Downregulation of CLDN6 inhibits cell migration and invasion and promotes apoptosis by regulation of the JAK2/STAT3 signaling pathway in hepatocellular carcinoma
Highlight box
Key findings
• The downregulation of CLDN6 is sufficient for the reduction of the invading and migrating ability of HCC cells and ultimately resulting in the death of HCC cells by inactivating the JAK2/STAT3 signaling pathway.
What is known and what is new?
• Studies have shown that CLDN6 is highly expressed in liver cancer tissues. JAK and STAT proteins are overexpressed in HCC, and JAK2 and STAT3 proteins are associated with prognosis in HCC patients.
• CLDN6 can promote apoptosis of HCC and down-regulate JAK2/STAT3 signal pathway.
What is the implication, and what should change now?
• This suggests that CLDN6 may be a biomarker of HCC with potential value as a therapeutic target.
Introduction
Epithelium and endothelium separate different tissues to protect multicellular organisms from external effects. These tissues need to form tight junctions (TJs), the selective gates that control the diffusion of ions and solutes around the cells. Additionally, the apical and basal plasma membrane domains are separated by TJ, which is connected to the mechanism that controls the apical-basal polarization (1). TJs are simple static components used to establish cell adhesion structure and are involved in mediating cellular signals. They receive environmental cues and transmit signals within a cell. CLDNs plays a role in maintaining TJ adhesion between cells (2), thus constituting a selective barrier to the pericellular membrane. CLDN is a tetramer protein ranging in size from 20 to 27 kDa. The CLDN family consists of more than 27 members. The sequence analysis of CLDNs leads to two groups according to their sequence similarity, called classical CLDNs and non-classical CLDNs (3).
In human tumors, CLDNs expression is often reduced or eliminated. These findings align with the well-established hypothesis that disruption or ineffectiveness of functional TJs is strongly associated with cancer. Instead, accumulated data show increased or abnormal expression of CLDNs in different cancers, indicating their special role in tumorigenesis. Although the CLDN family exhibits specific distribution patterns in different organs, abnormal expression of CLDNs promotes tumorigenesis. Notably, determining whether the expression of maladjusted CLDNs plays a carcinogenic role or leads to tumor inhibition depending on its target tissues and cells and whether it correlates with tumor outcomes and prognosis contributes to the study of tumor development and progression (4). CLDNs are expressed abnormally at both the transcriptional and post-transcriptional levels in cancer, although recent research has indicated that epigenetic mechanisms, such as DNA methylation, histone modification, and microRNAs (miRNAs), are essential for modulating CLDN expression.
Hepatocellular carcinoma (HCC) is the most common primary liver cancer, accounting for 90% of liver cancers. Common risk factors for HCC are chronic inflammatory infections caused by the hepatitis B and C viruses. These infections lead to cirrhosis and make HCC the cancer with the highest recurrence rate in the world (5). Despite considerable progress and innovation in surgical techniques, chemoradiotherapy, and targeted treatment for liver cancer in recent years, metastasis remains an important factor contributing to poor prognosis. This is why studying the underlying mechanisms of HCC onset and metastasis is essential, along with finding effective biomarkers to help with the diagnosis and targeted therapy (6).
In this research, CLDN6’s role in HCC was demonstrated. Furthermore, it was observed that silencing of CLDN6 significantly promotes apoptosis and inhibits migration and invasion in SMMC-7721 and MHCC-97H cells. The mechanism may be associated with the JAK2/STAT3 signaling pathway. We present this article in accordance with the MDAR reporting checklist (available at https://tcr.amegroups.com/article/view/10.21037/tcr-23-19/rc).
Methods
Cell culture
SMMC-7721 (Cat. No: CC-Y1476) and MHCC-97H (Cat. No: CC-Y1613) cell lines were supplied by Shanghai EK-Bioscience Biotechnology Co., Ltd. (China). Cell culture of every cell line was carried out at 37 ℃ using an incubator comprising 5% CO2. DMEM and RPMI 1640 media (HyClone, Logan, UT, USA) were added with 10% fetal bovine serum (FBS) (Gibco, Grand Island, NY, USA) and penicillin/streptomycin (Life Technologies, Carlsbad, CA, USA).
Transfection
For the downregulation of the CLDN6 gene, four short interfering RNAs (siRNAs) (NC-siRNA sense strand: 5'-UUCUCCGAACGUGUCACGUTT-3' and antisense strand, 5'-AGGUGACACGUUCGGAGAATT-3'; CLDN6-siRNA1 sense strand 5'-CCGGCCAGAUGCAGUGCAATT-3' and antisense strand, 5'-UUGCACUGCAUCUGGCCGGTT-3'; CLDN6-siRNA2 sense strand 5'-GGGAUUGUCUUUGUCAUCUTT-3' and antisense strand, 5'-AGAUGACAAAGACAAUCCCTT-3'; CLDN6-siRNA3 sense strand 5'-CCUACCAAGAAUUACGUCUTT-3' and antisense strand, 5'-AGACGUAAUUCUUGGUAGGTT-3') were purchased from GeneChem (Shanghai, China).
In the next step, a quantitative real-time polymerase chain reaction (qRT-PCR) assay was carried out for the purpose of selecting the sequence that facilitated CLDN6 knockdown for the next experiment. pcDNA-CLDN6 and pcDNA vector (pcDNA-NC) were used for overexpression. Lipo 2000 (Invitrogen, Waltham, MA, USA) was used for cell transfection. Cell inoculation was carried out into six-well plates at a density of 5×105 per well 24 h before transfection. CLDN6-siRNA or pcDNA-CLDN6 plasmids were introduced into each pore cell, respectively. The liquid was replaced after six hours. The cells were gathered for future experimentation one day following transfection.
qRT-PCR assay
RNA separation from the samples was done by utilizing Trizol (Invitrogen) as per the manufacturer’s guidelines, and the NanoVuebles Plus (Thermo Fisher Scientific, Waltham, MA, USA) instrument was employed for measurement of RNA quality by determining the A260/A280 ratio. Subsequently, reverse transcription of 1 g of every µ sample was done with the help of the PrimeScripSphereTakara First Strand cDNA synthesis kit (Takara, Kusatsu, Japan), followed by storage of the resulting product at −80 ℃. In order to perform qRT reactions, SYBR PreMix Ex Taq (Takara) and ABI StepOne PCR Real-time PCR System (Applied Biosystems, Carlsbad, CA, USA) were utilized.
The following primers employed in this research: CLDN6-forward: 5'-CCATCAGGGACTTCTATAA-3', CLDN6-reverse: CAGACGTAATTCTTGGTAGGGT; GAPDH-forward: 5'-CAGGAGGCATTGCTGATGAT-3', GAPDH-reverse: 5'-GAAGGCTGGGGCTCATTT.
Immunofluorescence technique
SMMC-7721 and MHCC-97H cells (4×104/well) were inoculated in confocal Petri dishes overnight at 37 ℃ in an incubator with 5% CO2. After discarding the medium, pre-cooled phosphate buffered saline (PBS) was employed for cell washing after which cell fixation was done with 4% paraformaldehyde for 30 min. Then, they were closed with 3% bovine serum albumin (BSA) for an hour at room temperature. Subsequently, incubation of the dishes was done using a rabbit monoclonal antibody against CLDN6 (Affinity Biosciences Cat# AF5213, Affinity Biosciences, Zhenjiang, China) overnight in an incubator at 4 ℃ and a fluorescent secondary antibody (Affinity Biosciences Cat# S0006) for an hour at room temperature. Visualization of the cells was done using laser scanning confocal microscopy.
Cell Counting Kit-8 (CCK-8) assay
Cell proliferation was assessed by a CCK-8 cell counting kit. The human hepatoma cell line was cultured in 96-well plates at 37 ℃ in an environment containing 5% carbon dioxide. When the cells reached 60% or 70% fusion, CLDN6-siRNA was introduced into the cells, after which they were incubated for 24, 48, or 72 h. Subsequently, 10% CCK-8 was diluted into each hole and incubated for another hour. With the aid of a microplate reader (Bio-Rad, Hercules, CA, USA), optical density (OD) measurements at 450 nm were recorded.
Invasion and migration assays
The assay chamber (Corning Life Sciences, Corning, NY, USA) was exposed to ultraviolet light for 30 min and then used in the experiment. Tumor cell invasion assay requires a pre-coated Matrigel (1:8). The upper portion of the chamber was introduced with tumor cells and serum-free media (migration: 2×104 cells/well; invasion: 5×104 cells/well). In the lower chamber, a conventional medium supplemented with 10% FBS was employed. Following incubation for one day at 37 ℃, the cells were fixed for 15 minutes with 4% paraformaldehyde and stained for 15 minutes with 0.5% crystal violet. A microscope was used to observe and count cells that were migrating or invading.
Wound healing assay
Measurement of cell migration was done by means of the wound healing assay. The cells (5×105/well) were inoculated in a 6-well plate until they reached 80% fusion. Twenty-four hours after transfection, an artificial wound was constructed on the cell monolayer using the tip of a 200 µL sterile pipette, followed by washing thrice with PBS. ImageJ was used to quantify the wound area after the wound was viewed under the inverted microscope for 0, 24, and 48 hours.
Wound healing rate = V0 − Vn/V0, where V0 indicates the initial wound area and Vn refers to the wound area after n hours.
Apoptosis detection
Following 24 hours of transfection, cells were collected by trypsin solution (EDTA-free) and stained with AnnexinV-FITC and PI (BestBio, Shanghai, China). As per the guidelines provided by the manufacturer, 300 µL of 1× Annexin V binding solution was introduced for the purpose of cell resuscitation. After adding 4 µL of Annexin V-FITC, cell incubation was done on ice for 15 min. Subsequently, 8 µL of PI staining solution was added and mixed gently in the absence of light, followed by incubation for 5 min on ice. Afterward, flow cytometry was employed for evaluating target cell apoptosis.
Western blotting
The cells lysed in RIPA buffers containing protease and phosphatase inhibitors. Using a BCA kit, the proteins’ concentrations were measured. SDS-PAGE was used to isolate whole cell proteins, which were then transferred to PVDF membranes (Millipore, Billerica, MA, USA). Blocking of the blots was done using 5% non-fat milk, followed by probing with the primary antibody and placement in a 4 ℃ refrigerator overnight. They were probed again with secondary antibodies for 2 h. For these analytical procedures, β-actin was utilized in the form of loading control. The primary antibodies used were as follows: rabbit anti-JAK2 (1:1,000, Affinity Biosciences Cat# AF6022), anti-STAT3 (1:1,000, Affinity Biosciences, RRID: AB_2835144), anti-p-JAK2 (1:1,000, Affinity Biosciences Cat# AF3024), anti-p-STAT3 (1:1,000, Affinity Biosciences Cat# AF3293), anti-CLDN6 (1:1,000, Affinity Biosciences Cat# AF5213), anti-Bcl-2 (1:1,000, Proteintech, 12789-1-AP; Proteintech, Wuhan, China), anti-cleaved caspase-3 (1:1,000, Proteintech, 66470-2-lg), anti-Bax (1:1,000, Proteintech, 50599-2-lg), and anti-β-actin (1:1,000, Affinity Biosciences, RRID: AB_2839420).
Statistical analysis
Graphpad Prism 8.0 was employed for conducting all statistical analytical procedures. The data were compared using t-test, analysis of variance (ANOVA), and Tukey’s honestly significant difference (HSD) test if appropriate. P<0.05 was considered as the significance threshold.
Results
Inhibition of invasion and migration of SMMC-7721 and MHCC-97H cells by CLDN6 silencing
Initially, treatment of SMMC-7721 and MHCC-97H cells was done with siRNA sequences for 48 h. qRT-PCR was employed for the detection of CLDN6 expression. CLDN6-siRNA2 and CLDN6-siRNA1 significantly down-regulated the expression of CLDN6 in SMMC-7721 (Figure 1A) and MHCC-97H (Figure 1B) cells, respectively. Therefore, these two sequences were used as knockdown sequences in subsequent tests. The findings of the immunofluorescence assay exhibited that following transfection with CLDN6-siRNA, CLDN6 protein expression level was remarkably reduced and increased upon transfection with pcDNA-CLDN6m indicating high transfection efficiency in the aforementioned cells (Figure 1C,1D). Then, transwell invasion and migration were carried out for examination of the influence of CLDN6 on cell invasion and migration. As observed in Figure 2A,2B, the downregulation of CLDN6 significantly blocked the invasion and migration ability of the cells (P<0.001), and through wound healing assay, we can also see the same trend in Figure 2C,2D.
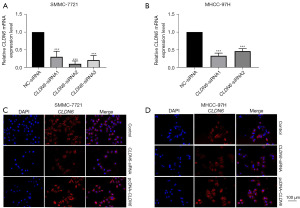
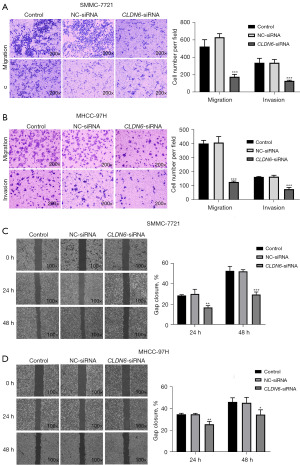
Promotion of SMMC-7721 and MHCC-97H cell apoptosis by CLDN6 silencing
The findings of the CCK-8 assay indicated inhibition of cell proliferation (Figure 3A,3B) after CLDN6-siRNA transfection at 24, 48, and 72 h (P<0.01, P<0.001). Flow cytometry was employed for analyzing tumor cell apoptosis (Figure 3C,3D). After CLDN6 silencing, tumor cell apoptosis appeared remarkably greater in comparison to control cells (P<0.001). The results of WB (Figure 4A,4B) showed the same trend. These findings were indicative of the inhibitory effects of CLDN6 knockdown on the proliferation of these cells, as well as its ability to promote cell apoptosis.
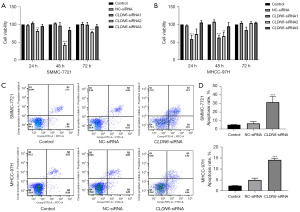
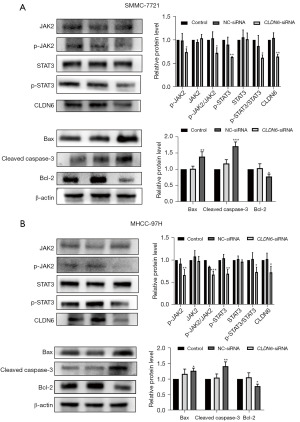
Induction of apoptosis by CLDN6 silencing via downregulation of JAK2/STAT3 signaling pathway activation
In oncogenesis, JAK2/STAT3 signaling is a vital regulator of tumor progression. To evaluate the influence of CLDN6 knockdown on this pathway, the levels of JAK2/STAT3 pathway-related proteins were analyzed through Western blotting. As shown in picture 3, CLDN6 silencing decreased the expression of p-JAK2, p-STAT3, p-JAK2/JAK2, p-STAT3/STAT3, CLDN6, and Bcl-2. However, Bax and cleaved caspase-3 expression levels were increased, while no remarkable differences were observed in JAK2 and STAT3 expression levels (Figure 4). These results clearly showed that CLDN6 silencing induced apoptosis by downregulating the activation of this pathway.
Promotion of HCC cell invasion and migration and inhibition of apoptosis by CLDN6 overexpression
MMC-7721 and MHCC-97H cells were transfected with pcDNA-NC or pcDNA-CLDN6 sequence for 48 h. Western blotting showed increased expression of CLDN6 (Figure 5A,5B), and immunofluorescence (Figure 1C,1D) analysis demonstrated overexpression, elevated Bcl-2 levels, and lowered Bax and cleaved caspase-3 levels. CLDN6 overexpression resulted in the inhibition of HCC cell apoptosis (Figure 5A,5B). The ability of the aforementioned cells to invade and migrate was significantly increased following CLDN6 overexpression. However, cell invasion and migration decreased significantly when a pathway inhibitor (AG490) was used (Figure 5C,5D). These results indicated that CLDN6 regulates cell invasion and migration via JAK2/STAT3 pathway in these cells.
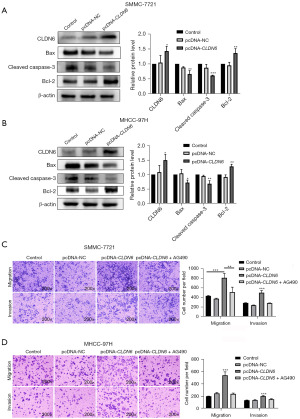
Discussion
TJs are essential for modulating cell polarity, adhesion, and permeability. They are present in the junctional complex connecting epithelial and endothelial cells (7), also, tight junctions must also be selectively permeable to ions, water, and macromolecules (8). Tumors and inflammatory tissues are characterized by decreased TJ integrity and increased cellular bypass permeability (9). The junction adhesion molecules, occludin, and CLDN are the three fundamental membrane proteins that make up TJs. The CLDN protein family is a vital regulator of TJ functioning (10). The CLDN family functions as an important modulator of tumorigenesis and metastasis, the altered expression of CLDN6 is linked to the development of various cancers whose malignant phenotypes include proliferation and apoptosis, migration and invasion and drug resistance (11). CLDN6 varies among tumor cells of various types. The expression of CLDN6 in gastric cancer (12), non-small cell lung cancer (NSCLC) (13), ovarian cancer (14), endometrial cancer (15), and esophageal squamous cell carcinoma (16) are greater than the expression observed in normal tissues. However, its expression is down-regulated in cervical cancer (17) and is undetectable in breast cancer (18). The abnormality of CLDN6 expression in some tumors renders CLDN6 a possible targeting molecule for treating these tumors (19), such as breast cancer (20) and NSCLC (21). The primary objective of this research was the investigation of the CLDN6 mechanism in HCC.
The most prevalent primary liver cancer is HCC, which is also the second-leading contributor to cancer-caused deaths globally (22), accounting for 75% to 85% of cases (23). HCC is the fastest increasing cause of cancer-related death and one of the leading causes of death in patients with compensated cirrhosis (24).
For early HCC, surgical resection and liver transplantation are the major treatment strategies. However, large or multifocal tumors with co-existing liver lesions are sometimes unresectable. Although liver transplantation and surgical resection are the mainstays of HCC treatment, numerous patients are inoperable in advanced stages. In stark contrast to other cancers, HCC is considered resistant to systemic chemotherapy (25). Sorafenib is the first systematic treatment to increase the survival rates of patients with advanced liver cancer (26). Although sorafenib has been proven effective and safe for HCC treatment, its potential role as an adjuvant for HCC is still controversial (27). According to the results of recent clinical trials, a single drug may not be sufficient to treat liver cancer (28). Therefore, combined therapy is the main strategy for treating advanced liver cancer systemically. In addition, it is important to find new therapeutic targets. With the continuous development of new treatment strategies, HCC outcomes are expected to be greatly improved in the future.
JAK/STAT signaling pathway is considered among the most important signal-cascading pathways in many cellular processes and is initiated by growth factors, cytokines, hormones, and other ligands. The JAK/STAT signal modulates several physiological mechanisms, including immunomodulation, cell proliferation, cell survival, apoptosis of myeloid and non-myeloid cells, and hematopoiesis (29). JAK/STAT is an evolutionarily conserved signal transduction pathway in all eukaryotes and the main signal transduction pathway for mammalian cytokines and growth factors, converting extracellular signals into transcriptional information to regulate physiological processes (30). Abnormal activation of intracellular signaling pathways causes cells with genetic and metabolic changes to show malignant phenotypes (31). Numerous changes are observed in signaling pathways regulating cell growth, division, death, fate, movement, tumor microenvironment, angiogenesis, and inflammation (32). In addition to the regulation of physiological processes, alterations in this signaling pathway also function in some pathophysiological diseases, including malignant tumors. Research has shown the activation of this pathway in multiple cancers, including HCC. Multiple effects of this pathway on cells and its association with other signaling pathways are key markers for the onset and development of cancer. Therefore, targeted activation of this pathway is a reasonable strategy for treating tumors (33). This pathway regulates various biological processes. However, it is particularly important for cell division, cell death (34), and tumor formation (35). Many mechanisms regulate JAK2/STAT3 signal transduction, in which tyrosine kinases are crucial. The synergistic effect of these mechanisms ensures optimal cell function during normal physiology and prevents inappropriate cell activities associated with the onset of diseases, such as tumors (36). STAT3 promotes cell invasion by adjusting matrix metalloproteinases (MMP) expression, such as MMP-2 and MMP-9 MMP (37). In addition, STAT3 also causes enhancement of HCC cell invasion via upregulation of the expression of Slug, Twist, and other epithelial-mesenchymal transition proteins (38). Other pathways involved in tumor metastasis, such as the PI3K/Akt2 signaling pathway, are also regulated by STAT3, which can synergistically increase tumor invasiveness (39). It is considered a strong candidate gene for the promotion of tumorigenesis in many human cancers, including the HCC (40). As a transcription factor, STAT3 stimulation promotes the expression of multiple genes, producing plenty of cancer markers. This finding highlights the carcinogenic role of STAT3 in the HCC (33). STAT3 is related to the adaptation of cancer cell metabolic processes to produce large amounts of energy biomolecules that promote cell survival (41). Therefore, JAK2/STAT3 signaling pathway is a vital regulator of liver cancer onset and advancement and may become a potential target for future clinical treatment.
This research observed that CLDN6 knockdown could reduce the invasive and migratory capacities of cells and inhibit JAK2/STAT3 signaling pathway activation. A particular effect was observed on p-JAK2 and p-STAT3 proteins, which was characterized by reduced expression levels of these proteins and increased apoptosis. The expression of anti-apoptotic protein Bcl-2 was decreased, and cleaved caspase-3 and Bax expression were contrasting. Conversely, CLDN6 overexpression can prevent apoptosis while promoting cell migration and invasion. The increase in Bcl2 expression level and the lowered expression levels of Bax and cleaved caspase-3 backed this hypothesis. Collectively, these findings are indicative of the involvement of CLDN6 in HCC progression by regulating the JAK2/STAT3 signaling pathway.
Conclusions
In conclusion, CLDN6 is a promising treatment target for HCC, but subsequent research is required to completely understand its underlying mechanisms and associated adverse effects to enhance therapeutic efficiency and potential individualized patient therapy. The JAK2/STAT3 signaling pathway’s diverse cellular effects, as well as how it interacts with other signaling pathways, are crucial for the onset and progression of cancers. Therefore, targeted activation JAK2/STAT3 pathway is a reasonable strategy to treat tumors with the modulation of this abnormal signaling pathway. The findings of this study demonstrate that the downregulation of CLDN6 is sufficient for the reduction of the invading and migrating ability of HCC cells and ultimately resulting in the death of HCC cells by inactivating the JAK2/STAT3 signaling pathway. This suggests that CLDN6 may be a biomarker of HCC with potential value as a therapeutic target.
Acknowledgments
Funding: This study was funded by
Footnote
Reporting Checklist: The authors have completed the MDAR reporting checklist. Available at https://tcr.amegroups.com/article/view/10.21037/tcr-23-19/rc
Data Sharing Statement: Available at https://tcr.amegroups.com/article/view/10.21037/tcr-23-19/dss
Peer Review File: Available at https://tcr.amegroups.com/article/view/10.21037/tcr-23-19/prf
Conflicts of Interest: All authors have completed the ICMJE uniform disclosure form (available at https://tcr.amegroups.com/article/view/10.21037/tcr-23-19/coif). The authors have no conflicts of interest to declare.
Ethical Statement: The authors are accountable for all aspects of the work in ensuring that questions related to the accuracy or integrity of any part of the work are appropriately investigated and resolved.
Open Access Statement: This is an Open Access article distributed in accordance with the Creative Commons Attribution-NonCommercial-NoDerivs 4.0 International License (CC BY-NC-ND 4.0), which permits the non-commercial replication and distribution of the article with the strict proviso that no changes or edits are made and the original work is properly cited (including links to both the formal publication through the relevant DOI and the license). See: https://creativecommons.org/licenses/by-nc-nd/4.0/.
References
- Torre LA, Bray F, Siegel RL, et al. Global cancer statistics, 2012. CA Cancer J Clin 2015;65:87-108. [Crossref] [PubMed]
- Mineta K, Yamamoto Y, Yamazaki Y, et al. Predicted expansion of the claudin multigene family. FEBS Lett 2011;585:606-12. [Crossref] [PubMed]
- Osanai M, Takasawa A, Murata M, et al. Claudins in cancer: bench to bedside. Pflugers Arch 2017;469:55-67. [Crossref] [PubMed]
- Zihni C, Mills C, Matter K, et al. Tight junctions: from simple barriers to multifunctional molecular gates. Nat Rev Mol Cell Biol 2016;17:564-80. [Crossref] [PubMed]
- Krause G, Winkler L, Mueller SL, et al. Structure and function of claudins. Biochim Biophys Acta 2008;1778:631-45. [Crossref] [PubMed]
- Kwon MJ, Kim SH, Jeong HM, et al. Claudin-4 overexpression is associated with epigenetic derepression in gastric carcinoma. Lab Invest 2011;91:1652-67. [Crossref] [PubMed]
- Li J, Ananthapanyasut W, Yu AS. Claudins in renal physiology and disease. Pediatr Nephrol 2011;26:2133-42. [Crossref] [PubMed]
- Hu CA, Hou Y, Yi D, et al. Autophagy and tight junction proteins in the intestine and intestinal diseases. Anim Nutr 2015;1:123-7. [Crossref] [PubMed]
- English DP, Santin AD. Claudins overexpression in ovarian cancer: potential targets for Clostridium Perfringens Enterotoxin (CPE) based diagnosis and therapy. Int J Mol Sci 2013;14:10412-37. [Crossref] [PubMed]
- Ushiku T, Shinozaki-Ushiku A, Maeda D, et al. Distinct expression pattern of claudin-6, a primitive phenotypic tight junction molecule, in germ cell tumours and visceral carcinomas. Histopathology 2012;61:1043-56. [Crossref] [PubMed]
- Anderson WJ, Zhou Q, Alcalde V, et al. Genetic targeting of the endoderm with claudin-6CreER. Dev Dyn 2008;237:504-12. [Crossref] [PubMed]
- Zavala-Zendejas VE, Torres-Martinez AC, Salas-Morales B, et al. Claudin-6, 7, or 9 overexpression in the human gastric adenocarcinoma cell line AGS increases its invasiveness, migration, and proliferation rate. Cancer Invest 2011;29:1-11. [Crossref] [PubMed]
- Micke P, Mattsson JS, Edlund K, et al. Aberrantly activated claudin 6 and 18.2 as potential therapy targets in non-small-cell lung cancer. Int J Cancer 2014;135:2206-14. [Crossref] [PubMed]
- Wang L, Jin X, Lin D, et al. Clinicopathologic significance of claudin-6, occludin, and matrix metalloproteinases -2 expression in ovarian carcinoma. Diagn Pathol 2013;8:190. [Crossref] [PubMed]
- Cao X, He GZ. Knockdown of CLDN6 inhibits cell proliferation and migration via PI3K/AKT/mTOR signaling pathway in endometrial carcinoma cell line HEC-1-B. Onco Targets Ther 2018;11:6351-60. [Crossref] [PubMed]
- Tsunoda S, Smith E, De Young NJ, et al. Methylation of CLDN6, FBN2, RBP1, RBP4, TFPI2, and TMEFF2 in esophageal squamous cell carcinoma. Oncol Rep 2009;21:1067-73. [Crossref] [PubMed]
- Zhang X, Ruan Y, Li Y, et al. Expression of apoptosis signal-regulating kinase 1 is associated with tight junction protein claudin-6 in cervical carcinoma. Int J Clin Exp Pathol 2015;8:5535-41. [PubMed]
- Jia H, Chai X, Li S, et al. Identification of claudin-2, -6, -11 and -14 as prognostic markers in human breast carcinoma. Int J Clin Exp Pathol 2019;12:2195-204. [PubMed]
- Du H, Yang X, Fan J, et al. Claudin 6: Therapeutic prospects for tumours, and mechanisms of expression and regulation Mol Med Rep 2021;24:677. (Review). [Crossref] [PubMed]
- Yafang L, Qiong W, Yue R, et al. Role of Estrogen Receptor-alpha in the Regulation of Claudin-6 Expression in Breast Cancer Cells. J Breast Cancer 2011;14:20-7. [Crossref] [PubMed]
- Wang Q, Zhang Y, Zhang T, et al. Low claudin-6 expression correlates with poor prognosis in patients with non-small cell lung cancer. Onco Targets Ther 2015;8:1971-7. [PubMed]
- Bray F, Ferlay J, Soerjomataram I, et al. Global cancer statistics 2018: GLOBOCAN estimates of incidence and mortality worldwide for 36 cancers in 185 countries. CA Cancer J Clin 2018;68:394-424. [Crossref] [PubMed]
- Zhang H, Zhang W, Jiang L, et al. Recent advances in systemic therapy for hepatocellular carcinoma. Biomark Res 2022;10:3. [Crossref] [PubMed]
- Fattovich G, Stroffolini T, Zagni I, et al. Hepatocellular carcinoma in cirrhosis: incidence and risk factors. Gastroenterology 2004;127:S35-50. [Crossref] [PubMed]
- Grandhi MS, Kim AK, Ronnekleiv-Kelly SM, et al. Hepatocellular carcinoma: From diagnosis to treatment. Surg Oncol 2016;25:74-85. [Crossref] [PubMed]
- Llovet JM, Castet F, Heikenwalder M, et al. Immunotherapies for hepatocellular carcinoma. Nat Rev Clin Oncol 2022;19:151-72. [Crossref] [PubMed]
- Bruix J, Takayama T, Mazzaferro V, et al. Adjuvant sorafenib for hepatocellular carcinoma after resection or ablation (STORM): a phase 3, randomised, double-blind, placebo-controlled trial. Lancet Oncol 2015;16:1344-54. [Crossref] [PubMed]
- Faivre S, Rimassa L, Finn RS. Molecular therapies for HCC: Looking outside the box. J Hepatol 2020;72:342-52. [Crossref] [PubMed]
- Rah B, Rather RA, Bhat GR, et al. JAK/STAT Signaling: Molecular Targets, Therapeutic Opportunities, and Limitations of Targeted Inhibitions in Solid Malignancies. Front Pharmacol 2022;13:821344. [Crossref] [PubMed]
- O'Shea JJ, Schwartz DM, Villarino AV, et al. The JAK-STAT pathway: impact on human disease and therapeutic intervention. Annu Rev Med 2015;66:311-28. [Crossref] [PubMed]
- Flavahan WA, Gaskell E, Bernstein BE. Epigenetic plasticity and the hallmarks of cancer. Science 2017;357:eaal2380. [Crossref] [PubMed]
- Jing X, Yang F, Shao C, et al. Role of hypoxia in cancer therapy by regulating the tumor microenvironment. Mol Cancer 2019;18:157. [Crossref] [PubMed]
- Hin Tang JJ, Hao Thng DK, Lim JJ, et al. JAK/STAT signaling in hepatocellular carcinoma. Hepat Oncol 2020;7:HEP18. [Crossref] [PubMed]
- Park SY, Lee CJ, Choi JH, et al. The JAK2/STAT3/CCND2 Axis promotes colorectal Cancer stem cell persistence and radioresistance. J Exp Clin Cancer Res 2019;38:399. [Crossref] [PubMed]
- Kiu H, Nicholson SE. Biology and significance of the JAK/STAT signalling pathways. Growth Factors 2012;30:88-106. [Crossref] [PubMed]
- Mengie Ayele T, Tilahun Muche Z, Behaile Teklemariam A, et al. Role of JAK2/STAT3 Signaling Pathway in the Tumorigenesis, Chemotherapy Resistance, and Treatment of Solid Tumors: A Systemic Review. J Inflamm Res 2022;15:1349-64. [Crossref] [PubMed]
- Huang L, Jian Z, Gao Y, et al. RPN2 promotes metastasis of hepatocellular carcinoma cell and inhibits autophagy via STAT3 and NF-κB pathways. Aging (Albany NY) 2019;11:6674-90. [Crossref] [PubMed]
- Kang FB, Wang L, Jia HC, et al. B7-H3 promotes aggression and invasion of hepatocellular carcinoma by targeting epithelial-to-mesenchymal transition via JAK2/STAT3/Slug signaling pathway. Cancer Cell Int 2015;15:45. [Crossref] [PubMed]
- Xie Y, Li J, Zhang C. STAT3 promotes the proliferation and migration of hepatocellular carcinoma cells by regulating AKT2. Oncol Lett 2018;15:3333-8. [PubMed]
- Khan AQ, Ahmed EI, Elareer NR, et al. Role of miRNA-Regulated Cancer Stem Cells in the Pathogenesis of Human Malignancies. Cells 2019;8:840. [Crossref] [PubMed]
- Huynh J, Chand A, Gough D, et al. Therapeutically exploiting STAT3 activity in cancer - using tissue repair as a road map. Nat Rev Cancer 2019;19:82-96. [Crossref] [PubMed]