Microtubule targeting agents in glioma
Microtubule targeting agents: mechanisms of action and classification
Microtubules are dynamic tubular polymers of α- and β-tubulin which provide structural integrity, promote migration, transport of molecules, vesicles and organelles and play essential roles during cell division by forming the mitotic spindles, orchestrating the transport and separation of chromosomes and cytokinesis. Blocking the microtubule polymerization-dynamics leads to disruption of cellular division, mitotic arrest at the metaphase-anaphase transition and induction of apoptosis (1). Microtubules are thus a good target for anticancer therapy. Many tubulin-binding agents (TBAs) have been purified from natural products and synthesized to be used as therapy for a variety of cancers (2).
Based on their binding-site and their mechanism of action TBAs can be classified in three categories (3): (I) the Vinca alcaloid group including vinblastine and vincristine, vindesine, vinorelbine, vinflunine, cryptophycin, dolastatins and others, compounds which bind to high-affinity sites at the microtubule plus end (Vinca binding domain) and induce microtubule destabilization. These drugs have long been used in the treatment of pediatric and adult hematological malignancies and continue to be employed for the treatment of various solid tumors in several combinations; (II) taxane compounds, including paclitaxel, docetaxel, cabazitaxel and the epothilone group, bind to β-tubulin on the inside of the microtubule (Taxane site) (4), induce microtubule polymerization at high doses and suppress microtubule dynamics at lower doses; (III) the Colchicine group includes colchicine (historically used to treat gout), combretastatins and its many synthetic derivates (AVE8062A, CA-1-P, CA-4-P, ZD6126) (5), 2-Methoxy-estradiol, ABT-751, E7010, etc.); these compounds bind to the colchicine site of free β-tubulin molecules generating tubulin-colchicine complexes which polymerize into the microtubule, altering its structure and suppressing microtubule dynamics. The chemical structure of several TBAs is presented in Figure 1. Vinka alkaloids and members of the colchicine group are also categorized as microtubule polymerization inhibitors, or destabilizing agents, whereas the taxane compounds are thought of as microtubule stabilizing agents (Figure 2).
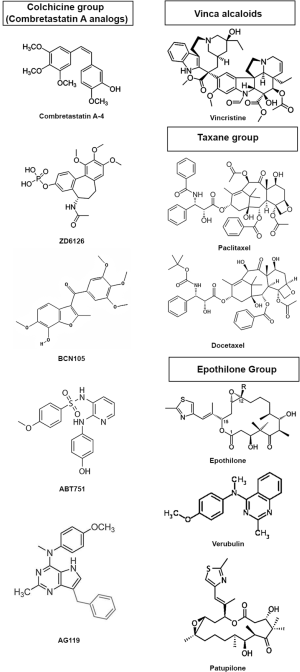
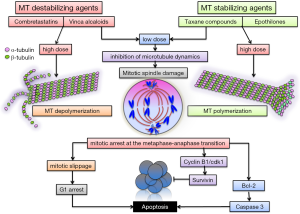
Interestingly TBAs, particularly members of the colchicine group, but also vinka alkaloids, exhibit anti-vascular effects, inducing hemorrhagic necrosis and decrease of blood flow within tumors in preclinical cancer models (6,7). This effect is thought to be due to the action of TBAs on cytoskeletal microtubules and is primarily evident in the tumor vasculature and not in the vessels of healthy organs. The tumor specificity of the anti-vascular effects of TBA’s is attributed to the differences between the cytoskeletal architecture of endothelial cells in newly formed tumor vessels and established normal blood vessels (8). It has been proposed that Combretastatin A-4 (CA-4) induces depolymerization of cytoskeletal microtubules, activation of stress signaling pathways, alterations of endothelial cell membrane permeability, membrane blebbing, detachment and necrosis, increased vascular permeability, red blood cell stacking, edema and loss of blood flow (5). Since CA-4 has limited solubility and bio-availability, several water soluble analogs (BPR0L075, BNC105, etc.) have been synthesized and tested in preclinical cancer models (5) and also in glioblastoma (GBM) (9-11).
Side effects and multi drug resistance
Drawbacks for the use of TBAs in oncology include side effects induced by these drugs, as they are inherently non-specific, targeting microtubules in cancerous and non-cancerous cells, and by the development of drug resistance. Most commonly observed are peripheral neuropathies and autonomic neuropathies, neuronal activity being highly dependent on proper functioning of microtubules (12,13). Frequent side effects are also caused by myelosupression, bone marrow cells relying heavily on cell division to maintain blood cell homeostasis (14).
Other limitations related to the use of TBA are represented by the heterogeneity within tumors, which, unlike cancer cell lines used in vitro, display lower and variable rates of mitosis and are thus able to escape targeting by anti-mitotic drugs. A concern is represented by the “mitotic slippage” of cancer cells targeted with TBA, which upon mitotic arrest, do not undergo apoptosis, but remain viable, acquiring multiploidic states, increased genetic instability and malignancy (15,16).
Resistance to microtubule-targeting agents is often due to drug efflux by ATP-binding cassette (ABC) transporters such as P-glycoprotein (Pgp). These transporters are energy-dependent membrane pumps involved in the transport of a wide variety of substrates across the plasma membrane and are integral members of specialized compartments like the blood-brain, the blood-testis, and the placental barriers. In the context of drug treatment these pumps actively eliminate drugs from cells, showing preference for many of the naturally occurring anticancer agents like taxanes, the vinca alkaloids, anthracyclines, epipodophyllotoxins and others, and are responsible for the development of multidrug resistance (17,18). Other described mechanisms of resistance include: altered expression of microtubules associated proteins (MAP) (19,20), aberrant and overexpression of specific β-tubulin isotypes, mutation within the β-tubulin protein itself (21) or impaired apoptotic signaling downstream of the mitotic arrest induced by TBAs. In the brain, β-III tubulin is not normally found in glial cells, but rather is a neuronal marker with high expression in young neurons. Expression of β-III tubulin in glial tumors is however aberrant, and correlates with resistance to taxanes and vinca alkaloids and also with increased malignancy, being found primarily in high grade glioma: astrocytoma (WHO-III) and glioblastomas (GBM: WHO-IV) (22). β-III tubulin is thus a more specific target for TBAs in glioma and studies have sought to find compounds which can target it directly. Ixabepilone, a derivate of epothilone B, has high antitumor activity in taxane resistant tumors by preferentially targeting αβIII-microtubules in cells expressing high levels of βIII-tubulin, however this drug cannot cross the blood brain barrier (BBB) (23), highlighting the numerous aspects to consider when designing therapies for central nervous system (CNS) malignancies with anti-tubulin agents.
Current use of microtubule targeting agents in the treatment of glioblastoma (GBM)
The current treatment for GBM, the deadliest and most common form of primary malignant brain cancer can only offer patients a 5-year survival rate of 5.1% (24). While immunotherapy is increasingly viewed as the fourth arm in the standard of care for GBM, added to maximal possible surgical rejection, radiation and chemotherapy with temozolamide (TMZ) (25-27), so far, large scale clinical trials have not shown long term survival benefit in GBM patients treated with immunotherapy (28). Therefore, the development of new antimitotic TBAs with increased specificity, decreased toxicity, capable of crossing the BBB and overcoming mechanisms of drug induced resistance is an attractive prospect (29). Employing digital modeling algorithms, several new compounds designed to specifically down-regulate β-III tubulin have been discovered: IDN5390111 and other seco-taxanes (30) as well as epothilones, such as ixabepilone and patupilone (EpothiloneB). These drugs are active against taxane resistant epithelial tumors and act by both suppressing the dynamic instability of microtubules in the mitotic spindle and by antagonizing the assembly of cytoskeletal microtubules leading to inhibition of migration and invasion of GBM cells (31,32).
Results from a phase I/II clinical trial which tested Patupilone in recurrent GBM, reported that the drug was relatively well tolerated, with no hematological side-effects, no cognitive changes, but with development of reversible sensory neuropathy in two of nine patients (33). The study demonstrated that the drug accumulates within the tumor tissue and that two patients benefited from long-term recurrence free survival (9.75 and 22 months) (33). Patupilone was also tested in preclinical studies of medulloblastoma, as a less toxic alternative to vincristine (34). Sagopilone (ZK-EPO), an analogue of epothilone B, which can cross the BBB, having demonstrated promising results in rodent models of GBM (35), has been tested in 15 patients with recurrent GBM (36). After four cycles of treatment, five patients achieved the 6 months progression free survival, however six patients developed neuropathy (36). A phase II multicenter follow-up trial in 37 patients with recurrent GBM, was unable to demonstrate antitumor activity, progression free survival at 6 months being only 6.7% and 46% of patients developed moderate to severe neuropathy (37).
Verubulin (MPC-0827, Azixa) is a quinazoline-derived small molecule, which has been demonstrated to inhibit microtubule formation, to cross the BBB and to induce mitotic arrest and apoptosis in several cancer cell lines (38). Verubulin has also been tested in a phase II clinical trial of 56 patients with recurrent GBM, either refractory or naïve for bevacizumab. While the drug was relatively well tolerated, this single agent treatment had little to no anti-tumor activity. Nonetheless, bevacizumab-naïve patients seemed to have fared better than the refractory group (median survival: 9.5 months compared to 3.4 months).
During the last three years, aided by the increasing availability and sophistication of in silico modeling algorithms and of high-throughput cell based screening assays, intense efforts to discover new antimitotic therapeutic agents have led to the discovery of a vast array of novel analogs of colchicine and combretastatin-A, some displaying both anti-mitotic as well as anti-vascular activity like: MDS-11P (39), indole compounds II and IAT (40), cis-constrained analogs of combretastatin A4: compounds 11 and 19 (41), cis 3-b (42), DAT-230 (43), ketone 24-b (44), phenothiazine derivative 21 (45), 1,1-diaryl-2-methoxyethylenes 4b and 4e (46), combretazet-3 (CAZ-3) (47), benzoimidazole analog 5 (48), compound 3-HCl (AG119) (49), furocoumarin analogs of combretastatins: compounds 2 and 3 (50), and the IsoCombretaQuinazolines (isoCoQ) compounds 4b, 4c and 4d (51).
In an effort to design antimitotic agents which can act on multiple targets on cancer cells and starting from the structural similarity of tubulin binding agents with receptor tyrosine kinase (RTK) inhibitors, Gangjee et al. have designed several analogs of combretastatin A to potentially act as inhibitors of both microtubule dynamics and RTKs (49). The authors showed that compound 3-HCl (later called AG119), a water soluble derivate of pyrrolo [3,2-d] pyrimidine, previously reported to have antiangiogenic, antimetastatic, and antitumor activity (52), when modified to incorporate a benzyl group acquires anti VEGFR2 activity, comparable to sunitinib. This was documented in an in vitro assay of tyrosine phosphorylation following stimulation with VEGF. The authors also showed that blood vessel formation in the chicken chorio-allantoic membrane is inhibited by AG119 at concentrations comparable to sunitinib (IC50 for 3-HCl =2.9 μM, and for sunitinib 1.3 μM). In vitro, AG119 bound to the colchicine site on microtubules and inhibited tubulin assembly, albeit 18-fold less than combretastatin A-4P (CA-4P). Tested on the NCI 60 tumor cell panel this compound induced growth inhibition in all cell lines at a nanomolar concentration including in the astrocytoma cell line SF-268, the gliosarcoma cell line SF-539 and the GBM cell lines SF295, SNB-19, SNB-75 and U251. 3-HCl was also effective in β-III tubulin overexpressing HeLa cells, resistant to paclitaxel, and in PgP overexpression ovarian cancer cells. The authors demonstrate that the compound induced G2/M mitotic arrest similar to CA-4P and docetaxel. Furthermore, AG119 inhibited tumor growth and tumor vascular density with higher efficacy than sunitinib, docetaxel or temozolomide (TMZ) when tested in in vivo flank tumors of either MDA-MB-435 breast cancer cells or U251 GBM cells. This compound was also effective in the 4T1 orthotopic breast cancer model where it also inhibited the formation of lung metastases. The authors conclude that AG119 is a microtubule depolymerization anti-cancer agent, superior to TMZ, docetaxel and sunitinib in preclinical cancer models, with concurrent an anti-angiogenic anti-VEGFR2 effect.
Subsequently, AG119 was tested, in an orthotopic, syngeneic GBM model, using GL261 cells. The in vivo anti-tumor effect of AG119 was compared with three other treatments: anti-VEGF antibodies, antibodies against c-Met, a RTK increased in GBMs and TMZ (53). Survival analysis of these four treatment groups (5–7 animals per group) indicated that the median survival was 20 days for untreated animals, 27 days for anti-VEGF treated, 28 days in the c-Met antibody group, 29 days in the AG119 treated animals and 34 days in the TMZ treated group. All treatment groups significantly increased survival when compared to the untreated group. MRI analysis showed a significant decrease of the tumor volume for all treatment groups. Analysis of blood perfusion into the tumors demonstrated significantly decreased blood flow in both AG119 and TMZ treated animals when compared to untreated controls. The inhibitory effect of AG119 and TMZ on TMZ sensitive and resistant human cell lines (U251 and T98G) was also analyzed, showing that the IC50 of AG119 wasn’t significantly different between U251 and T98G, suggesting that the anti-tumor effect of AG119 is not dependent of the MGMT methylation status. The authors conclude that AG119 significantly increases survival of GL261 glioma-bearing animals and decreases tumor vascularity warranting further explorations of this small molecule therapeutic in GBM. Comparing the results of the U251 flank tumors treated with AG119 (49) with the orthotopic GL26 tumor model in this study (53) one can notice that TMZ fared better in the orthotopic model, whereas AG119 seemed to have a more potent effect in flank tumors with respect to both tumor growth and tumor vascularity. This difference could be accounted for by the difference in BBB penetration or, possibly by differences in the immune response to treatment, since one model uses immune compromised mice, whereas animals in the syngeneic orthotopic model are immuno-competent. The myelo-suppressive effect of antimitotic agents is well documented; however, it was not explored in this study. The authors suggest that the decrease in perfusion following AG119 treatment is due to the inhibition of VEGFR2, based on data from the previous study, however, the anti-vascular effect of AG119 could also be a consequence of altering the cytoskeletal microtubules in vascular endothelial cells, as it has been shown before for several compounds derived from CA-4, or by increased necrosis within the tumor. This study highlights the need to test novel drugs for GBM, in models which more closely resemble the human disease, i.e., orthotopic location, also the use of immuno-competent animals, given the major role played by the immune system in modulating response to treatment.
An exciting group of new Combretastatin A-4 derivates has been recently developed, in which activity can be switched “on” and “off” by visible light. These drugs are represented by the photoisomerizable azo-Combretastatin A4 (54) and the closely related photostatins (55). Upon exposure to light, these compounds isomerize from an inactive trans, into an active cis conformation, allowing for a precise control of the activity on microtubule dynamics. These drugs open up the possibility of directed, specific, temporally and spatially controlled activation of anti-tubulin agents allowing for targeted antimitotic and anti-vascular therapy in various cancers, an elegant avenue to pursue and overcome the many drawbacks associated with systemic side-effects of TBAs.
In summary, our knowledge of the mechanisms of action, resistance and side-effects as well as our toolbox of small molecular compounds with tubulin binding activity has increased exponentially in the last decade. We are getting closer to the design of therapeutic agents with increased specificity, decreased toxicity, capable of crossing the BBB and overcoming mechanisms of drug induced resistance. Efforts so far have shown that single agent therapies for GBM fail, thus more successful therapies should entail a combination of agents, including surgery, radiation, multi-drug chemotherapy and immunotherapy with immune checkpoint blockade. Inhibitors of microtubule dynamics, if made selective, effective and safe, represent an important class of molecules to consider for GBM treatment. Nonetheless, rigorous, large scale, objective studies which evaluate all aspects of treatment: anti-tumor activity, BBB permeability, resistance mechanisms, side effects are required in preclinical trials before translating these treatments into the clinic.
Acknowledgments
Funding: This work was supported by National Institutes of Health/National Institute of Neurological Disorders & Stroke (NIH/NINDS) Grants R01-NS094804, R01-NS074387, R21-NS091555, and R37-NS094804 to M.G.C.; NIH/NINDS Grants R01-NS076991, R01-NS082311, R21-NS084275, and R01-096756 to P.R.L.; Leah’s Happy Heart Foundation, Chad Tough Foundation, and The Phase One Foundation to M.G.C. and P.R.L.; the Department of Neurosurgery, University of Michigan Medical School; the Michigan Institute for Clinical and Health Research, NIH 2UL1-TR000433; the Cancer Biology Training Grant, NIH/NCI T32-CA009676; the Training in Clinical and Basic Neuroscience, NIH/NINDS T32-NS007222; and the Medical Scientist Training Program, NIH/NIGMS T32-GM007863.
Footnote
Provenance and Peer Review: This article was commissioned and reviewed by the Section Editor Hao Xu, MD (Department of Neurosurgery, Tongji Hospital, Huazhong University of Science and Technology, Wuhan, China).
Conflicts of Interest: Both authors have completed the ICMJE uniform disclosure form (available at http://dx.doi.org/10.21037/tcr.2016.06.12). The authors have no conflicts of interest to declare.
Ethical Statement: The authors are accountable for all aspects of the work in ensuring that questions related to the accuracy or integrity of any part of the work are appropriately investigated and resolved.
Open Access Statement: This is an Open Access article distributed in accordance with the Creative Commons Attribution-NonCommercial-NoDerivs 4.0 International License (CC BY-NC-ND 4.0), which permits the non-commercial replication and distribution of the article with the strict proviso that no changes or edits are made and the original work is properly cited (including links to both the formal publication through the relevant DOI and the license). See: https://creativecommons.org/licenses/by-nc-nd/4.0/.
References
- Jordan MA, Wendell K, Gardiner S, et al. Mitotic block induced in HeLa cells by low concentrations of paclitaxel (Taxol) results in abnormal mitotic exit and apoptotic cell death. Cancer Res 1996;56:816-25. [PubMed]
- Dumontet C, Jordan MA. Microtubule-binding agents: a dynamic field of cancer therapeutics. Nat Rev Drug Discov 2010;9:790-803. [Crossref] [PubMed]
- Jordan MA, Wilson L. Microtubules as a target for anticancer drugs. Nat Rev Cancer 2004;4:253-65. [Crossref] [PubMed]
- Nogales E, Wolf SG, Khan IA, et al. Structure of tubulin at 6.5 A and location of the taxol-binding site. Nature 1995;375:424-7. [Crossref] [PubMed]
- Rajak H, Dewangan PK, Patel V, et al. Design of combretastatin A-4 analogs as tubulin targeted vascular disrupting agent with special emphasis on their cis-restricted isomers. Curr Pharm Des 2013;19:1923-55. [Crossref] [PubMed]
- Tozer GM, Prise VE, Wilson J, et al. Mechanisms associated with tumor vascular shut-down induced by combretastatin A-4 phosphate: intravital microscopy and measurement of vascular permeability. Cancer Res 2001;61:6413-22. [PubMed]
- Dark GG, Hill SA, Prise VE, et al. Combretastatin A-4, an agent that displays potent and selective toxicity toward tumor vasculature. Cancer Res 1997;57:1829-34. [PubMed]
- Prise VE, Honess DJ, Stratford MR, et al. The vascular response of tumor and normal tissues in the rat to the vascular targeting agent, combretastatin A-4-phosphate, at clinically relevant doses. Int J Oncol 2002;21:717-26. [PubMed]
- Subbiah IM, Lenihan DJ, Tsimberidou AM. Cardiovascular toxicity profiles of vascular-disrupting agents. Oncologist 2011;16:1120-30. [Crossref] [PubMed]
- Kuo CC, Hsieh HP, Pan WY, et al. BPR0L075, a novel synthetic indole compound with antimitotic activity in human cancer cells, exerts effective antitumoral activity in vivo. Cancer Res 2004;64:4621-8. [Crossref] [PubMed]
- Krishnan A, Wilson J, Leung HY. Approaches for developing novel microtubule targeting agents (MTAs) for therapeutic exploitation. Curr Pharm Des 2012;18:2804-10. [Crossref] [PubMed]
- Dubey J, Ratnakaran N, Koushika SP. Neurodegeneration and microtubule dynamics: death by a thousand cuts. Front Cell Neurosci 2015;9:343. [Crossref] [PubMed]
- Gornstein E, Schwarz TL. The paradox of paclitaxel neurotoxicity: Mechanisms and unanswered questions. Neuropharmacology 2014;76:175-83. [Crossref] [PubMed]
- Mukhtar E, Adhami VM, Mukhtar H, et al. Targeting microtubules by natural agents for cancer therapy. Mol Cancer Ther 2014;13:275-84. [Crossref] [PubMed]
- Chan KS, Koh CG, Li HY. Mitosis-targeted anti-cancer therapies: where they stand. Cell Death Dis 2012;3:e411 [Crossref] [PubMed]
- Chen JG, Yang CP, Cammer M, et al. Gene expression and mitotic exit induced by microtubule-stabilizing drugs. Cancer Res 2003;63:7891-9. [PubMed]
- Fojo T, Menefee M. Mechanisms of multidrug resistance: the potential role of microtubule-stabilizing agents. Ann Oncol 2007;18:v3-8. [Crossref] [PubMed]
- Ambudkar SV, Kimchi-Sarfaty C, Sauna ZE, et al. P-glycoprotein: from genomics to mechanism. Oncogene 2003;22:7468-85. [Crossref] [PubMed]
- Galmarini CM, Kamath K, Vanier-Viornery A, et al. Drug resistance associated with loss of p53 involves extensive alterations in microtubule composition and dynamics. Br J Cancer 2003;88:1793-9. [Crossref] [PubMed]
- Bonnet C, Boucher D, Lazereg S, et al. Differential binding regulation of microtubule-associated proteins MAP1A, MAP1B, and MAP2 by tubulin polyglutamylation. J Biol Chem 2001;276:12839-48. [Crossref] [PubMed]
- Kavallaris M. Microtubules and resistance to tubulin-binding agents. Nat Rev Cancer 2010;10:194-204. [Crossref] [PubMed]
- Katsetos CD, Dráberová E, Legido A, et al. Tubulin targets in the pathobiology and therapy of glioblastoma multiforme. I. Class III beta-tubulin. J Cell Physiol 2009;221:505-13. [Crossref] [PubMed]
- Dumontet C, Jordan MA, Lee FF. Ixabepilone: targeting betaIII-tubulin expression in taxane-resistant malignancies. Mol Cancer Ther 2009;8:17-25. [Crossref] [PubMed]
- Ostrom QT, Gittleman H, Fulop J, et al. CBTRUS Statistical Report: Primary Brain and Central Nervous System Tumors Diagnosed in the United States in 2008-2012. Neuro Oncol 2015;17:iv1-iv62. [Crossref] [PubMed]
- Fecci PE, Heimberger AB, Sampson JH. Immunotherapy for primary brain tumors: no longer a matter of privilege. Clin Cancer Res 2014;20:5620-9. [Crossref] [PubMed]
- Reardon DA, Freeman G, Wu C, et al. Immunotherapy advances for glioblastoma. Neuro Oncol 2014;16:1441-58. [Crossref] [PubMed]
- Calinescu AA, Kamran N, Baker G, et al. Overview of current immunotherapeutic strategies for glioma. Immunotherapy 2015;7:1073-104. [Crossref] [PubMed]
- Malkki H. Trial Watch: Glioblastoma vaccine therapy disappointment in Phase III trial. Nat Rev Neurol 2016;12:190. [Crossref] [PubMed]
- Katsetos CD, Reginato MJ, Baas PW, et al. Emerging microtubule targets in glioma therapy. Semin Pediatr Neurol 2015;22:49-72. [Crossref] [PubMed]
- Pepe A, Sun L, Zanardi I, et al. Novel C-seco-taxoids possessing high potency against paclitaxel-resistant cancer cell lines overexpressing class III beta-tubulin. Bioorg Med Chem Lett 2009;19:3300-4. [Crossref] [PubMed]
- Ferrandina G, Mariani M, Andreoli M, et al. Novel drugs targeting microtubules: the role of epothilones. Curr Pharm Des 2012;18:2793-803. [Crossref] [PubMed]
- Henry WI, Dubois J, Quick QA. The microtubule inhibiting agent epothilone B antagonizes glioma cell motility associated with reorganization of the actin-binding protein α-actinin 4. Oncol Rep 2011;25:887-93. [PubMed]
- Oehler C, Frei K, Rushing EJ, et al. Patupilone (epothilone B) for recurrent glioblastoma: clinical outcome and translational analysis of a single-institution phase I/II trial. Oncology 2012;83:1-9. [Crossref] [PubMed]
- Oehler C, von Bueren AO, Furmanova P, et al. The microtubule stabilizer patupilone (epothilone B) is a potent radiosensitizer in medulloblastoma cells. Neuro Oncol 2011;13:1000-10. [Crossref] [PubMed]
- Hoffmann J, Fichtner I, Lemm M, et al. Sagopilone crosses the blood-brain barrier in vivo to inhibit brain tumor growth and metastases. Neuro Oncol 2009;11:158-66. [Crossref] [PubMed]
- Silvani A, Gaviani P, Fiumani A, et al. Systemic sagopilone (ZK-EPO) treatment of patients with recurrent malignant gliomas. J Neurooncol 2009;95:61-4. [Crossref] [PubMed]
- Stupp R, Tosoni A, Bromberg JE, et al. Sagopilone (ZK-EPO, ZK 219477) for recurrent glioblastoma. A phase II multicenter trial by the European Organisation for Research and Treatment of Cancer (EORTC) Brain Tumor Group. Ann Oncol 2011;22:2144-9. [Crossref] [PubMed]
- Kasibhatla S, Baichwal V, Cai SX, et al. MPC-6827: a small-molecule inhibitor of microtubule formation that is not a substrate for multidrug resistance pumps. Cancer Res 2007;67:5865-71. [Crossref] [PubMed]
- Deng ZT, Feng T, Wang P, et al. Effects of the novel vascular targeting agent MDS-11P on tumor vascularity and its antitumor activity. Biochem Pharmacol 2011;82:1832-42. [Crossref] [PubMed]
- Li CM, Lu Y, Chen J, et al. Orally bioavailable tubulin antagonists for paclitaxel-refractory cancer. Pharm Res 2012;29:3053-63. [Crossref] [PubMed]
- Mur Blanch N, Chabot GG, Quentin L, et al. In vitro and in vivo biological evaluation of new 4,5-disubstituted 1,2,3-triazoles as cis-constrained analogs of combretastatin A4. Eur J Med Chem 2012;54:22-32. [Crossref] [PubMed]
- Nguyen TT, Lomberget T, Tran NC, et al. Synthesis and biological evaluation of novel heterocyclic derivatives of combretastatin A-4. Bioorg Med Chem Lett 2012;22:7227-31. [Crossref] [PubMed]
- Qiao F, Zuo D, Shen X, et al. DAT-230, a novel microtubule inhibitor, exhibits potent anti-tumor activity by inducing G2/M phase arrest, apoptosis in vitro and perfusion decrease in vivo to HT-1080. Cancer Chemother Pharmacol 2012;70:259-70. [Crossref] [PubMed]
- Sitnikov N, Velder J, Abodo L, et al. Total synthesis of indole-derived allocolchicine analogues exhibiting strong apoptosis-inducing activity. Chemistry 2012;18:12096-102. [Crossref] [PubMed]
- Abuhaie CM, Bîcu E, Rigo B, et al. Synthesis and anticancer activity of analogues of phenstatin, with a phenothiazine A-ring, as a new class of microtubule-targeting agents. Bioorg Med Chem Lett 2013;23:147-52. [Crossref] [PubMed]
- Aziz J, Brachet E, Hamze A, et al. Synthesis, biological evaluation, and structure-activity relationships of tri- and tetrasubstituted olefins related to isocombretastatin A-4 as new tubulin inhibitors. Org Biomol Chem 2013;11:430-42. [Crossref] [PubMed]
- Greene LM, Wang S, O'Boyle NM, et al. Combretazet-3 a novel synthetic cis-stable combretastatin A-4-azetidinone hybrid with enhanced stability and therapeutic efficacy in colon cancer. Oncol Rep 2013;29:2451-8. [PubMed]
- Zhou J, Jin J, Zhang Y, et al. Synthesis and antiproliferative evaluation of novel benzoimidazole-contained oxazole-bridged analogs of combretastatin A-4. Eur J Med Chem 2013;68:222-32. [Crossref] [PubMed]
- Gangjee A, Pavana RK, Ihnat MA, et al. Discovery of antitubulin agents with antiangiogenic activity as single entities with multitarget chemotherapy potential. ACS Med Chem Lett 2014;5:480-4. [Crossref] [PubMed]
- Lipeeva AV, Shults EE, Shakirov MM, et al. Synthesis and cytotoxic activity of a new group of heterocyclic analogues of the combretastatins. Molecules 2014;19:7881-900. [Crossref] [PubMed]
- Soussi MA, Provot O, Bernadat G, et al. IsoCombretaQuinazolines: Potent Cytotoxic Agents with Antitubulin Activity. ChemMedChem 2015;10:1392-402. [Crossref] [PubMed]
- Gangjee A, Pavana RK, Li W, et al. Novel water-soluble substituted pyrrolo[3,2-d]pyrimidines: design, synthesis, and biological evaluation as antitubulin antitumor agents. Pharm Res 2012;29:3033-9. [Crossref] [PubMed]
- Towner RA, Ihnat M, Saunders D, et al. A new anti-glioma therapy, AG119: pre-clinical assessment in a mouse GL261 glioma model. BMC Cancer 2015;15:522. [Crossref] [PubMed]
- Engdahl AJ, Torres EA, Lock SE, et al. Synthesis, Characterization, and Bioactivity of the Photoisomerizable Tubulin Polymerization Inhibitor azo-Combretastatin A4. Org Lett 2015;17:4546-9. [Crossref] [PubMed]
- Borowiak M, Nahaboo W, Reynders M, et al. Photoswitchable Inhibitors of Microtubule Dynamics Optically Control Mitosis and Cell Death. Cell 2015;162:403-11. [Crossref] [PubMed]