mediated by rhHER2-mAb was investigated and a mechanism of action through the effective binding of rhHER2-mAb to the HER2 antigen on the cell surface was observed. When an antibody binds to an antigen on the target cell, the Fc region of the antibody undergoes conformational changes and subsequently binds to Fc receptors on effector T cells, thereby mediating the ADCC effect (32-34). In this study, the rh-HER2 humanized recombinant antibody protein was produced with HER2 as the target, and its ADCC effect on different cell lines was examined. Verification in a mouse model also showed that the antibody effectively inhibited HER2 high-expressing cell lines in vivo. Our findings should provide technical support for the future development of HER2-targeted drugs and also provide evidence of humanized monoclonal antibody development using TGE in HEK293F.
Acknowledgments
Funding: None.
Footnote
Reporting Checklist: The authors have completed the ARRIVE reporting checklist. Available at https://tcr.amegroups.com/article/view/10.21037/tcr-23-432/rc
Data Sharing Statement: Available at https://tcr.amegroups.com/article/view/10.21037/tcr-23-432/dss
Peer Review File: Available at https://tcr.amegroups.com/article/view/10.21037/tcr-23-432/prf
Conflicts of Interest: All authors have completed the ICMJE uniform disclosure form (available at https://tcr.amegroups.com/article/view/10.21037/tcr-23-432/coif). The authors have no conflicts of interest to declare.
Ethical Statement: The authors are accountable for all aspects of the work in ensuring that questions related to the accuracy or integrity of any part of the work are appropriately investigated and resolved. Animal experiments were performed under a project license (No. 20191512) granted by ethics board of the First Affiliated Hospital of Naval Military Medical University, in compliance with the First Affiliated Hospital of Naval Military Medical University guidelines for the care and use of animals.
Open Access Statement: This is an Open Access article distributed in accordance with the Creative Commons Attribution-NonCommercial-NoDerivs 4.0 International License (CC BY-NC-ND 4.0), which permits the non-commercial replication and distribution of the article with the strict proviso that no changes or edits are made and the original work is properly cited (including links to both the formal publication through the relevant DOI and the license). See: https://creativecommons.org/licenses/by-nc-nd/4.0/.
References
- Tandon AK, Clark GM, Chamness GC, et al. HER-2/neu oncogene protein and prognosis in breast cancer. J Clin Oncol 1989;7:1120-8. [Crossref] [PubMed]
- Sanz-Moreno A, Palomeras S, Pedersen K, et al. RANK signaling increases after anti-HER2 therapy contributing to the emergence of resistance in HER2-positive breast cancer. Breast Cancer Res 2021;23:42. [Crossref] [PubMed]
- Slamon DJ, Clark GM, Wong SG, et al. Human breast cancer: correlation of relapse and survival with amplification of the HER-2/neu oncogene. Science 1987;235:177-82. [Crossref] [PubMed]
- Cameron D, Piccart-Gebhart MJ, Gelber RD, et al. 11 years' follow-up of trastuzumab after adjuvant chemotherapy in HER2-positive early breast cancer: final analysis of the HERceptin Adjuvant (HERA) trial. Lancet 2017;389:1195-205. [Crossref] [PubMed]
- Slamon D, Eiermann W, Robert N, et al. Adjuvant trastuzumab in HER2-positive breast cancer. N Engl J Med 2011;365:1273-83. [Crossref] [PubMed]
- Yao M, Fu P. Advances in anti-HER2 therapy in metastatic breast cancer. Chin Clin Oncol 2018;7:27. [Crossref] [PubMed]
- Zhu C, Xu Z, Zhang T, et al. Updates of Pathogenesis, Diagnostic and Therapeutic Perspectives for Ovarian Clear Cell Carcinoma. J Cancer 2021;12:2295-316. [Crossref] [PubMed]
- Nallet S, Fornelli L, Schmitt S, et al. Glycan variability on a recombinant IgG antibody transiently produced in HEK-293E cells. N Biotechnol 2012;29:471-6. [Crossref] [PubMed]
- Gutiérrez-Granados S, Cervera L, Kamen AA, et al. Advancements in mammalian cell transient gene expression (TGE) technology for accelerated production of biologics. Crit Rev Biotechnol 2018;38:918-40. [Crossref] [PubMed]
- Ding K, Han L, Zong H, et al. Production process reproducibility and product quality consistency of transient gene expression in HEK293 cells with anti-PD1 antibody as the model protein. Appl Microbiol Biotechnol 2017;101:1889-98. [Crossref] [PubMed]
- König J, Hust M, van den Heuvel J. Validation of the Production of Antibodies in Different Formats in the HEK 293 Transient Gene Expression System. Methods Mol Biol 2021;2247:59-76. [Crossref] [PubMed]
- da Silva HC Junior. Transient Gene Expression in Human Expi293 Cells. Methods Mol Biol 2022;2406:319-25. [Crossref] [PubMed]
- Greene E, Cazacu D, Tamot N, et al. Optimization of a transient antibody expression platform towards high titer and efficiency. Biotechnol J 2021;16:e2000251. [Crossref] [PubMed]
- Bang YJ, Van Cutsem E, Feyereislova A, et al. Trastuzumab in combination with chemotherapy versus chemotherapy alone for treatment of HER2-positive advanced gastric or gastro-oesophageal junction cancer (ToGA): a phase 3, open-label, randomised controlled trial. Lancet 2010;376:687-97. [Crossref] [PubMed]
- Chan CT, Metz MZ, Kane SE. Differential sensitivities of trastuzumab (Herceptin)-resistant human breast cancer cells to phosphoinositide-3 kinase (PI-3K) and epidermal growth factor receptor (EGFR) kinase inhibitors. Breast Cancer Res Treat 2005;91:187-201. [Crossref] [PubMed]
- Hu L, Hongfeng L. A narrative review of the current landscape and future perspectives of HER2-targeting antibody drug conjugates for advanced breast cancer. Translational Breast Cancer Research 2021;10:vol2.
- Heckman KL, Pease LR. Gene splicing and mutagenesis by PCR-driven overlap extension. Nat Protoc 2007;2:924-32. [Crossref] [PubMed]
- Concepcion J, Witte K, Wartchow C, et al. Label-free detection of biomolecular interactions using BioLayer interferometry for kinetic characterization. Comb Chem High Throughput Screen 2009;12:791-800. [Crossref] [PubMed]
- Rudnick SI, Lou J, Shaller CC, et al. Influence of affinity and antigen internalization on the uptake and penetration of Anti-HER2 antibodies in solid tumors. Cancer Res 2011;71:2250-9. [Crossref] [PubMed]
- Tihanyi B, Nyitray L. Recent advances in CHO cell line development for recombinant protein production. Drug Discov Today Technol 2020;38:25-34. [Crossref] [PubMed]
- Zhu J. Update on Production of Recombinant Therapeutic Protein: Transient Gene Expression. Shropshire, UK: Smithers Papra, 2013.
- Zhu J. Mammalian cell protein expression for biopharmaceutical production. Biotechnol Adv 2012;30:1158-70. [Crossref] [PubMed]
- Porosk L, Nebogatova J, Härk HH, et al. Predicting Transiently Expressed Protein Yields: Comparison of Transfection Methods in CHO and HEK293. Pharmaceutics 2022;14:1949. [Crossref] [PubMed]
- Almeida TB, Panova S, Walser R. NMR Reporter Assays for the Quantification of Weak-Affinity Receptor-Ligand Interactions. SLAS Discov 2021;26:1020-8. [Crossref] [PubMed]
- Gu S, Hu Z, Ngamcherdtrakul W, et al. Therapeutic siRNA for drug-resistant HER2-positive breast cancer. Oncotarget 2016;7:14727-41. [Crossref] [PubMed]
- Zhang L, Mu C, Zhang T, et al. Systemic Delivery of Aptamer-Conjugated XBP1 siRNA Nanoparticles for Efficient Suppression of HER2+ Breast Cancer. ACS Appl Mater Interfaces 2020;12:32360-71. [Crossref] [PubMed]
- Kulhari H, Jangid AK, Adams DJ. Monoclonal Antibody-Conjugated Dendritic Nanostructures for siRNA Delivery. Methods Mol Biol 2019;1974:195-201. [Crossref] [PubMed]
- Ito M, Hiramatsu H, Kobayashi K, et al. NOD/SCID/gamma(c)(null) mouse: an excellent recipient mouse model for engraftment of human cells. Blood 2002;100:3175-82. [Crossref] [PubMed]
- Yu H, Feng Y, Du W, et al. Off-the-shelf GMP-grade UC-MSCs as therapeutic drugs for the amelioration of CCl4-induced acute-on-chronic liver failure in NOD-SCID mice. Int Immunopharmacol 2022;113:109408. [Crossref] [PubMed]
- Okada S, Vaeteewoottacharn K, Kariya R. Application of Highly Immunocompromised Mice for the Establishment of Patient-Derived Xenograft (PDX) Models. Cells 2019;8:889. [Crossref] [PubMed]
- Zhao Y, Shuen TWH, Toh TB, et al. Development of a new patient-derived xenograft humanised mouse model to study human-specific tumour microenvironment and immunotherapy. Gut 2018;67:1845-54. [Crossref] [PubMed]
- Pereira NA, Chan KF, Lin PC, et al. The "less-is-more" in therapeutic antibodies: Afucosylated anti-cancer antibodies with enhanced antibody-dependent cellular cytotoxicity. MAbs 2018;10:693-711. [Crossref] [PubMed]
- Wang Z, Yin C, Lum LG, et al. Bispecific antibody-activated T cells enhance NK cell-mediated antibody-dependent cellular cytotoxicity. J Hematol Oncol 2021;14:204. [Crossref] [PubMed]
- Lajoie L, Congy-Jolivet N, Bolzec A, et al. Gradual Increase of FcγRIIIa/CD16a Expression and Shift toward IFN-γ Secretion during Differentiation of CD56(dim) Natural Killer Cells. Front Immunol 2017;8:1556. [Crossref] [PubMed]
(English Language Editor: L. Huleatt)