Establishing a prognostic model with ferroptosis-related long non-coding RNAs in bladder cancer
Highlight box
Key findings
• This study identified ferroptosis-associated lncRNAs that could help predicting the BLCA prognosis and provide some clinical information for the treatment of patients with BLCA.
What is known and what is new?
• Tumor metabolic reprogramming is associated with acquired sensitivity to ferroptosis.
• This study may reveal potentially valuable biomarkers and relevant therapeutic targets in ferroptosis-related signaling pathways.
What is the implication, and what should change now?
• This study results have not been verified by clinical trials. Therefore, it is recommended that basic experiments be carried out in the future to validate the function of ferroptosis-accosiated lncRNAs.
Introduction
Bladder cancer (BLCA) is considered one of the prominent forms of cancer worldwide, with an estimated annual incidence of around 550,000 new cases (1). Due to its high propensity for recurrence and metastasis, BLCA patients suffer tremendous pain and financial burden (2). For patients with the same graded stage of BLCA, survival and prognosis may differ for each patient, even if they receive the same treatment. In addition, the lack of early cancer markers or clinical prediction models limits the effectiveness of clinical treatment for BLCA. Therefore, it is particularly important to find reliable molecular biological markers and targets for the treatment.
Ferroptosis is a newly identified type of programmed cell death that is reliant on iron and is triggered by lipid peroxidation and fatal reactive oxygen species (ROS) (3). Ferroptosis is distinct from other cell death types, including apoptosis, necrosis, and autophagy (4). Moreover, ferroptosis was found to exhibit a close association with tumorigenesis and progression (5). The activation of the ferroptosis pathway presents a novel approach to investigating affective tumor therapeutic options (6). Long non-coding RNAs (lncRNAs) are non-coding RNA molecules that are around 200 nucleotides in length, govern diverse cellular processes of both physiological and biochemical nature by mediating chromosomal modifications, transcriptional activation, and interference (7,8). Recent studies have reported that ferroptosis-associated lncRNAs are involved in tumorigenesis, progression, and metastasis (8-10). Nonetheless, limited research has been conducted on ferroptosis-associated lncRNA profile in patients and its correlation with their overall survival (OS). This current study initially established several distinct prognostic signatures of lncRNA that are differentially expressed lncRNA and correlated to ferroptosis, utilizing data from The Cancer Genome Atlas (TCGA). Subsequently, this research explored the function of ferroptosis-associated mRNA, N6-methyladenosine (m6A) mRNA status, and immune responses in BLCA prognosis. We present this article in accordance with the TRIPOD reporting checklist (available at https://tcr.amegroups.com/article/view/10.21037/tcr-23-194/rc).
Methods
Data collection
The mRNA expression profiles of BLCA patients (19 normal and 414 tumor cases) and clinical datasets from the TCGA dataset were downloaded. The current study was conducted in accordance with the Declaration of Helsinki (as revised in 2013). The study included clinical data pertaining to survival time, survival status, gender, age, T (tumor) stage, N (tumor) stage, M (metastasis) stage, and TNM stage of the patients. FerrDb (http://www.zhounan.org/ferrdb/current/) was accessed to download Ferroptosis-related genes. Subsequently, the study employed Pearson correlation analysis to evaluate ferroptosis-related lncRNAs and BLCA association. Statistical significance was established for the correlation when the correlation coefficient value was at least |R2| >0.4 (P<0.001). Differences in the expression of lncRNAs associated with ferroptosis were significant at false discovery rate (FDR) <0.05 and |log2 fold-change (FC)| ≥1 (P<0.05). The present study conducted an analysis of the roles played by differentially-expressed genes (DEGs) associated with ferroptosis, both those that were up-regulated and those that were down-regulated. Additionally, gene ontology (GO) was employed for the evaluation of the DEG-associated biological pathways. Utilizing the data sourced from the Kyoto Encyclopedia of Genes and Genomes (KEGG), the biological processes (BP), molecular functions (MF), and cellular components (CC), the fact that BP, CC, and MF are the main categories/sub-ontologies of GO regulated by differentially-expressed ferroptosis-associated lncRNAs were functionally analyzed through the R software, clusterProfiler, org.Hs.eg.db, enrichplot, and ggplot2 package based on the basis of KEGG data.
A prognostic model of ferroptosis-related lncRNAs
The correlation between ferroptosis-associated lncRNA and survival was assessed with a one-way Cox regression analysis, independent of the cutoff used. Risk scores were analyzed by calculating risk scores (11) for each BLCA patient, and the scores were ranked in order from highest to lowest and were divided into high- and low-risk groups.
Column line diagram and predictive signature
To investigate the correlation between risk scores, associated clinicopathological features, and OS of patients with BLCA, columnar plots, including age, gender, grade, stage, TNM classification, and risk score, were utilized for calculating the overall score and predicting the survival at 1, 3, and 5 years.
Immunoassay and gene expression
This research evaluated the two risk groups by comparing the algorithms of TIMER (12), CIBERSORT (13,14), CIBERSORT-ABS, QUANTISEQ, MCP Conter (15), XCELL, and EPIC, combined with the features of ferroptosis-associated lncRNAs, and used heat maps to demonstrate the differences in immune responses under different algorithms and analyze their potential immune checkpoints and the gene expression associated with m6A in BLCA.
Statistical analysis
The statistical analyses were conducted utilizing R statistical software version 4.1.1 and Strawberry Perl version 5.32.1.1. The Benjamini-Hochberg method was utilized for the identification of differentially-expressed lncRNAs. The receiver operating characteristic (ROC) curves and decision curve analysis (DCA) were utilized for assessing the prognostic marker sensitivity and specificity for BLCA in comparison to other clinicopathologic. The study employed univariate and multifactorial Cox regression models to evaluate risk ratios and 95% confidence intervals. A difference was deemed statistically significant at P<0.05.
Results
GO and KEGG enrichment analysis of genes related to ferroptosis
Sixty-one DEGs associated with ferroptosis (25 suppressed and 36 overexpressed) were identified. GO: BP revealed that the endogenous apoptotic signaling pathways, responses to steroid hormones and oxidative stress are involved in. MF mainly regulates DNA binding transcriptional repressive activity, RNA polymerase II specificity, and iron ion binding; CC mainly up-regulates basolateral plasma membrane, endoplasmic reticulum lumen, and heterochromatin. The KEGG-based analysis revealed that up-regulated genes exhibited main involvement in microRNA synthesis in cancer cells, human cytomegalovirus infection, multiple pathways, including the PI3K-Akt, the TNF, IL-17, P53, and the HIF signaling pathways, as well as ferroptosis (Figure 1A,1B).
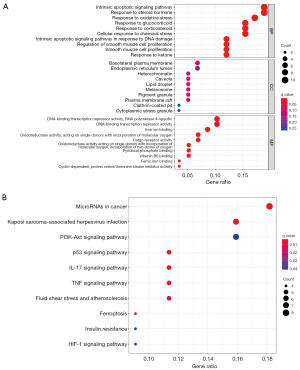
A prognostic model of lncRNAs based on ferroptosis
Single-factor COX analysis identified 151 ferroptosis-associated lncRNAswith ferroptosis, of which 36 of these lncRNAs were collected in the multi-factor COX analysis. These 36 differentially-expressed lncRNAs were AC010973.2, ZFHX2-AS1, PTOV1-AS2, AC020765.2, AC008105.1, etc. The risk score was then calculated and a prognostic model for lncRNAs was built.
Survival analysis and multi-factor test
The patients were categorized into two distinct groups, namely high-risk and low-risk, using the median risk scores as the criterion. Kaplan-Meier (K-M) curves showed a poorer prognosis in the high-risk group (P<0.001, Figure 2A). Subsequently, this research performed ROC and DCA curves to evaluate the risk score model accuracy (AUC value was 0.810), both showing good accuracy (Figure 2B). Survival time (in years), survival status, and risk score of each patient are shown in Figure 2C. In the ROC curve analysis over time, the AUC predictions of the new lncRNAs models for 1-, 2-, and 3-year survival were 0.810, 0.796, and 0.781, respectively (Figure 2D). As the risk score of BLCA patients increased, most of the new lncRNAs identified were negatively correlated with our risk model (Figure 2E). As the risk score increases, patients with high-risk scores exhibited higher mortality rates compared to those with low-risk scores. The heat map represents the expression profile of 36 lncRNAs (Figure 2F).
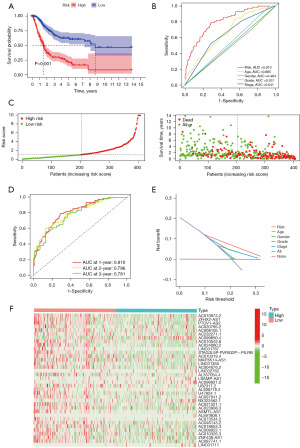
The univariate and multifactorial Cox analyses results revealed that the lncRNAs model (hazard ratio (HR): 1.026; 95% CI: 1.016–1.037), tumor stage (HR: 1.657; 95% CI: 1.360–2.018), age (HR: 1.030; 95% CI: 1.014–1.047) were independent prognostic factors which affect the survival of patients with BLCA (Figure 3A,3B). Figure 3C illustrates the lncRNA and mRNA correlation. This research further analyzed the heat map of the relationship between prognostic models of ferroptosis-related lncRNAs and clinicopathological manifestations (Figure 4A). The hybrid column line plot combining clinicopathological features and the new prognostic model of ferroptosis-associated lncRNAs (Figure 4B) showed better stability and accuracy, and can be utilized to clinically guide the treatment of patients with BLCA.
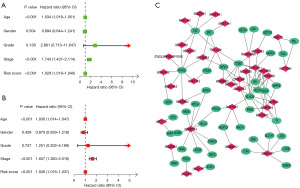
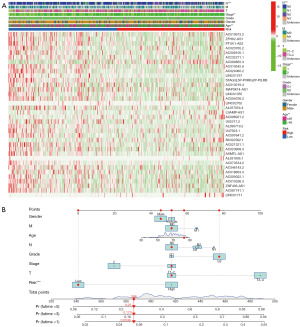
Immune responses and gene expression
Figure 5A shows the heat map of immune responses on the basis of TIMER, CIBERSORT, CIBERSORT-ABS, QUANTISEQ, MCP Counter, XCELL, and EPIC calculations. In light of the significance of immunotherapy based on checkpoint inhibitors, this study delved deeper into the differences in immune checkpoint expressions between the two groups. This analysis yielded significantly different expressions of PDCD1LG2, TNFRSF25, CD276, TMIGD2, NRP1, TNFSF15, TNFSF4, and LGALS9 in the two risk groups (Figure 5B). The comparison of m6A-associated mRNA expression between the two groups suggested significantly different expression of METTL3, YTHDC2, FTO, RBM15, ALKBH5, and YTHDC1 (Figure 5C).
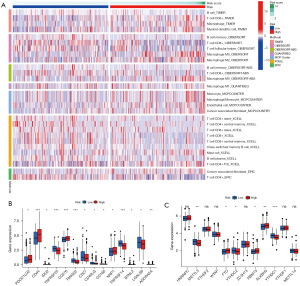
Discussion
In some cases, tumor metabolic reprogramming is associated with acquired sensitivity to ferroptosis, and therefore, a better understanding of the processes involved in regulating ferroptosis will ultimately help to discover new therapeutic strategies to improve cancer treatment. lncRNAs and microRNAs are increasingly recognized as key mediators of ferroptosis regulation (16). This study first identified lncRNAs from the TCGA database as novel prognostic markers associated with ferroptosis. Next, this research the role of immune checkpoint inhibitors (ICIs) in BLCA in two risk groups. This study reveals potentially valuable biomarkers and relevant therapeutic targets in ferroptosis-related signaling pathways.
Overall, this analysis identified 61 differentially-expressed DEGs, which were mainly involved in microRNA synthesis and multiple signaling pathways, including PI3K-Akt, TNF, IL-17, P53, and HIF signaling pathways in cancer cells in GO and KEGG enrichment analysis. Recent studies have shown that p53 suppresses cystine uptake and sensitizes cells to ferroptosis by downregulating SLC7A11, a key component of the cystine/glutamate reverse transporter protein (17-19). Miess et al. (20) found that the deletion of MT1DP resulted in stabilizing the hypoxia-inducible factors HIF-1/2α. Restoration of functional VHL by exogenous pVHL expression restores oxidative metabolism in renal cell carcinomas and renders them insensitive to ferroptosis induction. Additionally, abnormalities in suppressing the PI3K-AKT-mTOR signaling axis make cancer cells susceptible to ferroptosis activation, and high-frequency events in human carcinoid tumors impart ferroptosis resistance to cancer cells (21). Thirty-six differentially-expressed lncRNAs were screened in the analytical study as independent prognostic factors for BLCA. These 36 ferroptosis-associated lncRNAs have not been further experimentally studied in BLCA, and the prognostic role in BLCA is unclear. These findings also provide us with new ideas for the next step in studying the treatment of BLCA.
This research classified differentially-expressed ferroptosis-associated lncRNAs into high- and low- risk, groups, according to their scores, for the purpose of exploring potential role in BLCA. Recent studies have shown significant clinical success of immune checkpoint inhibitors (ICIs) in cancer immunotherapy (22), but only one-third of the patients respond to the ICIs (23,24). The combination of ferroptosis and ICIs synergistically enhances antitumor activity, even in ICI-resistant types (22). Few studies are available on the association between ferroptosis and ICIs. Gai et al. (25) showed that MT1DP could modulate the miR-365a-3p/NRF2 axis to improve the sensitivity of elaterin-induced ferroptosis in non-small cell lung cancer. Additionally, the research conducted by Jiang et al. (26) revealed that the TYRO3 oncogene had the capacity to decrease the occurrence of ferroptosis induced by anti-PD-1/PD-L1 in tumor cells. This, in turn, resulted in resistance to anti-PD-1/PD-L1 therapy. Consequently, the suppression of the TYRO3 oncogene promoted tumor ferroptosis and enhanced the susceptibility of resistant tumors to anti-PD-1 therapy. Meanwhile, some recent studies have identified regulators of ferroptosis, including the ATF3/4, SLC7A11, BECN1 pathway, and P53 signaling pathways (27-30).
Ferroptosis is a new type of cell death type, and the rapid advance in ferroptosis in tumors also provides new opportunities and challenges for tumor treatment. However, the tumor microenvironment is extremely complex, just as the interrelationship between ferroptosis and host immunogenicity is still unclear, and many questions still need to be further explored. This study identified ferroptosis-associated lncRNAs that could help predict the prognosis of BLCA and provide some clinical information for the treatment of BLCA patients. Nevertheless, because this study was only limited to the TCGA database and the model needed to be further validated in basic experiments and clinical samples, there are limitations in the application of these findings. In the future, this team will focus on the mechanistic study of ferroptosis-associated lncRNAs to provide new ideas for the therapeutic approach of BLCA patients.
Conclusions
The expression of ferroptosis-associated lncRNAs is correlated to BLCA prognosis. According to the K-M curve results, patients with high-risk lncRNAs have a poor prognosis. This risk assessment model is more effective than traditional clinicopathological features in predicting BLCA prognosis and can provide a basis for early clinical diagnosis and screening of prognostic markers in patients with BLCA.
Acknowledgments
Funding: This study was supported by
Footnote
Reporting Checklist: The authors have completed the TRIPOD reporting checklist. Available at https://tcr.amegroups.com/article/view/10.21037/tcr-23-194/rc
Peer Review File: Available at https://tcr.amegroups.com/article/view/10.21037/tcr-23-194/prf
Conflicts of Interest: All authors have completed the ICMJE uniform disclosure form (available at https://tcr.amegroups.com/article/view/10.21037/tcr-23-194/coif). The authors have no conflicts of interest to declare.
Ethical Statement: The authors are accountable for all aspects of the work in ensuring that questions related to the accuracy or integrity of any part of the work are appropriately investigated and resolved. The study was conducted in accordance with the Declaration of Helsinki (as revised in 2013).
Open Access Statement: This is an Open Access article distributed in accordance with the Creative Commons Attribution-NonCommercial-NoDerivs 4.0 International License (CC BY-NC-ND 4.0), which permits the non-commercial replication and distribution of the article with the strict proviso that no changes or edits are made and the original work is properly cited (including links to both the formal publication through the relevant DOI and the license). See: https://creativecommons.org/licenses/by-nc-nd/4.0/.
References
- Richters A, Aben KKH, Kiemeney LALM. The global burden of urinary bladder cancer: an update. World J Urol 2020;38:1895-904. [Crossref] [PubMed]
- Lu JL, Xia QD, Lu YH, et al. Efficacy of intravesical therapies on the prevention of recurrence and progression of non-muscle-invasive bladder cancer: A systematic review and network meta-analysis. Cancer Med 2020;9:7800-9. Erratum in: Cancer Med 2021;10:5722. [Crossref] [PubMed]
- Su Y, Liu Z, Xie K, et al. Ferroptosis: A Novel Type of Cell Death in Male Reproduction. Genes (Basel) 2022;14:43. [Crossref] [PubMed]
- Chen X, Kang R, Kroemer G, et al. Broadening horizons: the role of ferroptosis in cancer. Nat Rev Clin Oncol 2021;18:280-96. [Crossref] [PubMed]
- Hassannia B, Vandenabeele P, Vanden Berghe T. Targeting Ferroptosis to Iron Out Cancer. Cancer Cell 2019;35:830-49. [Crossref] [PubMed]
- Cheng X, Wang Y, Li Y, et al. Quantification of ferroptosis pattern in bladder carcinoma and its significance on immunotherapy. Sci Rep 2022;12:9066. [Crossref] [PubMed]
- Gao N, Li Y, Li J, et al. Long Non-Coding RNAs: The Regulatory Mechanisms, Research Strategies, and Future Directions in Cancers. Front Oncol 2020;10:598817. [Crossref] [PubMed]
- Gil N, Ulitsky I. Regulation of gene expression by cis-acting long non-coding RNAs. Nat Rev Genet 2020;21:102-17. [Crossref] [PubMed]
- Qu L, He X, Tang Q, et al. Iron metabolism, ferroptosis, and lncRNA in cancer: knowns and unknowns. J Zhejiang Univ Sci B 2022;23:844-62. [Crossref] [PubMed]
- Lian J, Zhang C, Lu H. A Ferroptosis-Related LncRNA Signature Associated with Prognosis, Tumor Immune Environment, and Genome Instability in Hepatocellular Carcinoma. Comput Math Methods Med 2022;2022:6284540. [Crossref] [PubMed]
- Zhu K, Xiaoqiang L, Deng W, et al. Development and validation of a novel lipid metabolism-related gene prognostic signature and candidate drugs for patients with bladder cancer. Lipids Health Dis 2021;20:146. [Crossref] [PubMed]
- Li T, Fan J, Wang B, et al. TIMER: A Web Server for Comprehensive Analysis of Tumor-Infiltrating Immune Cells. Cancer Res 2017;77:e108-10. [Crossref] [PubMed]
- Newman AM, Liu CL, Green MR, et al. Robust enumeration of cell subsets from tissue expression profiles. Nat Methods 2015;12:453-7. [Crossref] [PubMed]
- Charoentong P, Finotello F, Angelova M, et al. Pan-cancer Immunogenomic Analyses Reveal Genotype-Immunophenotype Relationships and Predictors of Response to Checkpoint Blockade. Cell Rep 2017;18:248-62. [Crossref] [PubMed]
- Shi J, Jiang D, Yang S, et al. LPAR1, Correlated With Immune Infiltrates, Is a Potential Prognostic Biomarker in Prostate Cancer. Front Oncol 2020;10:846. [Crossref] [PubMed]
- Mou Y, Wang J, Wu J, et al. Ferroptosis, a new form of cell death: opportunities and challenges in cancer. J Hematol Oncol 2019;12:34. [Crossref] [PubMed]
- Jiang L, Kon N, Li T, et al. Ferroptosis as a p53-mediated activity during tumour suppression. Nature 2015;520:57-62. [Crossref] [PubMed]
- Yang Y, Ma Y, Li Q, et al. STAT6 inhibits ferroptosis and alleviates acute lung injury via regulating P53/SLC7A11 pathway. Cell Death Dis 2022;13:530. [Crossref] [PubMed]
- Guan Z, Chen J, Li X, et al. Tanshinone IIA induces ferroptosis in gastric cancer cells through p53-mediated SLC7A11 down-regulation. Biosci Rep 2020;40:BSR20201807. [Crossref] [PubMed]
- Miess H, Dankworth B, Gouw AM, et al. The glutathione redox system is essential to prevent ferroptosis caused by impaired lipid metabolism in clear cell renal cell carcinoma. Oncogene 2018;37:5435-50. [Crossref] [PubMed]
- Yi J, Zhu J, Wu J, et al. Oncogenic activation of PI3K-AKT-mTOR signaling suppresses ferroptosis via SREBP-mediated lipogenesis. Proc Natl Acad Sci U S A 2020;117:31189-97. [Crossref] [PubMed]
- Tang R, Xu J, Zhang B, et al. Ferroptosis, necroptosis, and pyroptosis in anticancer immunity. J Hematol Oncol 2020;13:110. [Crossref] [PubMed]
- Zhang Z, Xu Z, Yan Y. Role of a Pyroptosis-Related lncRNA Signature in Risk Stratification and Immunotherapy of Ovarian Cancer. Front Med (Lausanne) 2022;8:793515. [Crossref] [PubMed]
- Liu J, Wang Y, Yin J, et al. Pan-Cancer Analysis Revealed SRSF9 as a New Biomarker for Prognosis and Immunotherapy. J Oncol 2022;2022:3477148. [Crossref] [PubMed]
- Gai C, Liu C, Wu X, et al. MT1DP loaded by folate-modified liposomes sensitizes erastin-induced ferroptosis via regulating miR-365a-3p/NRF2 axis in non-small cell lung cancer cells. Cell Death Dis 2020;11:751. [Crossref] [PubMed]
- Jiang Z, Lim SO, Yan M, et al. TYRO3 induces anti-PD-1/PD-L1 therapy resistance by limiting innate immunity and tumoral ferroptosis. J Clin Invest 2021;131:e139434. [Crossref] [PubMed]
- Song X, Long D. Nrf2 and Ferroptosis: A New Research Direction for Neurodegenerative Diseases. Front Neurosci 2020;14:267. [Crossref] [PubMed]
- Li Y, Yan J, Zhao Q, et al. ATF3 promotes ferroptosis in sorafenib-induced cardiotoxicity by suppressing Slc7a11 expression. Front Pharmacol 2022;13:904314. [Crossref] [PubMed]
- Li Q, Li K, Guo Q, et al. CircRNA circSTIL inhibits ferroptosis in colorectal cancer via miR-431/SLC7A11 axis. Environ Toxicol 2023;38:981-9. [Crossref] [PubMed]
- Tan Y, Huang Y, Mei R, et al. HucMSC-derived exosomes delivered BECN1 induces ferroptosis of hepatic stellate cells via regulating the xCT/GPX4 axis. Cell Death Dis 2022;13:319. [Crossref] [PubMed]