A STEP ahead for CAR-T cell therapy of large B cell lymphoma: understanding the molecular determinants of resistance
Large B cell lymphomas (LBCLs) are classified as a heterogeneous group of hematologic malignancies characterized by the proliferation of malignant mature B cells in nodal or extra-nodal anatomical sites (1). Diffuse large B cell lymphoma (DLBCL) represents the most common histologic type of LBCL and is the most frequent lymphoma in the adult population (1). A significant fraction of LBCL relapse after achieving an initial complete response or, in the case of refractoriness, fail to adequately respond to first line treatment. Due to their aggressive behavior and acquired resistance to available therapies, relapsed/refractory (r/r) LBCLs are still a major unmet medical need in the clinical practice of hematology (2).
Chimeric antigen receptor (CAR)-T cells are a therapeutic cellular product obtained by the transduction of a genetically engineered CAR into autologous T cells obtained from the patient (3). The CAR-T cell therapy procedure encompasses multiple stages: (I) initially, the patient undergoes leukapheresis to collect T cells that will be engineered; (II) the collected T cells are genetically modified through the addition of a CAR sequence with a viral or non-viral approach; (III) the obtained CAR-T cells are amplified in vitro; (IV) a lymphodepleting regimen is administered to the patient, in order to obtain a favorable immune environment for the proper expansion and persistence of CAR-T cells; (V) CAR-T cells are infused to the patient (4). CARs can be developed to target surface antigens of the tumor in order to activate the T cell cytotoxic response. In the case of LBCLs, currently available CAR-T cells in the clinical practice are designed to react against the surface antigen CD19 (5). The first CAR-T cells approved for r/r LBCLs, in particular for DLBCL, include axicabtagene ciloleucel (axi-cel) and tisagenlecleucel (tisa-cel), both targeting the CD19 antigen on the cell surface of neoplastic cells (6,7).
Although CAR-T cells directed against CD19 (CAR19 T cells) have shown therapeutic activity in 50–80% of r/r LBCL patients, approximately 60% of these cases will eventually experience disease progression and relapse (8-11). The mechanisms of resistance to CAR-T cells are most likely due to multiple factors, that may involve both the target lymphoma cells as well as the immune effector cells of the host. In more detail, the resistance mechanisms characterized to date include alterations of the tumor microenvironment, the loss of the expression of the CD19 antigen on the lymphoma cell surface, the properties and functionality of the CAR19 T cells themselves, as well as the function and potential exhaustion of the immune system of the host (12). Despite a certain advancement in the field, at present the resistance mechanisms to CAR19 T cells are far from being fully understood.
Given the complexity and multiplicity of the mechanisms of resistance to CAR19 T cells in B cell lymphoma patients, a comprehensive analysis of the interactions among all these factors is needed and may help clarify the most appropriate management of CAR19 T cells in the individual patient. On these grounds, Sworder et al. have recently developed a combined diagnostic strategy to define the molecular determinants of resistance to CAR19 T cell therapy in r/r LBCL prior to CAR-T cell infusion as well as after treatment (13).
In the observational study by Sworder et al., a total of 254 LBCL patients were considered (13). Patients had been treated at multiple sites, including Stanford University, MD Anderson Cancer Center, and Essen University Hospital. Of these patients, 115 were treatment-naïve, while the other 139 were r/r LBCL. Histologic subtypes included in the study were composed of DLBCL, T-cell/histiocytic rich large B-cell lymphoma (THRLBCL), high grade B cell lymphoma (HGBCL), primary mediastinal B-cell lymphoma (PMBCL), and transformed follicular lymphoma (TFL), i.e., follicular lymphoma that has histologically transformed to an aggressive B cell lymphoma. Among the 139 r/r cases of LBCL, 65 patients were assigned to the discovery cohort and 73 to the validation cohort, while one case was not evaluable. Importantly, the two cohorts did not significantly differ in terms of rate of progression, event free survival (EFS), and overall survival (OS), rendering them comparable for the analysis in the context of a training-validation approach. For the patients of both the discovery and the validation cohorts, plasma and peripheral blood mononuclear cells (PBMCs) were separated from blood samples collected at different stages of the CAR-T cell therapy procedure: (I) before lymphodepletion therapy; (II) prior to axi-cel infusion; (III) during treatment; and (IV) at relapse, in case of its occurrence. Conversely, tumor biopsies were collected where available in a subgroup of patients, before CAR-T cell therapy and at the time of relapse.
The study took advantage of previous evidence on the role of liquid biopsy in LBCL (14). Liquid biopsy performed on plasma of patients with cancer is a non-invasive approach that, taking advantage of next generation sequencing (NGS), allows to explore multiple biomarkers generated by the analysis of cell free DNA (cfDNA) circulating in plasma, with a specific focus on circulating tumor DNA (ctDNA) (14-18). In the case of LBCL, liquid biopsy has previously allowed the understanding of the spatial molecular heterogeneity of the tumor and the assessment of minimal residual disease that provides a tool for the early prediction of treatment failure in these patients (14-18).
In this context, a major advancement provided by the study of Sworder et al. is represented by the development of the Simultaneous Tumor and Effector Profiling (STEP) platform, which represents a novel tool allowing a comprehensive and integrated analysis of multiple biomarkers related to both the tumor and the host immune system and obtained by NGS applied to plasma samples (Figure 1) (13). One notable feature of STEP is the non-invasive nature of this approach, thus allowing the longitudinal analysis at multiple timepoints during the patient clinical history.
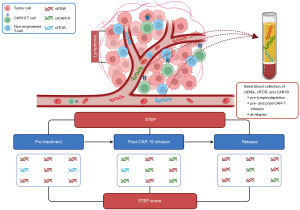
The STEP platform represents an extension of the CAncer Personalized Profiling by deep sequencing (CAPP-seq) and, in particular, combines the analysis of molecules of ctDNA, cell free CAR19 DNA (cfCAR19), and non-engineered T cell receptor cfDNA (cfTCR) (13). Importantly, the design of STEP explores simultaneously three different compartments that are relevant to CAR-T cell therapy: (I) the tumor, identified by ctDNA; (II) the CAR-T cell population, identified by cfCAR19; and (III) other effector T cells of the host, identified by cfTCR. These biomarkers allowed both quantitative investigations, such as the levels of ctDNA and cfCAR19 at multiple timepoints, and qualitative investigations, such as the differences in the mutation profile and tumor genotype of patients according to their response to CAR-T cell treatment.
Consistent with several reports on first line and/or CAR19-treated DLBCL, also in this study the ctDNA levels analyzed longitudinally predicted treatment response both before and after the infusion of CAR19 T cells (13,17,19). In particular, higher levels of ctDNA measured prior to CAR19 treatment and 4 weeks after the infusion were significantly correlated to worse outcomes in both the discovery and validation cohorts. Curiously, the ctDNA thresholds stratifying outcomes after CAR-T cell therapy in r/r LBCL were similar to those predicting EFS also in treatment-naïve DLBCL receiving first line chemoimmunotherapy (17).
Similar to fluorescence activated cell sorting (FACS) analysis of CAR19, the measurement of cfCAR19 retroviral fragments enabled the monitoring of CAR-T cells after infusion (13). Although the two detection methods estimated different CAR19 T cell levels, the two approaches showed a significant correlation, since higher levels detected by FACS were matched with higher levels detected with STEP and vice versa. While cfCAR19 levels were not different between patients responding to treatment versus those who experienced treatment failure, the fragment length of cfCAR19 predicted the grade of tumor infiltration by CAR-T cells. In detail, cfCAR19 molecules were defined as “long” if their length was >310 bp, while the “short” fragments measured <150 bp. This difference in fragment length was associated with a correlation with FACS analysis, suggesting that short cfCAR19 fragments likely originated from CAR-T cells infiltrating the tumor. Indeed, shorter fragments were correlated with lower levels of circulating CAR19 T cells measured with FACS analysis. The presence of shorter fragments of cfCAR19 molecules was probably due to the higher exposure of the CAR-T cell population to nucleases in the tumor microenvironment.
Moreover, in the early post-infusion phase, cfCAR19 levels, representative of CAR-T cells, showed a correlation with levels of cfTCR, representative of non-engineered host effector T cells, but this correlation decreased over time (13). In particular, this finding implies that the populations of CAR-T cells and non-engineered host T cells might expand and/or contract in a different way, have a different spatial distribution, and/or display different responses to the immune stimuli exerted by the neoplasia.
The genomic characteristics of tumor samples at different stages prior to CAR19 infusion and post-treatment were also investigated (13). The study compared the genomic features of r/r LBCL patients with a separate group of treatment-naïve LBCL, in order to identify baseline and emerging alterations associated with resistance to CAR19 T cell therapy. Throughout this analysis, various factors influencing treatment outcomes were identified, as well as reciprocal interactions between tumor cells, CAR-T cells, and the tumor microenvironment. Although the mutational profile of r/r and treatment-naive LBCL appeared similar overall, certain genes, such as TP53 and c-MYC, exhibited higher mutation rates in the r/r samples. Additionally, differences were noted in the cell of origin and LymphGen subtype distribution in treatment-naïve versus r/r DLBCL patients.
Mutations in several genes, including IRF8, PAX5, TMEM30A, and TP53, were significantly correlated to poorer EFS after CAR19 therapy (13). In the context of subsequent treatment failure, genetic alterations emerged in various genes, including PPM1D, CD19, PAX5, and TP53. Furthermore, the somatic copy number alteration (SCNA) analyses on patients with adverse outcomes after CAR-T cell infusion identified amplifications of the 9p24.1 gene locus, which encodes the immune checkpoint molecules CD274 (PD-L1) and PDCD1LG2 (PD-L2). The hyper-expression that results from the amplification of PD-L1 and PD-L2 in the tumor cells may confer immune escape abilities and explains, at least in part, the resistance to axi-cel (20).
Specific mutation profiles of the tumor were found to have distinct effects on the expansion of CAR-T cells (13). Some mutations, such as those affecting BCL2, BTG, and TNFRSF14, were associated with heightened CAR19 T cell expansion, indicating a potential positive influence on the treatment efficacy. Conversely, the presence of the IRF4 mutation was linked to lower CAR-T cell expansion, suggesting that this mutation might hinder the effectiveness of axi-cel therapy. Of particular interest, the mutations observed in TNFRSF14, a member of the tumor necrosis factor receptor superfamily, were also linked to alterations in the immune cell composition within the tumor microenvironment, namely increased levels of T follicular helper cells and resting memory CD4 T cells. Previous evidence has documented that these specific mutations could promote an immunosuppressive environment, which might potentially impact the overall success of CAR-T cell therapy (21). In this respect, elevated levels of tumor-infiltrating CAR-T cells at the time of disease progression displayed gene signatures linked to those characteristics of increased exhaustion and higher inflammatory status of T cells.
To determine the impact of the STEP biomarkers on the EFS and OS of r/r LBCL, univariate Cox proportional hazards regression models were applied to the discovery cohort (13). Higher levels of ctDNA before treatment and at week 1 and week 4 after treatment were significantly associated with lower EFS and OS, while higher levels of cfCAR19 at week 1 were correlated to improved EFS. Subsequently, a multivariable model that included ctDNA levels at week 4 and cfCAR19 levels at week 1 was built, leading to the development of the STEP score (Figure 1). The STEP score has been shown to be prognostic for the investigated outcomes in both cohorts, with particularly poor EFS [hazard ratio (HR) =2.7, P<0.001] and OS (HR =5.4, P<0.001) for patients with high STEP score in the validation cohort.
In conclusion, the main results of this study pave the way to a deep understanding of the major molecular factors that are responsible for the resistance to CAR19 T cell therapy in r/r LBCL. In particular, the STEP score developed by Sworder et al. is meant to be a clinical tool to assess the outcome of patients treated with axi-cel (13). An additional encouraging finding is the positive correlation between the plasma levels of short cfCAR19 fragments and the infiltration of the tumor by CAR19 T cells. Notably, the measurement of cfCAR19 molecules would allow, for the first time, to assess the presence of CAR-T cells within tumors using a non-invasive method.
Since the study included a relatively limited number of patients and the majority of them were affected by one single type of LBCL, i.e., DLBCL, these initial promising findings need to be confirmed and expanded in a larger cohort of r/r LBCL patients with a broader inclusion of different subtypes of LBCL. Additionally, in order to highlight further possible molecular correlations between cfDNA and resistance to CAR19 T cells, the STEP analysis should be extended also to r/r LBCL patients treated with CAR-T cell products other than axi-cel, including both other CAR19 T-cells and CAR-T cells targeting other lymphoma antigens that are under scrutiny.
Despite these limitations, however, the study by Sworder et al. represents a revolutionary milestone in the understanding and managing of resistance to the treatment with CAR-T cells in LBCL (13). In the future, the application of STEP in lymphoma therapy with CAR-T cells might lead to optimizing the treatment strategy in view of a precision medicine approach exploiting a combination of biomarkers predictive of response and aiming at offering the best treatment option for a given patient. In addition, the STEP platform designed for B cell lymphoma also provides an important proof of concept that, in the future, might be adapted to the analysis of resistance of other tumor types to CAR-T cells directed toward antigens other than CD19.
Acknowledgments
Funding: Work by the authors described in this review has been supported by
Footnote
Provenance and Peer Review: This article was commissioned by the editorial office, Translational Cancer Research. The article has undergone external peer review.
Peer Review File: Available at https://tcr.amegroups.com/article/view/10.21037/tcr-23-1396/prf
Conflicts of Interest: All authors have completed the ICMJE uniform disclosure form (available at https://tcr.amegroups.com/article/view/10.21037/tcr-23-1396/coif). G.G. declares advisory board and speaker’s bureau honoraria from AbbVie, AstraZeneca, BeiGene, Incyte, Janssen, and Roche. The other authors have no conflicts of interest to declare.
Ethical Statement: The authors are accountable for all aspects of the work in ensuring that questions related to the accuracy or integrity of any part of the work are appropriately investigated and resolved.
Open Access Statement: This is an Open Access article distributed in accordance with the Creative Commons Attribution-NonCommercial-NoDerivs 4.0 International License (CC BY-NC-ND 4.0), which permits the non-commercial replication and distribution of the article with the strict proviso that no changes or edits are made and the original work is properly cited (including links to both the formal publication through the relevant DOI and the license). See: https://creativecommons.org/licenses/by-nc-nd/4.0/.
References
- Kurz KS, Ott M, Kalmbach S, et al. Large B-Cell Lymphomas in the 5th Edition of the WHO-Classification of Haematolymphoid Neoplasms-Updated Classification and New Concepts. Cancers (Basel) 2023;15:2285.
- Perales MA, Anderson LD Jr, Jain T, et al. Role of CD19 Chimeric Antigen Receptor T Cells in Second-Line Large B Cell Lymphoma: Lessons from Phase 3 Trials. An Expert Panel Opinion from the American Society for Transplantation and Cellular Therapy. Transplant Cell Ther 2022;28:546-59. [Crossref] [PubMed]
- Sermer D, Brentjens R. CAR T-cell therapy: Full speed ahead. Hematol Oncol 2019;37:95-100. [Crossref] [PubMed]
- De Marco RC, Monzo HJ, Ojala PM. CAR T Cell Therapy: A Versatile Living Drug. Int J Mol Sci 2023;24:6300. [Crossref] [PubMed]
- Roberts ZJ, Better M, Bot A, et al. Axicabtagene ciloleucel, a first-in-class CAR T cell therapy for aggressive NHL. Leuk Lymphoma 2018;59:1785-96. [Crossref] [PubMed]
- U.S. Food & Drug Administration. YESCARTA (axicabtagene ciloleucel) [cited 2023 Aug 02]. Available online: https://www.fda.gov/vaccines-blood-biologics/cellular-gene-therapy-products/yescarta-axicabtagene-ciloleucel
- U.S. Food & Drug Administration. KYMRIAH (tisagenlecleucel) [cited 2023 Aug 02]. Available online: https://www.fda.gov/vaccines-blood-biologics/cellular-gene-therapy-products/kymriah-tisagenlecleucel
- Neelapu SS, Locke FL, Bartlett NL, et al. Axicabtagene Ciloleucel CAR T-Cell Therapy in Refractory Large B-Cell Lymphoma. N Engl J Med 2017;377:2531-44. [Crossref] [PubMed]
- Schuster SJ, Bishop MR, Tam CS, et al. Tisagenlecleucel in Adult Relapsed or Refractory Diffuse Large B-Cell Lymphoma. N Engl J Med 2019;380:45-56. [Crossref] [PubMed]
- Abramson JS, Palomba ML, Gordon LI, et al. Lisocabtagene maraleucel for patients with relapsed or refractory large B-cell lymphomas (TRANSCEND NHL 001): a multicentre seamless design study. Lancet 2020;396:839-52. [Crossref] [PubMed]
- Locke FL, Ghobadi A, Jacobson CA, et al. Long-term safety and activity of axicabtagene ciloleucel in refractory large B-cell lymphoma (ZUMA-1): a single-arm, multicentre, phase 1-2 trial. Lancet Oncol 2019;20:31-42. [Crossref] [PubMed]
- Talleur AC, Gottschalk S. A tour de force provides novel insights into CD19-CAR T cell resistance mechanisms. Cell Rep Med 2023;4:100949. [Crossref] [PubMed]
- Sworder BJ, Kurtz DM, Alig SK, et al. Determinants of resistance to engineered T cell therapies targeting CD19 in large B cell lymphomas. Cancer Cell 2023;41:210-225.e5. [Crossref] [PubMed]
- Talotta D, Almasri M, Cosentino C, et al. Liquid biopsy in hematological malignancies: current and future applications. Front Oncol 2023;13:1164517. [Crossref] [PubMed]
- Rossi D, Diop F, Spaccarotella E, et al. Diffuse large B-cell lymphoma genotyping on the liquid biopsy. Blood 2017;129:1947-57. [Crossref] [PubMed]
- Scherer F, Kurtz DM, Newman AM, et al. Distinct biological subtypes and patterns of genome evolution in lymphoma revealed by circulating tumor DNA. Sci Transl Med 2016;8:364ra155. [Crossref] [PubMed]
- Kurtz DM, Scherer F, Jin MC, et al. Circulating Tumor DNA Measurements As Early Outcome Predictors in Diffuse Large B-Cell Lymphoma. J Clin Oncol 2018;36:2845-53. [Crossref] [PubMed]
- Kurtz DM, Esfahani MS, Scherer F, et al. Dynamic Risk Profiling Using Serial Tumor Biomarkers for Personalized Outcome Prediction. Cell 2019;178:699-713.e19. [Crossref] [PubMed]
- Frank MJ, Hossain NM, Bukhari A, et al. Monitoring of Circulating Tumor DNA Improves Early Relapse Detection After Axicabtagene Ciloleucel Infusion in Large B-Cell Lymphoma: Results of a Prospective Multi-Institutional Trial. J Clin Oncol 2021;39:3034-43. [Crossref] [PubMed]
- Cherkassky L, Morello A, Villena-Vargas J, et al. Human CAR T cells with cell-intrinsic PD-1 checkpoint blockade resist tumor-mediated inhibition. J Clin Invest 2016;126:3130-44. [Crossref] [PubMed]
- Boice M, Salloum D, Mourcin F, et al. Loss of the HVEM Tumor Suppressor in Lymphoma and Restoration by Modified CAR-T Cells. Cell 2016;167:405-418.e13. [Crossref] [PubMed]