Therapeutic strategies for HER2-positive breast cancer with central nervous system involvement: a literature review and future perspectives
Introduction
Breast cancer (BC) is the most frequent cancer worldwide among women (1). Approximately 15–20% of patients have overexpression/amplification of the human epidermal growth factor receptor 2 (HER2), identifying a more aggressive and historically prognostically poorer BC subtype (2). BC is one of the tumors with the higher incidence of brain metastases (BMs), with a rate of 10–16%, second only to lung cancer (3). Patients with HER2-positive (HER2+) metastatic breast cancer (MBC) develop BM in up to 55% of cases (4). The median time to onset of BM in HER2+ patients has been estimated to be 28–36 vs. 47–54 months in luminal subtypes (5). In the setting of MBC patients, those with BM often have extracranial metastases; the presence and the number of extracranial metastases represent a risk factor for the onset of BM (3). So far, most anticancer drugs for the treatment of BC have demonstrated to have limited intracranial activity, especially due to the presence of the blood-brain barrier (BBB), leading to the definition of the brain as a “sanctuary site” (6). Most deaths due to BC (about 90%) result from the presence of metastases rather than from the primary tumor, therefore the treatment of BM is still a clinical challenge (7). Despite all the advances in the understanding of BC biology, the key mechanism of development of BM in BC patients has not been completely elucidated yet (8). Indeed, the presence of the BBB restricts the access of cancer cells to the brain. For this reason, the cells must determine the modification of the brain extracellular matrix (ECM) to facilitate their passage and creating metastatic foci (9). As with other metastatic sites, the development of BMs from BC is a multi-step process with a cascade mechanism (10). We present this article in accordance with the Narrative Review reporting checklist (available at https://tcr.amegroups.com/article/view/10.21037/tcr-23-1126/rc).
Methods
In this review we describe the mechanism of onset of brain and leptomeningeal metastases in patients with HER2+ BC. We discuss updated data from clinical trials with different drugs conducted in this setting. Finally, we provide an overview on ongoing studies and potential strategies to improve patients’ clinical outcome. For this review, we performed an updated literature search on the role of HER2 in brain MBC and its treatment on main medical research databases and on international cancer meetings websites. For clinical trials, we collected and reviewed data of both completed and ongoing studies (see Table 1 for the search strategy summary).
Table 1
Items | Specification |
---|---|
Date of search | Up to May 2023 |
Databases and other sources searched | PubMed, Scopus and Web of Science; abstracts from ESMO, ASCO |
Search term used | “HER2-positive breast cancer”, “HER2 and brain metastasis”, “advanced breast cancer”, “integrated treatment”, “radiotherapy”, “HER2 target therapy” |
Timeframe | 1993–2023 |
Inclusion and exclusion criteria | Only articles in English were considered |
Selection process | Selection and collection of data was conducted independently by Passalacqua MI, Ciappina G, Di Pietro M, Squeri A, Granata B, Muscolino P and Spagnolo CC, data were analyzed and interpreted by Santarpia M |
ESMO, European Society for Medical Oncology; ASCO, American Society of Clinical Oncology.
Mechanisms of brain and leptomeningeal metastases development
The process of BMs development involves a series of steps through which the BC cells acquire a more aggressive phenotype and metastasize to different organs. The first step is a change in cell morphology and adhesion through the epithelial-mesenchymal transition (EMT). BC cells acquire typical mesenchymal characteristics allowing invasion, intravasation and distant metastasis (11). Preclinical studies investigated several pathways involved in the metastatic behavior of cancer cells and, in particular, the role of the X-inactive-specific transcript (XIST), that was demonstrated significantly downregulated in metastatic brain tissue. XIST is a long non-coding RNA (lncRNA) gene which plays a role in silencing X chromosome-linked genes. In particular, XIST-downregulation in BC seems to be responsible for the activation of the tyrosine kinase receptor c-MET, that facilitates the EMT (12). In the The Cancer Genome Atlas (TCGA) database, about 28% of HER2+ BCs have a decreased expression of XIST (13). Based on this evidence, it appears that XIST-expression suppresses the EMT-phenotype and therefore reduces metastasis (14).
The BBB is an anatomical-functional structure made up of three elements: endothelial cells (ECs), pericytes and astrocytes. Under physiological conditions, the BBB has the function of protecting the brain tissue from potentially harmful agents present in the blood, while allowing the passage of the substances necessary for the metabolic functions of the brain (15). Preclinical and clinical studies provided a precise characterization of the anatomical structure of the BBB, made up by the capillaries of the neuroparenchyma, where ECs with a series of physical, transport, and metabolic properties that regulating central nervous system (CNS) homeostasis are connected to each other by tight junctions (TJ) and surrounded by a basal lamina shared with the pericytes and with the astrocyte endfeet (16). The endothelium of the BBB and the cells of the brain parenchyma constitute the neurovascular unit (NVU) which acts as a “gatekeeper” that accurately selects the crossing of molecules and cells within the CNS (17). Regarding its functional role, the BBB is surrounded by the basal lamina, an ECM enriched in glycoproteins that can be cleaved by specific proteases to regulate the function of the BBB, both in physiological and pathological conditions. All three components of the BBB (ECs, pericytes and astrocytes) contribute to the formation of the basal lamina, which in turn provides for the release of ligands that can activate multiple pathways in the NVU (18,19). The BBB can be damaged as a result of the development of new, aberrant blood arteries during tumor progression, and this is known as the blood-tumor barrier (BTB). BTB is characterized by an aberrant distribution of pericytes and loss of astrocyte endfeet connections (20). Moreover, ECs lose TJ in BTB and consequently the intratumoral vascularization does not allow to re-establish the normal structure of the BBB in the presence of BMs. However, the BTB seems to maintain some of the fundamental functions of the BBB, including the presence of intracellular transporters both at the level of ECs and tumor cells (21). Furthermore, it appears that BTB takes on different characteristics depending on the type of supporting tumor. In particular, BMs from HER2+ breast tumors overexpress GLUT1 and BRCP transporters if compared to other subtypes (22). Drugs are distributed unevenly in BMs in mice models because the BTB is more porous and heterogeneous than the BBB, including in its permeability (23). In preclinical models, systemic injection in mice of low molecular weight molecules showed a higher distribution in BMs (24). The lipophilic, low molecular weight molecule lapatinib has been shown in preclinical studies to have an increased level distribution in BC BMs if compared to the surrounding healthy brain parenchyma (25). In the case of cancer cells, after extravasation into the blood circulation, these must penetrate through the BBB to determine BMs. Preclinical models have demonstrated the expression of membrane molecules on neoplastic cells, which allow them to breach the BBB and colonize the brain parenchyma through facilitated transcellular transport (26). For example, the expression of the 2,6-sialyltransferase molecule ST6GALNAC5 in circulating BC cells has been demonstrated (27). At least two other factors have been identified as facilitators of BBB passage by cancer cells: cyclooxygenase 2 (COX2) and heparin-binding epidermal growth factor (EGF)-like growth factor (HBEGF). COX2 is a prostaglandin capable of increasing the permeability of the BBB in inflammatory conditions. Preclinical studies have shown that the reduction of COX2 values resulted in a lower tendency to BM (28). HBEGF is a ligand of the epidermal growth factor receptor (EGFR) that promotes tumor cell motility and invasiveness (29). High levels of both COX2 and HBEGF could be responsible for the extravasation of BC cells into the brain (14). Another potential factor able to facilitate the formation of BMs, particularly in patients with HER2+ BC, is β-integrin. In fact, it seems that β-integrin interacts with HER2, causing a greater production of vascular endothelial growth factor (VEGF), which in turn is responsible for the alteration of the vascular endothelium, in particular of the loss of tight junctions, thereby favoring the increasing of the permeability of the BBB and favoring the formation of the BM (30). Once the BBB is crossed, tumor cells must interact with the local microenvironment, in particular with microglia cells, i.e., the innate brain immunity. When the microglia is activated, it triggers the release of inflammatory factors and cytokines aimed at fighting the potential pathogen. However, microglia can act both as an inhibitor of tumor development (M1 microglia) and as a tumor promoter (M2 microglia). Tumor cells escape the protective function of microglia through two mechanisms: the first is the inhibition of cytokine release by M1, and the second is facilitating a switch from the M1 to M2 phenotype (31).
Cancer stem-like cells (CSCs) play a fundamental role in the development of BMs. These represent a small fraction of primary tumor cells that exhibit characteristics typical of stem cells and have a high replication potential (32). These cells are also endowed with high motility and invasiveness and have also been found in HER2+ BC with BM (33).
Several pathways have been identified as responsible for the activity of CSCs, but one in particular seems to have a key role in the formation of BMs from HER2+ BC, that is the truncated glioma-associated oncogene homolog 1 (TGLI1). TGLI1 is a transcription factor involved in angiogenesis, cell migration, and invasion. Preclinical studies have demonstrated that high levels of TGLI1 appear to be related to a higher incidence of BM and a lower BM-free survival. Furthermore, CSCs deriving from radioresistant BMBCs show elevated levels of TGLI1 (34).
Also, the alterations of the TP53 gene seem to be implicated in the pathogenesis of BMs from HER2+ BC. The TP53 gene encodes the p53 tumor suppressor which has an important regulatory function of the cell cycle, apoptosis and DNA repair mechanisms (35).
In a study by Koboldt et al., alterations of TP53 gene have been identified in approximately 72% HER2+ primary tumors (compared with 12% of luminal A and 29% of luminal B tumors) (36), suggesting that it has a key role in these tumors, although the molecular mechanism that directly correlates the TP53 mutations with the onset of BM is not yet well known (14).
CNS involvement in patients with HER2+ BC could also be represented by leptomeningeal metastases in the absence of brain parenchymal involvement, although this is a rarer event (37). Regarding the leptomeningeal localization, the pathogenetic mechanisms are not yet clear. The meninges are represented by the dura mater, arachnoid and pia mater. Pia mater and arachnoid constitute the leptomeninges. The space below the arachnoid, known as the subarachnoid space, is filled with blood vessels, cerebrospinal fluid (CSF), and connective tissue rich in trabeculae, functioning as a gateway for cancer cells (38). On the basis of preclinical studies, at least three different mechanisms have been proposed for the pathogenesis of leptomeningeal disease: (I) direct invasion through surrounding structures such as the dura mater, bone or nerves, (II) diffusion by hematogenous way, and finally (III) diffusion through the pores present on the choroidal plexus (39). From a molecular point of view, it seems that in the CSF of patients with leptomeningeal metastases there is an increase in the expression of the C3 fraction of complement proteins. This C3 protein would be able to interact with C3a receptors present at the level of the choroid plexus, causing an increase in permeability even in the presence of an intact BBB (40).
In conclusion, a better knowledge of the molecular and pathogenetic mechanisms underlying the formation of brain and leptomeningeal metastases in patients with HER2+ BC is necessary to address specific therapeutic strategies to improve objective responses and long-term clinical benefit (Figure 1).
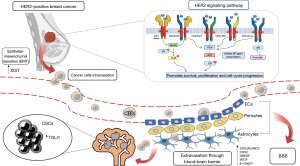
Therapeutic strategies for BC with BM
The prevalence of HER2+ BC patients with BMs has increased in the last years as a consequence of the improved survival rates in this subgroup of BC and thanks to the increased detection of metastatic brain localization through advanced imaging techniques. The prognosis of patients with BMs is generally poor, but the optimization of care with integrated treatments and the development of new drugs are slowly improving the outcomes. In particular, better survival outcomes were demonstrated in patients with HER2+ BC BMs than HER2− BC (41). The definition of subgroups by well-recognized prognostic factors is essential to address the correct therapeutic strategy for each patient. In fact, independently of biological features of the tumor, the most important favorable prognostic factors in patients with BM, and in general in those with advanced stages of disease, are referable to a good Eastern Cooperative Oncology Group (ECOG) Performance Status, age <60 years old (42), the presence of single BM, the absence of extracranial disease, a good control of the systemic disease and a long time from initial diagnosis to metastatic disease (43,44).
Therapeutic strategies for BMs are currently based on both local treatments, such as surgery and radiotherapy, and on systemic treatments. The combination of different strategies, as a result of multidisciplinary management, can be selected on the basis of specific characteristics, including the number of BM, their localization, and the biologic features of primary tumor (45).
Local treatments of BM
Local therapeutic strategies for BM have been largely evaluated in all subgroups of BC patients, including HER2+ BC.
Neurosurgical resection of BM can be a therapeutic option in patients with few lesions or large symptomatic lesions (≥3 cm), when well-controlled systemic disease coexists. In patients with low performance status or in imminent life threatening, surgery can also be used to contrast the central mass effect and neurological related symptoms, thus obtaining a quick clinical benefit (46).
The combination of surgery and radiotherapy have also been explored. In a randomized, multicentric study including 48 patients, surgery followed by whole-brain radiotherapy (WBRT) was compared with WBRT alone. WBRT following complete surgical resection showed to decrease the recurrence rate both in the initial metastatic site (10% vs. 46%, P<0.001) and in other brain sites (14% vs. 37%, P<0.01) as well as death caused from intracranial progression (14% vs. 44%, P=0.003). However, overall survival (OS) was comparable in the two groups (47). Another multicentric randomized trial, including 64 patients, compared surgery followed by WBRT and radiotherapy (RT) alone. A prolonged OS was observed with surgery followed by radiotherapy compared to radiotherapy (10 vs. 6 months; P=0.04) (48).
WBRT after surgery is burdened by both short- and long-term toxicities regarding to the global health status, physical functioning, cognitive functioning, and fatigue. Generally, most of these effects are transitory, but negatively impact on the quality of life of treated patients (49).
Post-operative stereotactic radiosurgery (SRS) was compared with post-operative WBRT in two phase 3 randomized clinical trials. Data deriving from these studies led to the recommendation of SRS after surgery when feasible. In fact, although no differences in terms of OS were demonstrated between the two radiotherapy techniques, a reduced risk of cognitive impairment was achieved with SRS, and local control was slightly better with SRS in both studies (50,51).
Radiotherapy with stereotactic methods (e.g., gamma-knife, cyber-knife, volumetric modulated arc therapy (VMAT) or linear accelerator) consists in the administration of a single dose or hypo-fractionated RT with a high dose radiation on a limited volume target (52). To date, no clinical trials have been designed to establish whether stereotactic radiotherapy, in patients with a single BM, can replace surgery. There are no randomized trials directly comparing stereotactic RT to surgery, so they are considered a comparable treatment in terms of efficacy for patients with few or small brain lesions.
However, surgery is not always feasible, even in presence of few BMs or small lesions, so SRS alone can be considered the choice option. It was demonstrated that in patients with ≤10 BM, SRS alone give an excellent local control (around 90%), even if in the group of patients with 5–10 BM the risk of leptomeningeal dissemination was higher compared to patients with only 2–4 BM. However, two-years outcome in terms of neurological death, new brain-lesion incidence and neurocognitive abilities were similar (53). Another study demonstrated that SRS alone in patients with ≥10 BM achieved a median OS of only 4 months, although an initial local control was obtained (54). An exploratory analysis of a retrospective study evaluating the neurocognitive outcome in the same cohort of patients, demonstrates that, although a stereotactic treatment is feasible in patients with more than four BMs, the overall volume of the intracerebral disease is a factor that has a statistically significant impact on the treatment efficacy and must be less than 15 cm3 (55).
Patients with one to three BMs were enrolled in a clinical study and randomly assigned to receive WBRT or WBRT followed by stereotactic radiosurgery boost. It was demonstrated that WBRT and stereotactic boost therapy increased functional autonomy in all patients and survival in those with a single unresectable BM (56).
WBRT is recommended both for patients who are ineligible for surgery or stereotactic RT and as a consolidation therapy after SRS, in presence of high-volume BM or high number of BMs (57). The median overall survival after WBRT was detected around 3–6 months when administered as initial treatment to patients with multiple BMs, with a 10–15% of BM patients still alive at 1 year (58). The WBRT regimen generally involves the administration of a total radiant dose of 30–40 Gy in 10–15 daily fractions. It is not proven if higher doses can enhance survival or local control. Moreover, fractions per day greater than 3 Gy can increase the risk of neurotoxicity (59).
For patients with asymptomatic BMs, systemic therapies can be administered first to control systemic disease. The WBRT should be reserved to treat worsening cerebral and neurological symptoms. In selected cases, systemic therapy has a favorable prognosis on BMs and spares patients from long-term toxicities deriving from WBRT (44).
At last, best supportive care may be considered for patients with a very poor prognosis (60).
Systemic therapy
Systemic treatment can be considered the first choice in newly diagnosed patients with CNS localization by HER2+ BC if BMs are small, numerous, asymptomatic or barely symptomatic. Various treatment options and drug combinations have proven to be effective in the management of BM-positive BC (61).
Trastuzumab-pertuzumab
Results obtained from the phase 3 CLEOPATRA trial, led to the approval of pertuzumab plus trastuzumab plus a taxane as first-line treatment in patients with advanced HER2+ BC. This combination showed to be more effective than trastuzumab plus taxane in terms of both progression-free survival (PFS) and OS [HR for PFS 0.68, P=0.001; hazard ratio (HR) for OS: 0.68, P=0.001]. Patients with BMs at diagnosis were excluded from this study, nevertheless, BMs were the first recurrence in 13% of enrolled population (62). An exploratory analysis of the CLEOPATRA trial showed a longer median time to the development of CNS metastases as first site of disease progression in the pertuzumab arm (15.0 vs. 11.9 months, HR 0.58). These data support the efficacy of the combination of these monoclonal antibodies in advanced BC with BM, despite their low BBB permeability (63). The efficacy of the combination was evaluated in HER2+ BC patients with pretreated BMs in PATRICIA trial, exploring high dose of trastuzumab to enhance the effect at CNS level (Results are described below, in “New perspective section”) (64).
Antibody-drug conjugates (ADCs)
Based on the positive results from EMILIA trial, trastuzumab emtansine (T-DM1) was previously considered the gold standard for second-line therapy in HER2+ metastatic BC. In fact, T-DM1 showed a significant improvement in PFS and OS compared to lapatinib and capecitabine in this setting, with a median PFS as assessed by independent review of 9.6 months with T-DM1 vs. 6.4 months with lapatinib plus capecitabine [HR 0.65; 95% confidence interval (CI): 0.55 to 0.77; P<0.001], and median OS of 30.9 vs. 25.1 months (HR 0.68; 95% CI: 0.55 to 0.85; P<0.001) (65). In an exploratory, retrospective analysis of the EMILIA trial, among the 95 patients with asymptomatic BMs included in the trial, OS was better in patients receiving T-DM1 compared to those receiving lapatinib and capecitabine (median OS, 26.8 vs. 12.9 months) (66). The intracranial activity of T-DM1 in HER2+ BC patients was examined by Bartsch et al. T-DM1 was administered as a main systemic therapy for BM or upon documented CNS progression following initial local treatment. At 8.5 months of median follow-up, the median OS was not reached, while the intracranial PFS was 5 months (67). The efficacy and safety of T-DM1 in this clinical scenario were further confirmed by a subgroup analysis of 398 BM-positive patients who were enrolled in the phase 3 KAMILLA trial, a single-arm, open-label study. The BM response rate was 21%, and the median PFS and OS were 5.5 and 18.9 months, respectively (68). In the earlier setting, data from the KATHERINE trial showed a significant survival benefit with adjuvant T-DM1 when administered after failure to achieve pathological complete response (pCR) with neoadjuvant therapy, but the incidence of CNS recurrence as a first site of metastasis was numerically higher in T-DM1 group compared to trastuzumab (5.9% vs. 4.3%, respectively) (69).
Recently, new agents have been developed, including the ADCs and tyrosine kinase inhibitors (TKIs), some of which have already been approved for HER2+ BC, based on meaningful clinical benefit, also in patients with BMs. These drugs include the trastuzumab deruxtecan (T-DXd) (70) and tucatinib (71). Tucatinib activity will be described in TKIs section.
T-DXd is an ADC currently approved for the second-line treatment of advanced HER2+ BC due to the outstanding outcomes of DESTINY-Breast03 trial. DESTINY-Breast03 is a phase 3, multicentric, open-label, randomised trial that compared efficacy and safety of the T-DXd to T-DM1 in patients with HER2+ MBC who have previously received trastuzumab and a taxane (70). The last update, with a median duration of study follow-up of 28.4 months with T-DXd and 26.5 months with T-DM1, reported a median PFS by blinded independent central review of 28.8 months with T-DXd and 6.8 months with T-DM1 (HR 0.33; P<0.0001). Median OS was not reached, with 72 (28%) overall survival events reported in the T-DXd group and 97 (37%) overall survival events in the T-DM1 group (HR 0.64; P=0.0037). All prespecified subgroups, including patients with stable BM, experienced the same benefit. A manageable safety profile of T-DXd was confirmed with longer treatment duration. Grade 3 or worse adverse events were numerically similar in both groups, but the exposure adjusted incidence rates were lower in T-DXd group (grade 3 or worse: 0.36 in T-DXd vs. 0.65 in T-DM1 group). The most common drug-related adverse events were neutrophil and platelet count decreased and anemia in both arm and, in addition, transaminases increasing in T-DM1 group. Adverse events that led to discontinuations with T-DXd were pneumonitis (6% of patients), interstitial lung disease (5%), and pneumonia (2%), and with T-DM1 were platelet count decreased (2%), pneumonitis (1%), and thrombocytopenia (1%). Adjudicated drug-related interstitial lung disease or pneumonitis that occurred in the T-DXd group were 4% of grade 1, 10% of grade 2, <1% of grade 3 and no grade 4–5 adverse events, with a median time to first onset of 8.1 months [interquartile range (IQR): 4.2–15.0 months] (72).
Encouraging results have been obtained in the phase II clinical trial TUXEDO-1, where the activity of T-DXd was explored in HER2+ BC patients with active BMs. Patients with newly diagnosed, untreated BMs or BMs progressing after prior local treatment, prior therapy with trastuzumab and pertuzumab, and no indication for immediate local therapy, were enrolled in this trial. T-DXd was administered intravenously once every three weeks at a dose of 5.4 mg/kg. Intracranial response was the primary endpoint. Among fifteen patients enrolled, 2 (13.3%) had a complete intracranial response, nine (60%) had a partial intracranial response and three (20%) maintained stable disease, with a best overall intracranial response rate of 73.3%, thus meeting the predefined primary outcome. Moreover, at a median follow-up of 12 months, median OS was not reached and the median PFS was 14 months (73).
In the phase II DEBBRAH trial, T-DXd was assessed in patients with CNS involvement, showing an interesting intracranial activity in patients with stable, untreated, or progressing BMs, achieving all them partial responses, as well as generally manageable toxicity and maintained quality of life (74).
In conclusion, ADCs have shown significant activity on BM, despite their large molecular size, probably, as previously commented, as a result of a partially disrupted BBB in advanced disease with CNS localization.
Tyrosine-kinase inhibitors (TKIs)
TKIs are frequently used as later-line therapy options for HER2+ metastatic BC with CNS involvement.
Lapatinib, a first-generation TKI, is a dual HER1 and HER2 kinase inhibitor. Better outcomes for BM positive patients have been reported when lapatinib was combined with capecitabine, than when used as a monotherapy (75). The LANDSCAPE trial is a single arm phase 2, open-label, which enrolled HER2+ advanced BC patients with BM who had not previously received WBRT or systemic therapy including capecitabine or lapatinib. With a median follow up of 21.2 months, out of 45 enrolled patients, 29 had an objective CNS response (65.9%, 95% CI: 50.1–79.5%); all were partial responses. The median time to progression (TTP) and the median time to brain radiotherapy were 5.5 and 8.3 months, respectively (76). The intracranial response rates in this study may have been overestimated because half of the patients had asymptomatic BM. However, these findings suggest that patients with early-stage intracranial disease have better prognosis, raising the question of whether to introduce a routine screening technique in a high-risk group (3). In the CEREBEL trial, which involved 540 patients without BMs, the preventive effect of the combination capecitabine-lapatinib on the development of BMs was analyzed. These patients were randomly assigned to receive capecitabine plus lapatinib or trastuzumab. The incidence of CNS events was low and comparable between the capecitabine-lapatinib arm (3%) and the capecitabine-trastuzumab arm (5%). Therefore, the combination of capecitabine-lapatinib is not considered better to trastuzumab-based therapy to control intracranial disease or to prevent new CNS localization (77). Moreover, the combination of lapatinib and SRS (concurrent or sequential) demonstrated to significantly decrease local failure and to increased OS in patients with BM, with greater benefit in local control among patients with smaller lesions (78).
Neratinib is a novel and irreversible pan-HER TKI, that has been evaluated in combination with capecitabine in patients with HER2+ BC with BM. The activity of neratinib plus capecitabine was first evaluated in the phase II trial TBCRC 022 in this cohort of patients, both pretreated and untreated with lapatinib. The primary endpoint was the composite CNS objective response rate (ORR), that reached 49% in lapatinib-naïve cohort and 33% in lapatinib-treated cohort. Median PFS was 5.5 and 3.1 months, respectively, remarking the evidence that the efficacy of HER2-directed therapy in the brain is enhanced by chemotherapy (79). The NALA trial is a phase III study which compared neratinib plus capecitabine to lapatinib plus capecitabine in patients with metastatic HER2+ BC who have received two or more prior HER2-directed regimens. Patients with stable, asymptomatic CNS disease were included. Neratinib with capecitabine demonstrated to be superior in terms of PFS (HR 0.76) and showed a significant intracranial activity resulting in lower incidence of BM (22% vs. 29.2% in lapatinib-capecitabine) and less interventions for symptomatic CNS metastases. Diarrhea, nausea, palmar-plantar erythrodysesthesia syndrome, and vomiting were the most common adverse events in both arms. Grade 3 diarrhea occurred in 74 patients (24.4%) with neratinib, most prevalent during the first cycle, and 39 patients (12.5%) with lapatinib (80).
Tucatinib is an oral tyrosine kinase inhibitor that is highly selective for the kinase domain of HER2, with minimal inhibition of HER1. In the HER2CLIMB, a phase III trial, patients who had received both dual anti-HER2 blockade and T-DM1 as prior treatments, were enrolled to receive tucatinib or placebo in combination with capecitabine and trastuzumab. Tucatinib showed higher activity compared to placebo in PFS (7.8 vs. 5.6 months; HR 0.54, 95% CI: 0.42–0.71, P<0.001) and OS (21.9 vs. 17.4 months; HR 0.66, 95% CI: 0.50–0.87, P=0.0048) (71). Active BMs were not an exclusion criterion, in fact this trial was the first large randomized study to enroll patients with active BM. If immediate treatment was required, enrollment was allowed after the local treatment. Median PFS was 7.6 months (95% CI: 6.2–9.5) and 5.4 months (95% CI: 4.1–5.7) in the experimental and control arm, respectively, in patients with active BMs or previous evidence of CNS involvement (total =291; 198 in tucatinib group and 93 in control group). In these 291 patients, the addition of tucatinib improved intracranial PFS (9.9 vs. 4.2 months; HR 0.48, 95% CI: 0.34–0.69, P<0.00001) and OS (18.1 vs. 12 months; HR 0.58; 95% CI:0.40–0.85, P=0.005), with a 1-year intracranial PFS of 35% vs. 0% in the experimental group and in the control group, respectively. Moreover, as per protocol, 30 patients with isolated brain progression could continue treatment after a local intervention and the median time from randomization to second disease progression or death was 15.9 months with tucatinib vs. 9.7 months in the control group (HR 0.33; 95% CI: 0.11–0.02). Adverse events most frequently detected in the tucatinib-combination group were diarrhea, palmar-plantar erythrodysesthesia syndrome, nausea, fatigue, and vomiting, mostly of grade 1 or 2. The most common adverse events of grade 3 or higher observed in the tucatinib-combination group were palmar-plantar erythrodysesthesia syndrome, diarrhea, elevations in alanine aminotransferase (ALT) and aspartate aminotransferase (AST) levels, and fatigue. Adverse events led to the discontinuation of tucatinib in 5.7% of the patients and of placebo in 3.0% of the patients (81). In addition, the activity of the combination of tucatinib plus T-DM1 is under study in the ongoing clinical trials COMPASS-RD (NCT04457596) and HER2CLIMB-02 (NCT03975647), which are analyzing the high-risk post-neoadjuvant therapy and metastatic BC disease, respectively.
Pyrotinib is an oral, irreversible inhibitor of the TK activity of EGFR, HER2 and HER4 (82). The combination of pyrotinib and capecitabine has been evaluated in the subgroup of 31 patients with BMs in the PHENIX phase III trial, showing a significant benefit on PFS compared to capecitabine plus placebo (6.9 vs. 4.2 months) (83). The activity and the safety of pyrotinib plus capecitabine has been investigated also in the phase II PERMEATE trial, both in patients who had radiotherapy-naive HER2+ BM (cohort A) and progressive disease after radiotherapy (cohort B). At a median follow up of 15.7 months, the intracranial ORR (primary endpoint) was 74.6% (44 of 59 patients) in cohort A and 42.1% (8 of 19 patients) in cohort B. The most common grade 3 adverse effects (AE) was diarrhea in both cohorts and no treatment-related deaths occurred (84).
Poziotinib is an oral pan-HER kinase inhibitor that demonstrated a potent activity through irreversible inhibition of HER1, HER2 and HER4 (85). The phase II NOV120101-203 trial evaluated the efficacy and safety of poziotinib monotherapy in patients with HER2+ metastatic BC who had progressed from more than two HER2-directed therapies, including patients with BM (86). Long term analysis showed clinically meaningful OS benefit for patients with HER2+ metastatic BC (87), but further studies addressed to HER2+ BC with BM are necessary (Table 2).
Table 2
Category | Drug | Trial | Phase | Trial design | N. of pts (to date) | OS (months) | mPFS (months) |
---|---|---|---|---|---|---|---|
Anti-HER2 monoclonal antibody | Pertuzumab | CLEOPATRA (NCT00567190) | III | Exp arm: trastuzumab + pertuzumab + taxane | 808 | Exp arm: 56.5 | Exp arm: 18.7 |
Control arm: trastuzumab + placebo + taxane | Control arm:40.8 | Control arm: 12.4 | |||||
Antibody-drug conjugates (ADCs) | Ado-T-DM1 | EMILIA (NCT00829166) | III | Exp arm: ado-T-DM1 | 991 | Exp arm: 30.9 | Exp arm: 9.6 |
Control arm: lapatinib+capecitabine | Control arm: 25.1 | Control arm: 6.4 | |||||
KAMILLA (NCT01702571) (post hoc analysis in pts with BM) | IIIb | Ado-T-DM1 (single arm) | 398 | 18.9 | 5.5 | ||
Trastuzumab deruxtecan | DESTINY-BREAST03 (NCT03529110) | III | Exp arm: trastuzumab deruxtecan | 524 | Exp arm: NR | Exp arm: 28.8 | |
Control arm: T-DM1 | Control arm: NR | Control arm: 6.8 | |||||
TUXEDO-1 (NCT04752059) | II | Trastuzumab deruxtecan (single arm) in pts with newly diagnosed untreated brain metastases or brain metastases progressing after previous local therapy, previous exposure to trastuzumab and pertuzumab and no indication for immediate local therapy* | 15 | NR | 14 (95% CI: 11.0%–NE) | ||
DEBBRAH (NCT04420598) | II | Trastuzumab deruxtecan in: | 21** | NE | 6-month PFS rate: 78.7% (95% CI: 52.7–91.5%); 9-month PFS rate: 61.4% (95% CI: 31.0–81.6%) in the first 3 cohorts | ||
Cohort 1: HER2-positive BC with non-progressing BM (after WBRT and/or SRS and or surgery) | |||||||
Cohort 2: HER2-positive or HER2-low BC with asymptomatic untreated BM | |||||||
Cohort 3: HER2-positive BC with progressing BMs after local treatment | |||||||
Cohort 4: HER2-low expressing BC with progressing BMs after local treatment | |||||||
Cohort 5: HER2-positive or HER2-low expressing BC with LMC | |||||||
Tyrosine-kinase inhibitors (TKIs) | Lapatinib | LANDSCAPE (NCT00967031) | II | Lapatinib + capecitabine in pts with HER-2 positive BC with brain metastases not previously treated with WBRT (single arm) | 44 | 17.0 | 6.2 |
CEREBEL (NCT00820222)ꝉ | III | Exp arm: lapatinib + capecitabine | 540 | Exp arm: 22.7 | Exp arm: 6.6 | ||
Control arm: trastuzumab + capecitabine | Control arm: 27.3 | Control arm: 8.1 | |||||
Neratinib | NALA (NCT01808573) | III | Exp arm: neratinib + capecitabine | 621 | Exp arm: 24.0 | Exp arm: 5.6 | |
Control arm: lapatinib + capecitabine | Control arm: 22.2 | Control arm: 5.5 | |||||
Tucatinib | HER2CLIMB (NCT02614794) | III | Exp arm: tucatinib + trastuzumab + capecitabine | 480 | Exp arm: 21.9 | Exp arm: 7.8 | |
Control arm: placebo + trastuzumab + capecitabine | Control arm: 17.4 | Control arm: 5.6 | |||||
Pyrotinib | PHENIX (NCT02973737) | III | Exp arm: pyrotinib + capecitabine | 279 | Exp arm: NR | Exp arm: 11.1 | |
Control arm: placebo + capecitabine | Control arm: NR | Control arm: 4.1 | |||||
PERMEATE (NCT03691051) | II | Pyrotinib + capecitabine in pts with BM from HER-2 positive BC (pretreated with Trastuzumab) in: | 78 | ||||
Cohort A: pts with BM who have not previously been treated with radiation therapy | Cohort A: 35.9 (95% CI: 25.1–NR) 1-year OS rate: 84.6% (95% CI: 76.0–94.4%); 3-year OS rate: 49.8% (95% CI: 38.0–64.6%) | Cohort A: 10.7 (95% CI: 7.6–14.9) | |||||
Cohort B: pts with progression disease or new lesions after WBRT or SRS | Cohort B: 31.4 (95% CI: 22.0–NR) 1-year OS rate: 78.9% (95% CI: 64.0–99.6%); 3-year OS rate: 29.1% (95% CI: 13.0–60.4%) | Cohort B: 5.6 (95% CI: 5.4–11.9) |
*, The primary endpoint of the study was intracranial response rate measured according to the response assessment in neuro-oncology brain metastases criteria. In the per protocol population (PP; n=14 patients), the response rate was 78.6% (95% CI: 49.2–95.3%) (11/14). **, Cohort 1: n=8; cohort 2: n=4; cohort 3: n=9. Patients recruitment in cohorts 4 and 5 was ongoing at the time of data cutoff (October 20, 2021). ꝉ, Primary endpoint of the study was incidence of CNS metastases as first site of relapse. Incidence of CNS metastases as first site of relapse was 3% for lapatinib-capecitabine and 5% for trastuzumab-capecitabine (treatment differences, 21.6%; 95% CI: 22% to 5%; P=0.360). MBC, metastatic breast cancer; pts, patients; OS, overall survival; mPFS, median progression-free survival; exp, experimental; T-DM1, trastuzumab emtansine; BM, brain metastasis; BC, breast cancer; NR, not reached; NE, not estimable; LMC, leptomeningeal carcinomatosis; WBRT, whole-brain radiotherapy; SRS, stereotactic radiosurgery.
New perspectives in treatment of BMs
In patients with BMs and leptomeningeal disease, the intrathecal administration of drugs is an emergent treatment strategy under investigation.
The potential activity of intrathecal trastuzumab, compared to intrathecal administration of chemotherapy or to WBRT has been underlined in some case reports and retrospective analysis (88). Intrathecal administration of trastuzumab in patients with leptomeningeal metastases have been investigated in phase I/II trial, using different schedule of treatment and dose. The Phase I trial NCT01373710 has evaluated weekly intrathecal trastuzumab and established the maximum tolerated dose at 150 mg. The Phase II part of the same trial is ongoing. Another phase I/II trial (NCT01325207) established the maximum tolerated dose at 80 mg, administered for the first month two times in a week and after, weekly until progression. The primary endpoint of the second part (phase II) was the attainment of a 25% of response rate, but it has not been reached. Clinical benefit has been observed in 69% of patients (89). The second part of another phase I/II trial with intrathecal administration of trastuzumab at 150 mg is ongoing (90).
Leptomeningeal disease in patients with HER2+ BC is still an open challenge. Despite the recent, significant advances in the treatment of BM in HER2+ BC, thanks to DestinyBreast03 (72) and HER2CLIMB (81), more data is needed about the role of T-DXd and Tucatinib in this setting, due to the exclusion of these patients from the trials. A recent retrospective study reported the activity of T-DXd in patients with HER2+ metastatic BC and leptomeningeal metastases (LM) progression (91), based on the revised magnetic resonance imaging (MRI)-scorecard for response assessment in LM by the European Organization for Research and Treatment of Cancer (EORTC) Brain Tumor Group and Response Assessment in Neuro-Oncology (RANO) (92). Across 8 patients enrolled, seven (87.5%) had prior WBRT and all were very heavily pretreated with a median of 4.5 prior therapies. Median time on T-DXd was 7.6 months with a median of 10 cycles. Clinical benefit response was 100%, with 4 patients that achieved a partial response on MRI as best response, while 4 patients had stable disease. No patient reported BM progression while on T-DXd and the median OS was 10.4 months since the start of T-DXd (91). To validate these data, prospective clinical trials are necessary.
In the phase II PATRICIA study, including patients with metastatic HER2 + BC that underwent CNS progression after radiant treatment, another unconventional strategy was evaluated: the systemic use of high dose of trastuzumab (6 mg/kg weekly) in combination with pertuzumab. The ORR was 11% and the clinical benefit rate (CBR) [complete response + partial response + stable disease (SD) of ≥4 or ≥6 months] was 68% (SD ≥4 months) and 51% (SD ≥6 months), without new safety signals (64).
Across new therapeutic approaches to increase activity of anticancer agents in CNS, the possibility to temporarily disrupt BBB to deliver higher concentrations of anti-HER2 drugs into the brain has been evaluated. The first strategy to obtain an increasing permeability was an osmotic approach by using mannitol to open the cerebral blood vessels, however this strategy did not provide evidence of efficacy (NCT00397501). A more promising strategy in BBB disruption (BBBD) is under study on patients with BC HER2+ BM (NCT03714243) and consists of combine the use of focused ultrasound (FUS), that enhances drug transport by a microbubble-assisted mechanism, and the BBBD MRI-guided, that has the advantage of being localized and temporaneous. The systemic administration of trastuzumab, in combination with this strategy, has already demonstrated both antitumor effect in mice with BMs and early safety results in humans (93,94).
The use of nanoparticles conjugated with anticancer agents, such as molecular inhibitors of EGFR or HER2, has been explored in preclinical model, demonstrating significantly prolonged survival in mice with HER2+ BC BM (95). It was also observed a strongly inhibition of tumor progression during the first moments of metastasis development, hypothesizing a possible preventive role in development of BM (96). Recent developments led to study the use of lipid nanoparticulate formulations, characterized by major surface to mass ratio if compared to other colloidal particles and by the capability of adsorbing and/or binding other molecules, such as cancer and immunotherapeutic agents (97). These compounds have been explored in preclinical models with HER2+ BC, with several lipid nanocarrier candidates for immunotherapy. Among these, for example, are numbered liposomes carrying LAG3-Ig + P5 HER2/neuderived peptide (98) or trastuzumab (99), immunoliposomes carrying anti-Her2/neu monoclonal antibody (100), virosomes carrying Fab’ fragments of an antirat Neu (anti-rNeu) monoclonal antibody (101) and exosomes carrying anti-human CD3 and anti-human HER2 antibodies (102). Further studies in human models are needed for their clinical validation.
Ongoing trials
A genomic-guided phase 2 trial (Alliance A071701, NCT03994796) is actually recruiting patients with progressive parenchymal CNS disease, after biopsy of intracranial and extracranial lesions, to individuate specific targetable pathways (neurotrophic tropomyosin receptor kinase (NTRK), ROS1 fusions, CDK and PI3K pathways). If present, treatment known to be CNS-permeable (such as Entrectinib for NTRK and ROS1, Abemaciclib for CDKs, or GDC-0084 for PI3K) will be allowed.
Novel ADCs are under investigation in phase 2 trials enrolling patients with HER2+ BM, such as trastuzumab rezetecan (SHR-A1811) single agent or in combination with pyrotinib or bevacizumab (NCT05769010) and ARX788 single agents (NCT05018702).
Various combinations of different agents are under study. The combination of palbociclib, trastuzumab, and lapatinib with fulvestrant in ER+/HER2+ BC BM is being evaluated in a single-arm trial in China (NCT04334330). Another unique combination of tucatinib, abemaciclib, and trastuzumab is being evaluated for patients with “triple positive” MBC, with specific CNS secondary endpoints (NCT03846583).
Early-phase clinical trials are evaluating the role of afatinib, a TKI that disrupts HER2 and EGFR kinases, in BMs from various solid tumors (NCT02423525, NCT02768337). An ongoing phase I/II study compare the effects of combination of afatinib and T-DM1 against T-DM1 alone in individuals with HER2+ BC and CNS dissemination (HER2BAT, NCT04158947).
Phase 1 clinical trials are testing the interaction of WBRT with the anti-angiogenic TKI sorafenib and the ATM inhibitor AZD1390 (NCT01724606 and NCT03423628).
Finally, a single-arm Phase 2 trial for HER2+ BM is testing the safety and effectiveness of combining the PI3K inhibitor GDC-0084 with trastuzumab (NCT03765983). More ongoing trials are reported in Table 3.
Table 3
Trial | Trial design | Phase | No. of pts (to date) | Primary endpoint(s) | Secondary endpoint(s) |
---|---|---|---|---|---|
Alliance A071701 (NCT03994796) | Exp arm I (CDK gene mutation): abemaciclib PO BID on days 1–28 until disease progression or unacceptable toxicity | II | 150 | ORR in the brain | Systemic response for extracranial disease, clinical benefit rate for CNS, DOR for BM, DOR for extracranial disease, PFS-intracranial, PFS-extracranial, OS, safety |
Exp arm II (PI3K gene mutation): PI3K inhibitor paxalisib PO QD on days 1–28 until disease progression or unacceptable toxicity | |||||
Exp arm III (NTRK/ROS1 gene mutation): entrectinib PO QD on days 1–28 until disease progression or unacceptable toxicity | |||||
NCT04334330 | Palbociclib, trastuzumab, pyrotinib and fulvestrant | II | 34 | ORR in CNS | Safety, OS, PFS, ORR, time to CNS progression, time to radiotherapy |
NCT04582968 | Pyrotinib + capecitabine + SRT/WBRT | I/II | 39 | Safety, ORR intracranial | ORR intracranial rate with SRT/WBRT; PFS intracranial; PFS extracranial |
NCT05042791 | Exp arm I: pyrotinib + capecitabine + SRT | II | 362 | ORR in CNS | None |
Exp arm II: pyrotinib + capecitabine + WBRT | |||||
NCT03933982 | Pyrotinib + vinorelbine in pretreated pts | II | 30 | ORR in CNS | Time to progression; OS; time to radiotherapy |
NCT04303988 | Pyrotinib + temozolomide in pretreated pts | II | 59 | ORR in CNS | Clinical benefit rate for CNS; PFS; OS; first progression site; safety |
NCT03846583 | Tucatinib, abemaciclib, trastuzumab, aromatase inhibitor in: | I | 0 | MTD | ORR in CNS; DOR in CNS; extracranial ORR; PFS; OS |
• Exp arm: dose escalation | |||||
• Arm A: active brain metastases | |||||
• Arm B: surgical resection needed | |||||
• Arm C: progressive extracranial disease | |||||
NCT02423525 | Afatinib patients with BMs having failed prior therapy | I | 24 | MTD | ORR; PFS; OS |
DESTINY-BREAST 07 (NCT04538742) | T-DXd in combination to: | I/II | 245 | Safety | ORR; PFS; PFS2; DOR; OS; T-DXd serum concentration; durvalumab serum concentration; pertuzumab serum concentration; paclitaxel plasma concentration; tucatinib plasma concentration; T-DXd immunogenicity; durvalumab immunogenicity; pertuzumab immunogenicity |
• Exp arm 0: none | |||||
• Exp arm 1: durvalumab | |||||
• Exp arm 2: pertuzumab | |||||
• Exp arm 3: paclitaxel | |||||
• Exp arm 4: durvalumab + paclitaxel | |||||
• Exp arm 5: tucatinib | |||||
• Exp arm 6I: tucatinib (in pts with active BM) | |||||
• Exp arm 7: none (in pts with active BM) | |||||
NCT02768337 | Patients undergone neurosurgical resection of brain metastasis/ses | I/II | 70 | Ratio of afatinib concentration in: (resected brain metastases)/(plasma) − each measured in (ng/mL) | Safety of afatinib alone and combined with targeted low-dose radiotherapy—assessed by number of participants with treatment-related adverse events |
• Exp arm 1: afatinib only at recommended phase 2 dose (RP2D) | |||||
• Exp arm 2: afatinib RP2D + 2 Gy targeted radiotherapy | |||||
• Exp arm 3: afatinib RP2D + 4 Gy targeted radiotherapy | |||||
HER2BAT (NCT04158947) | Exp arm: T-DM1 + afatinib | II | 130 | Safety; ORR | PFS |
Control arm: T-DM1 | |||||
NCT01724606 | Sorafenib + radiotherapy | I | 21 | MTD | CNS-PFS |
NCT03423628 | AZD1390 (ATM inhibitor) + radiation therapy | I | 120 | Incidence DLTs; MTD | EFS |
• Arm A: 35 Gy of IMRT administered at daily fractions of 3.5 Gy over 10 fractions (2 weeks) | |||||
• Arm B: 30 Gy of WBRT or PBRT administered at daily fractions of 3 Gy over 10 fractions (2 weeks) | |||||
• Arm C: 60 Gy of IMRT administered at daily fractions of 2 Gy over 30 fractions (6 weeks) | |||||
NCT03765983 | GDC-0084 (PI3K inhibitor) + trastuzumab | II | 47 | ORR in CNS | CBR; DOR; PFS; OS |
InTTercePT (NCT05041842) | Tucatinib addition in pts treated with pertuzumab and trastuzumab who developed an isolated brain relapse treated with local treatment (surgery and/or radiation therapy) | II | 55 | PFS | OS; CNS-PFS; safety |
NCT05769010 | SHR-A1811 (anti-HER2 antibody-drug conjugate) in combination to: | II | 75 | ORR in CNS | ORR; PFS; safety |
• Exp arm 1: none | |||||
• Exp arm 2: pyrotinib | |||||
• Exp arm 3: bevacizumab | |||||
NCT05018702 | ARX788 (site-specific anti-HER2 antibody-drug conjugate) in pts resistant or refractory to TKI | II | 32 | Clinical benefit rate for CNS | PFS; OS; site of first progression; safety |
NCT04348747 | Anti-HER2/HER3 dendritic cell vaccine + pembrolizumab in pts with asymptomatic BM | II | 23 | ORR in CNS | Volumetric quantification of brain metastases; ORR in no-CNS; CNS-PFS; no-CNS PFS; PFS; proportion of patients who have a CNS PFS; OS; safety; proportion of patients not requiring retreatment for their brain metastasis at 6 months since the first dose of anti-HER2/3 vaccine; rate of failure of irradiated lesions |
BMBCs, brain metastatic breast cancers; pts, patients; Exp, experimental; ORR, objective response rate; CNS, central nervous system; DOR, duration of response; BM, brain metastasis; PFS, progression-free survival; OS, overall survival; MTD, maximum tolerated dose; IMRT, intensity-modulated radiation therapy; WBRT, whole-brain radiotherapy; PBRT, partial brain radiation therapy; T-DM1, trastuzumab emtansine; DLTs, dose-limiting toxicities; EFS, event-free survival; CBR, clinical benefit rate; HER2, human epidermal growth factor receptor 2.
Conclusions
HER2+ BC is known, historically, to have an aggressive behavior and poor prognosis and has been identified as a challenge for the oncologists because of limited valid therapeutic options for a long time. In the last years, the advent of new effective anti-HER2 drugs has radically improved overall survival outcomes of patients with this specific BC subtype leading to an improved prognosis compared to other subtypes, such as triple-negative BC (TNBC). However, a major incidence of BMs in these patients has been observed. Hence, the research has mainly focused on finding effective strategies to treat this subset of patients. For example, SRS demonstrated a better local control of disease with a reduced risk of cognitive impairment and consequently a better quality of life. Different targeted agents have demonstrated significant efficacy with tolerable safety profiles in HER2+, BC patients with BMs, and have already been approved for clinical use in this setting. In particular, T-DXd demonstrated promising efficacy, both in patients with untreated BMs or BMs progressing after prior anti-HER-2 treatment, with very encouraging intracranial response rates. Tyrosine kinase inhibitors also represent a fundamental weapon for the treatment of these patients. The addition of tucatinib, for example, in combination with capecitabine and trastuzumab, results in a significant increase in intracranial PFS and OS. The efficacy of these drugs is radically changing the therapeutic algorithm of these patients, leaving loco-regional treatments (neurosurgical and/or radiotherapy) as later options, with the aims of improving survival and preserving quality of life of patients. Despite these advances, clinical challenges still remain for patients with leptomeningeal metastases and for patients who have undergone cerebral progression following both loco-regional treatments and the systemic therapies discussed above. A deeper understanding of the molecular and pathogenetic mechanisms underlying BMs development, especially in HER2+ BC, could suggest novel targets and be helpful in personalizing treatment strategies. In this context, the role of CSCs and of BTB should also be further elucidated.
Acknowledgments
Funding: None.
Footnote
Reporting Checklist: The authors have completed the Narrative Review reporting checklist. Available at https://tcr.amegroups.com/article/view/10.21037/tcr-23-1126/rc
Peer Review File: Available at https://tcr.amegroups.com/article/view/10.21037/tcr-23-1126/prf
Conflicts of Interest: All authors have completed the ICMJE uniform disclosure form (available at https://tcr.amegroups.com/article/view/10.21037/tcr-23-1126/coif). M.S. serves as an unpaid editorial board member of Translational Cancer Research from December 2022 to November 2024. The other authors declare no conflict of interest.
Ethical Statement: The authors are accountable for all aspects of the work in ensuring that questions related to the accuracy or integrity of any part of the work are appropriately investigated and resolved.
Open Access Statement: This is an Open Access article distributed in accordance with the Creative Commons Attribution-NonCommercial-NoDerivs 4.0 International License (CC BY-NC-ND 4.0), which permits the non-commercial replication and distribution of the article with the strict proviso that no changes or edits are made and the original work is properly cited (including links to both the formal publication through the relevant DOI and the license). See: https://creativecommons.org/licenses/by-nc-nd/4.0/.
References
- Sung H, Ferlay J, Siegel RL, et al. Global Cancer Statistics 2020: GLOBOCAN Estimates of Incidence and Mortality Worldwide for 36 Cancers in 185 Countries. CA Cancer J Clin 2021;71:209-49. [Crossref] [PubMed]
- Loibl S, Gianni L. HER2-positive breast cancer. Lancet 2017;389:2415-29. [Crossref] [PubMed]
- Bailleux C, Eberst L, Bachelot T. Treatment strategies for breast cancer brain metastases. Br J Cancer 2021;124:142-55. [Crossref] [PubMed]
- Warrior S, Cohen-Nowak A, Kumthekar P. Modern Management and Diagnostics in HER2+ Breast Cancer with CNS Metastasis. Cancers (Basel) 2023;15:2908. [Crossref] [PubMed]
- Sperduto PW, Kased N, Roberge D, et al. The effect of tumor subtype on the time from primary diagnosis to development of brain metastases and survival in patients with breast cancer. J Neurooncol 2013;112:467-72. [Crossref] [PubMed]
- Palmieri D, Chambers AF, Felding-Habermann B, et al. The biology of metastasis to a sanctuary site. Clin Cancer Res 2007;13:1656-62. [Crossref] [PubMed]
- Riggio AI, Varley KE, Welm AL. The lingering mysteries of metastatic recurrence in breast cancer. Br J Cancer 2021;124:13-26. [Crossref] [PubMed]
- Steeg PS. The blood-tumour barrier in cancer biology and therapy. Nat Rev Clin Oncol 2021;18:696-714. [Crossref] [PubMed]
- Chen W, Hoffmann AD, Liu H, et al. Organotropism: new insights into molecular mechanisms of breast cancer metastasis. NPJ Precis Oncol 2018;2:4. [Crossref] [PubMed]
- Tomasik B, Bieńkowski M, Górska Z, et al. Molecular aspects of brain metastases in breast cancer. Cancer Treat Rev 2023;114:102521. [Crossref] [PubMed]
- De Craene B, Berx G. Regulatory networks defining EMT during cancer initiation and progression. Nat Rev Cancer 2013;13:97-110. [Crossref] [PubMed]
- Yang Z, Jiang X, Jiang X, et al. X-inactive-specific transcript: A long noncoding RNA with complex roles in human cancers. Gene 2018;679:28-35. [Crossref] [PubMed]
- Xing F, Liu Y, Wu SY, et al. Loss of XIST in Breast Cancer Activates MSN-c-Met and Reprograms Microglia via Exosomal miRNA to Promote Brain Metastasis. Cancer Res 2018;78:4316-30. [Crossref] [PubMed]
- Bryan S, Witzel I, Borgmann K, et al. Molecular Mechanisms Associated with Brain Metastases in HER2-Positive and Triple Negative Breast Cancers. Cancers (Basel) 2021;13:4137. [Crossref] [PubMed]
- Alahmari A. Blood-Brain Barrier Overview: Structural and Functional Correlation. Neural Plast 2021;2021:6564585. [Crossref] [PubMed]
- Daneman R, Prat A. The blood-brain barrier. Cold Spring Harb Perspect Biol 2015;7:a020412. [Crossref] [PubMed]
- Kaplan L, Chow BW, Gu C. Neuronal regulation of the blood-brain barrier and neurovascular coupling. Nat Rev Neurosci 2020;21:416-32. [Crossref] [PubMed]
- Thomsen MS, Routhe LJ, Moos T. The vascular basement membrane in the healthy and pathological brain. J Cereb Blood Flow Metab 2017;37:3300-17. [Crossref] [PubMed]
- Ayloo S, Gu C. Transcytosis at the blood-brain barrier. Curr Opin Neurobiol 2019;57:32-8. [Crossref] [PubMed]
- Sun H, Xu J, Dai S, et al. Breast cancer brain metastasis: Current evidence and future directions. Cancer Med 2023;12:1007-24. [Crossref] [PubMed]
- Mo F, Pellerino A, Soffietti R, et al. Blood-Brain Barrier in Brain Tumors: Biology and Clinical Relevance. Int J Mol Sci 2021;22:12654. [Crossref] [PubMed]
- Brosnan EM, Anders CK. Understanding patterns of brain metastasis in breast cancer and designing rational therapeutic strategies. Ann Transl Med 2018;6:163. [Crossref] [PubMed]
- Arvanitis CD, Ferraro GB, Jain RK. The blood-brain barrier and blood-tumour barrier in brain tumours and metastases. Nat Rev Cancer 2020;20:26-41. [Crossref] [PubMed]
- Sprowls SA, Arsiwala TA, Bumgarner JR, et al. Improving CNS Delivery to Brain Metastases by Blood-Tumor Barrier Disruption. Trends Cancer 2019;5:495-505. [Crossref] [PubMed]
- Taskar KS, Rudraraju V, Mittapalli RK, et al. Lapatinib distribution in HER2 overexpressing experimental brain metastases of breast cancer. Pharm Res 2012;29:770-81. [Crossref] [PubMed]
- Valiente M, Ahluwalia MS, Boire A, et al. The Evolving Landscape of Brain Metastasis. Trends Cancer 2018;4:176-96. [Crossref] [PubMed]
- Srinivasan ES, Deshpande K, Neman J, et al. The microenvironment of brain metastases from solid tumors. Neurooncol Adv 2021;3:v121-32. [Crossref] [PubMed]
- Bos PD, Zhang XH, Nadal C, et al. Genes that mediate breast cancer metastasis to the brain. Nature 2009;459:1005-9. [Crossref] [PubMed]
- Miyamoto S, Yagi H, Yotsumoto F, et al. Heparin-binding epidermal growth factor-like growth factor as a new target molecule for cancer therapy. Adv Exp Med Biol 2008;622:281-95. [Crossref] [PubMed]
- Tai YL, Chu PY, Lai IR, et al. An EGFR/Src-dependent β4 integrin/FAK complex contributes to malignancy of breast cancer. Sci Rep 2015;5:16408. [Crossref] [PubMed]
- Wu SY, Watabe K. The roles of microglia/macrophages in tumor progression of brain cancer and metastatic disease. Front Biosci (Landmark Ed) 2017;22:1805-29. [Crossref] [PubMed]
- Aramini B, Masciale V, Grisendi G, et al. Dissecting Tumor Growth: The Role of Cancer Stem Cells in Drug Resistance and Recurrence. Cancers (Basel) 2022;14:976. [Crossref] [PubMed]
- Zeng X, Liu C, Yao J, et al. Breast cancer stem cells, heterogeneity, targeting therapies and therapeutic implications. Pharmacol Res 2021;163:105320. [Crossref] [PubMed]
- Sirkisoon SR, Carpenter RL, Rimkus T, et al. TGLI1 transcription factor mediates breast cancer brain metastasis via activating metastasis-initiating cancer stem cells and astrocytes in the tumor microenvironment. Oncogene 2020;39:64-78. [Crossref] [PubMed]
- Su Y, Sai Y, Zhou L, et al. Current insights into the regulation of programmed cell death by TP53 mutation in cancer. Front Oncol 2022;12:1023427. [Crossref] [PubMed]
- Cancer Genome Atlas Network. Comprehensive molecular portraits of human breast tumours. Nature 2012;490:61-70. [Crossref] [PubMed]
- Garcia-Alvarez A, Papakonstantinou A, Oliveira M. Brain Metastases in HER2-Positive Breast Cancer: Current and Novel Treatment Strategies. Cancers (Basel) 2021;13:2927. [Crossref] [PubMed]
- Kuruppu D, Bhere D, Farrar CT, et al. A model of breast cancer meningeal metastases: characterization with in vivo molecular imaging. Cancer Gene Ther 2019;26:145-56. [Crossref] [PubMed]
- Nguyen A, Nguyen A, Dada OT, et al. Leptomeningeal Metastasis: A Review of the Pathophysiology, Diagnostic Methodology, and Therapeutic Landscape. Curr Oncol 2023;30:5906-31. [Crossref] [PubMed]
- Nguyen TK, Nguyen EK, Soliman H. An overview of leptomeningeal disease. Ann Palliat Med 2021;10:909-22. [Crossref] [PubMed]
- Wang R, Zhu Y, Liu X, et al. The Clinicopathological features and survival outcomes of patients with different metastatic sites in stage IV breast cancer. BMC Cancer 2019;19:1091. [Crossref] [PubMed]
- Slimane K, Andre F, Delaloge S, et al. Risk factors for brain relapse in patients with metastatic breast cancer. Ann Oncol 2004;15:1640-4. [Crossref] [PubMed]
- Pestalozzi BC, Zahrieh D, Price KN, et al. Identifying breast cancer patients at risk for Central Nervous System (CNS) metastases in trials of the International Breast Cancer Study Group (IBCSG). Ann Oncol 2006;17:935-44. [Crossref] [PubMed]
- Sperduto PW, Kased N, Roberge D, et al. Effect of tumor subtype on survival and the graded prognostic assessment for patients with breast cancer and brain metastases. Int J Radiat Oncol Biol Phys 2012;82:2111-7. [Crossref] [PubMed]
- Mills MN, Figura NB, Arrington JA, et al. Management of brain metastases in breast cancer: a review of current practices and emerging treatments. Breast Cancer Res Treat 2020;180:279-300. [Crossref] [PubMed]
- Kalkanis SN, Kondziolka D, Gaspar LE, et al. The role of surgical resection in the management of newly diagnosed brain metastases: a systematic review and evidence-based clinical practice guideline. J Neurooncol 2010;96:33-43. [Crossref] [PubMed]
- Patchell RA, Tibbs PA, Regine WF, et al. Postoperative radiotherapy in the treatment of single metastases to the brain: a randomized trial. JAMA 1998;280:1485-9. [Crossref] [PubMed]
- Vecht CJ, Haaxma-Reiche H, Noordijk EM, et al. Treatment of single brain metastasis: radiotherapy alone or combined with neurosurgery? Ann Neurol 1993;33:583-90. [Crossref] [PubMed]
- Soffietti R, Kocher M, Abacioglu UM, et al. A European Organisation for Research and Treatment of Cancer phase III trial of adjuvant whole-brain radiotherapy versus observation in patients with one to three brain metastases from solid tumors after surgical resection or radiosurgery: quality-of-life results. J Clin Oncol 2013;31:65-72. [Crossref] [PubMed]
- Brown PD, Ballman KV, Cerhan JH, et al. Postoperative stereotactic radiosurgery compared with whole brain radiotherapy for resected metastatic brain disease (NCCTG N107C/CEC·3): a multicentre, randomised, controlled, phase 3 trial. Lancet Oncol 2017;18:1049-60. [Crossref] [PubMed]
- Kayama T, Sato S, Sakurada K, et al. Effects of Surgery With Salvage Stereotactic Radiosurgery Versus Surgery With Whole-Brain Radiation Therapy in Patients With One to Four Brain Metastases (JCOG0504): A Phase III, Noninferiority, Randomized Controlled Trial. J Clin Oncol 2018; Epub ahead of print. [Crossref] [PubMed]
- Ma L, Pinnaduwage D, McDermott M, et al. Whole-procedural radiological accuracy for delivering multi-session gamma knife radiosurgery with a relocatable frame system. Technol Cancer Res Treat 2014;13:403-8. [PubMed]
- Yamamoto M, Serizawa T, Higuchi Y, et al. A Multi-institutional Prospective Observational Study of Stereotactic Radiosurgery for Patients With Multiple Brain Metastases (JLGK0901 Study Update): Irradiation-related Complications and Long-term Maintenance of Mini-Mental State Examination Scores. Int J Radiat Oncol Biol Phys 2017;99:31-40. [Crossref] [PubMed]
- Grandhi R, Kondziolka D, Panczykowski D, et al. Stereotactic radiosurgery using the Leksell Gamma Knife Perfexion unit in the management of patients with 10 or more brain metastases. J Neurosurg 2012;117:237-45. [Crossref] [PubMed]
- Ali MA, Hirshman BR, Wilson B, et al. Survival Patterns of 5750 Stereotactic Radiosurgery-Treated Patients with Brain Metastasis as a Function of the Number of Lesions. World Neurosurg 2017;107:944-951.e1. [Crossref] [PubMed]
- Andrews DW, Scott CB, Sperduto PW, et al. Whole brain radiation therapy with or without stereotactic radiosurgery boost for patients with one to three brain metastases: phase III results of the RTOG 9508 randomised trial. Lancet 2004;363:1665-72. [Crossref] [PubMed]
- Le Rhun E, Guckenberger M, Smits M, et al. EANO-ESMO Clinical Practice Guidelines for diagnosis, treatment and follow-up of patients with brain metastasis from solid tumours. Ann Oncol 2021;32:1332-47. [Crossref] [PubMed]
- Tsao M, Xu W, Sahgal A. A meta-analysis evaluating stereotactic radiosurgery, whole-brain radiotherapy, or both for patients presenting with a limited number of brain metastases. Cancer 2012;118:2486-93. [Crossref] [PubMed]
- Rades D, Haatanen T, Schild SE, et al. Dose escalation beyond 30 grays in 10 fractions for patients with multiple brain metastases. Cancer 2007;110:1345-50. [Crossref] [PubMed]
- Corti C, Antonarelli G, Criscitiello C, et al. Targeting brain metastases in breast cancer. Cancer Treat Rev 2022;103:102324. [Crossref] [PubMed]
- Stavrou E, Winer EP, Lin NU. How we treat HER2-positive brain metastases. ESMO Open 2021;6:100256. [Crossref] [PubMed]
- Baselga J, Cortés J, Kim SB, et al. Pertuzumab plus trastuzumab plus docetaxel for metastatic breast cancer. N Engl J Med 2012;366:109-19. [Crossref] [PubMed]
- Swain SM, Baselga J, Miles D, et al. Incidence of central nervous system metastases in patients with HER2-positive metastatic breast cancer treated with pertuzumab, trastuzumab, and docetaxel: results from the randomized phase III study CLEOPATRA. Ann Oncol 2014;25:1116-21. [Crossref] [PubMed]
- Lin NU, Pegram M, Sahebjam S, et al. Pertuzumab Plus High-Dose Trastuzumab in Patients With Progressive Brain Metastases and HER2-Positive Metastatic Breast Cancer: Primary Analysis of a Phase II Study. J Clin Oncol 2021;39:2667-75. [Crossref] [PubMed]
- Verma S, Miles D, Gianni L, et al. Trastuzumab emtansine for HER2-positive advanced breast cancer. N Engl J Med 2012;367:1783-91. [Crossref] [PubMed]
- Krop IE, Lin NU, Blackwell K, et al. Trastuzumab emtansine (T-DM1) versus lapatinib plus capecitabine in patients with HER2-positive metastatic breast cancer and central nervous system metastases: a retrospective, exploratory analysis in EMILIA. Ann Oncol 2015;26:113-9. [Crossref] [PubMed]
- Bartsch R, Berghoff AS, Vogl U, et al. Activity of T-DM1 in Her2-positive breast cancer brain metastases. Clin Exp Metastasis 2015;32:729-37. [Crossref] [PubMed]
- Montemurro F, Delaloge S, Barrios CH, et al. Trastuzumab emtansine (T-DM1) in patients with HER2-positive metastatic breast cancer and brain metastases: exploratory final analysis of cohort 1 from KAMILLA, a single-arm phase IIIb clinical trial Ann Oncol 2020;31:1350-8. [Crossref] [PubMed]
- von Minckwitz G, Huang CS, Mano MS, et al. Trastuzumab Emtansine for Residual Invasive HER2-Positive Breast Cancer. N Engl J Med 2019;380:617-28. [Crossref] [PubMed]
- Cortés J, Kim SB, Chung WP, et al. Trastuzumab Deruxtecan versus Trastuzumab Emtansine for Breast Cancer. N Engl J Med 2022;386:1143-54. [Crossref] [PubMed]
- Murthy RK, Loi S, Okines A, et al. Tucatinib, Trastuzumab, and Capecitabine for HER2-Positive Metastatic Breast Cancer. N Engl J Med 2020;382:597-609. [Crossref] [PubMed]
- Hurvitz SA, Hegg R, Chung WP, et al. Trastuzumab deruxtecan versus trastuzumab emtansine in patients with HER2-positive metastatic breast cancer: updated results from DESTINY-Breast03, a randomised, open-label, phase 3 trial. Lancet 2023;401:105-17. [Crossref] [PubMed]
- Bartsch R, Berghoff AS, Furtner J, et al. 280P Intracranial activity of trastuzumab-deruxtecan (T-DXd) in HER2-positive breast cancer patients with active brain metastases: Results from the first stage of the phase II TUXEDO-1 trial. Ann Oncol 2021;32:S486. [Crossref]
- Pérez-García JM, Vaz Batista M, Cortez P, et al. Trastuzumab deruxtecan in patients with central nervous system involvement from HER2-positive breast cancer: The DEBBRAH trial. Neuro Oncol 2023;25:157-66. [Crossref] [PubMed]
- Lin NU, Diéras V, Paul D, et al. Multicenter phase II study of lapatinib in patients with brain metastases from HER2-positive breast cancer. Clin Cancer Res 2009;15:1452-9. [Crossref] [PubMed]
- Bachelot T, Romieu G, Campone M, et al. Lapatinib plus capecitabine in patients with previously untreated brain metastases from HER2-positive metastatic breast cancer (LANDSCAPE): a single-group phase 2 study. Lancet Oncol 2013;14:64-71. [Crossref] [PubMed]
- Pivot X, Manikhas A, Żurawski B, et al. CEREBEL (EGF111438): A Phase III, Randomized, Open-Label Study of Lapatinib Plus Capecitabine Versus Trastuzumab Plus Capecitabine in Patients With Human Epidermal Growth Factor Receptor 2-Positive Metastatic Breast Cancer. J Clin Oncol 2015;33:1564-73. [Crossref] [PubMed]
- Parsai S, Miller JA, Juloori A, et al. Stereotactic radiosurgery with concurrent lapatinib is associated with improved local control for HER2-positive breast cancer brain metastases. J Neurosurg 2019;132:503-11. [Crossref] [PubMed]
- Freedman RA, Gelman RS, Anders CK, et al. TBCRC 022: A Phase II Trial of Neratinib and Capecitabine for Patients With Human Epidermal Growth Factor Receptor 2-Positive Breast Cancer and Brain Metastases. J Clin Oncol 2019;37:1081-9. [Crossref] [PubMed]
- Saura C, Oliveira M, Feng YH, et al. Neratinib Plus Capecitabine Versus Lapatinib Plus Capecitabine in HER2-Positive Metastatic Breast Cancer Previously Treated With ≥ 2 HER2-Directed Regimens: Phase III NALA Trial. J Clin Oncol 2020;38:3138-49. [Crossref] [PubMed]
- Lin NU, Borges V, Anders C, et al. Intracranial Efficacy and Survival With Tucatinib Plus Trastuzumab and Capecitabine for Previously Treated HER2-Positive Breast Cancer With Brain Metastases in the HER2CLIMB Trial. J Clin Oncol 2020;38:2610-9. [Crossref] [PubMed]
- Xuhong JC, Qi XW, Zhang Y, et al. Mechanism, safety and efficacy of three tyrosine kinase inhibitors lapatinib, neratinib and pyrotinib in HER2-positive breast cancer. Am J Cancer Res 2019;9:2103-19. [PubMed]
- Jiang Z, Yan M, Hu X, et al. Pyrotinib combined with capecitabine in women with HER2+ metastatic breast cancer previously treated with trastuzumab and taxanes: A randomized phase III study. J Clin Oncol 2019;37:1001. [Crossref]
- Yan M, Ouyang Q, Sun T, et al. Pyrotinib plus capecitabine for patients with human epidermal growth factor receptor 2-positive breast cancer and brain metastases (PERMEATE): a multicentre, single-arm, two-cohort, phase 2 trial. Lancet Oncol 2022;23:353-61. [Crossref] [PubMed]
- Kim HJ, Kim HP, Yoon YK, et al. Antitumor activity of HM781-36B, a pan-HER tyrosine kinase inhibitor, in HER2-amplified breast cancer cells. Anticancer Drugs 2012;23:288-97. [Crossref] [PubMed]
- Park YH, Lee KH, Sohn JH, et al. A phase II trial of the pan-HER inhibitor poziotinib, in patients with HER2-positive metastatic breast cancer who had received at least two prior HER2-directed regimens: results of the NOV120101-203 trial. Int J Cancer 2018;143:3240-7. [Crossref] [PubMed]
- Park YH, Jung KH, Sohn J, et al. 176P Poziotinib for HER2-positive metastatic breast cancer (MBC): Final clinical efficacy and safety results for long-term follow-up of the phase II NOV120101-203 trial. Ann Oncol 2022;33:S207-8. [Crossref]
- Figura NB, Rizk VT, Armaghani AJ, et al. Breast leptomeningeal disease: a review of current practices and updates on management. Breast Cancer Res Treat 2019;177:277-94. [Crossref] [PubMed]
- Kumthekar P, Lassman AB, Lin N, et al. LPTO-02. Intrathecal (IT) trastuzumab (T) for the treatment of leptomeningeal disease (LM) IN patients (PTS) with human epidermal receptor-2 positive (HER2+) CANCER: a multicenter phase 1/2 study. Neurooncol Adv 2019;1:i6. [Crossref]
- Bonneau C, Paintaud G, Trédan O, et al. Phase I feasibility study for intrathecal administration of trastuzumab in patients with HER2 positive breast carcinomatous meningitis. Eur J Cancer 2018;95:75-84. [Crossref] [PubMed]
- Alder L, Trapani D, Bradbury C, et al. Durable responses in patients with HER2+ breast cancer and leptomeningeal metastases treated with trastuzumab deruxtecan. NPJ Breast Cancer 2023;9:19. [Crossref] [PubMed]
- Le Rhun E, Devos P, Winklhofer S, et al. Prospective validation of a new imaging scorecard to assess leptomeningeal metastasis: A joint EORTC BTG and RANO effort. Neuro Oncol 2022;24:1726-35. [Crossref] [PubMed]
- Park EJ, Zhang YZ, Vykhodtseva N, et al. Ultrasound-mediated blood‐brain/blood-tumor barrier disruption improves outcomes with trastuzumab in a breast cancer brain metastasis model. Journal of Controlled Release 2012;163:277-84. [Crossref] [PubMed]
- Meng Y, Reilly RM, Pezo RC, et al. MR-guided focused ultrasound enhances delivery of trastuzumab to Her2-positive brain metastases. Sci Transl Med 2021;13:eabj4011. [Crossref] [PubMed]
- Patil R, Ljubimov AV, Gangalum PR, et al. MRI virtual biopsy and treatment of brain metastatic tumors with targeted nanobioconjugates: nanoclinic in the brain. ACS Nano 2015;9:5594-608. [Crossref] [PubMed]
- Hamilton AM, Aidoudi-Ahmed S, Sharma S, et al. Nanoparticles coated with the tumor-penetrating peptide iRGD reduce experimental breast cancer metastasis in the brain. J Mol Med (Berl) 2015;93:991-1001. [Crossref] [PubMed]
- Fan Y, Marioli M, Zhang K. Analytical characterization of liposomes and other lipid nanoparticles for drug delivery. J Pharm Biomed Anal 2021;192:113642. [Crossref] [PubMed]
- Mohammadian Haftcheshmeh S, Zamani P, et al. Immunoliposomes bearing lymphocyte activation gene 3 fusion protein and P5 peptide: A novel vaccine for breast cancer. Biotechnol Prog 2021;37:e3095. [Crossref] [PubMed]
- Amin M, Pourshohod A, Kheirollah A, et al. Specific delivery of idarubicin to HER2-positive breast cancerous cell line by trastuzumab-conjugated liposomes. J Drug Deliv Sci Technol 2018;47:209-14. [Crossref]
- Lingappa M, Song H, Thompson S, et al. Immunoliposomal Delivery of 213Bi for α-Emitter Targeting of Metastatic Breast Cancer. Cancer Res. 2010;70:6815-23. [Crossref] [PubMed]
- Waelti E, Wegmann N, Schwaninger R, et al. Targeting her-2/neu with antirat Neu virosomes for cancer therapy. Cancer Res 2002;62:437-44. [PubMed]
- Shi X, Cheng Q, Hou T, et al. Genetically Engineered Cell-Derived Nanoparticles for Targeted Breast Cancer Immunotherapy. Mol Ther 2020;28:536-47. [Crossref] [PubMed]