Advantages of IMRT optimization with MCO compared to IMRT optimization without MCO in reducing small bowel high dose index for cervical cancer patients—individualized treatment options
Highlight box
Key findings
• In this study, we found that the individualizing intensity-modulated radiation therapy (IMRT) plan using the multi-criteria optimization (MCO) method provides better protection of other organs at risk (OARs) and equivalently in planning treatment volume (PTV) coverage, while lowering the high-dose index in the small bowel as much as possible for patients with cervical cancer, thus providing a rapid approach to achieving individualized IMRT for cervical cancer patients.
What is known and what is new?
• Traditional IMRT plans are time-consuming and labor-intensive, while MCO helps reduce optimization time.
• Investigated the dosimetric changes in the treatment target volume and the OARs after reducing high-dose exposure to the small bowel through the application of the Eclipse MCO program.
What is the implication, and what should change now?
• IMRT optimization with MCO has advantages in reducing the small bowel high-dose index of cervical cancer patients, and can provide a rapid method for individualized treatment of cervical cancer patients.
Introduction
Cervical cancer is one of the diseases with high incidence and mortality among malignant tumors of the female reproductive system (1). It has been reported that the global incidence of cervical cancer in women in 2018 was about 569,000 cases, and the number of deaths exceeded for 361,000 cases (2). Surgery, external irradiation, brachytherapy (radiotherapy), and chemotherapy are the main treatments used for cervical cancer. Of these, among which radiotherapy plays a crucial role in the treatment of cervical cancer (3,4).
In the field of radiation therapy, there have been advancements in radiation treatment techniques. Compared to the earlier 3D conformal radiation techniques (3D-CRT), techniques such as intensity-modulated radiation therapy (IMRT), volumetric-modulated arc therapy (VMAT), and image-guided radiation therapy (IGRT) achieve adequate dose coverage of the tumor while minimizing radiation to surrounding normal tissues (5-7). IMRT allows higher radiation doses to be delivered to the tumor while reducing the toxicity to the surrounding normal tissue, ultimately improving the treatment efficacy. Due to these advantages, IMRT is widely used in clinical treatment protocols for cervical cancer (7).
The quality of the IMRT plan depends on how well it meets the clinical objectives during the planning phase. These objectives primarily include target area dose coverage and preservation of normal organs. These two conflicting parameters are weighed and optimized by the dosimetrist based on priorities. Ultimately, the clinical intent of the physician is translated into a truly executable treatment plan. However, multiple optimizations are often required to obtain an IMRT plan with better benefits, which can also be considered a trial-and-error process. Especially in cervical cancer radiotherapy, the small bowel of some patients is sensitive to radiation. This necessitates lowering the D2cc (D2cc is the minimal dose to the 2 cm3 of the small bowel receiving the maximal dose) level below 5,200 cGy to reduce radiotherapy side effects, with a total dose of 5,040 cGy delivered to the patient (8). However, the D2cc index is an unclear optimization target compared to the maximum dose, which would consume a significant amount of time and effort until an acceptable and optimal clinical delivery plan can be created. In addition, the quality of the plan can be influenced by the experience of the dosimetrist.
Multi-criteria optimization (MCO) is a new optimization method that operates using Pareto surfaces of optimal plans (9-11). Its efficiency has been demonstrated in terms of dose quality and planning time (12-16). In recent years, Varian has developed the MCO tool in Eclipse (commercial treatment planning system), which can be manipulated by visualized trade-off exploration. This advanced tool within Eclipse facilitates the creation of Pareto surfaces, which graphically represent the ideal dose distribution, providing physicians and dosimetrists with invaluable real-time dosimetry parameters. One of the pivotal factors significantly influencing the outcomes of optimization in MCO IMRT plans lies in the selection of optimization objectives and the associated weightings. Physicians and dosimetrists play a crucial role in achieving optimal planning for their intended treatment objectives by meticulously adjusting the balance of these specific optimization goals. Consequently, MCO streamlines the process, bypassing time-consuming iterative calculations and assisting physicians and dosimetrists in achieving more favorable IMRT plans.
However, to the best of our knowledge, there is a notable gap in existing research when it comes to the utilization of the Eclipse MCO project within the context of participation in IMRT planning for cervical cancer radiation therapy. Specifically, there has been limited exploration of the dosimetric effects on adjacent normal tissues and organs when implementing MCO to mitigate high-dose exposure to the small bowel. Therefore, the primary objective of this study is to identify and analyze the dosimetric changes in the treatment target volume and the surrounding organs at risk (OARs) after reducing high-dose exposure to the small bowel through the application of the Eclipse MCO program. This research has the potential to enhance the efficiency of treatment planning and prognosis for cervical cancer radiation therapy, thereby contributing to the overall improvement in the quality of life for patients undergoing such treatment. This article is presented in accordance with the MDAR reporting checklist (available at https://tcr.amegroups.com/article/view/10.21037/tcr-22-2792/rc).
Methods
Patient cohort
The present study compared the dose parameters of target areas and OARs in IMRT planning with and without MCO. The outcome measures included dose parameters of target areas and OARs, which belonged to a comparison of means between two sample groups. Based on previous research, comparative studies of planning typically require a minimum of 20 study subjects. The study recruited 25 patients with cervical cancer who had previously received radiotherapy using the IMRT technique between January 2021 and May 2022. The patient inclusion criteria were as follows: (I) histopathological confirmation of cervical cancer, (II) underwent IMRT radiation therapy, and (III) a prescribed dose of 180 cGy/28 fractions for the patient. The study was conducted in accordance with the Declaration of Helsinki (as revised in 2013). This study was approved by the Ethics Committee of Shandong First Medical University Affiliated Cancer Hospital (approval ID: SDTHEC2020008005) and patient informed consent for this retrospective study was waived. During the simulated positioning, all patients underwent computed tomography (CT) in the prone position (CT, Siemens Healthcare, Forchheim, Germany) with a pelvic holder for fixation. It should be noted that before the CT scan, the patient took 300 mL of water to fill the bladder volume.
Target definitions
Experienced physician delineated the clinical target volume (CTV) of cervical cancer based on CT images, which followed the radiation therapy oncology group (RTOG) outline guidelines (17-19). The CTV included the primary cervical tumor site as well as the metastatic lymph node area. The planning target volume (PTV) was created from the CTV and extended outward by 5 mm. To ensure accuracy and precision, a senior physician reviewed the delineation process. OARs including the rectum, bladder, femur-head, and small bowel were also depicted.
Treatment planning
The steps of this study are shown in Figure 1. IMRT plans without MCO (W-IMRT) were generated using a commercial treatment planning system (Eclipse, version 15.6, Varian Medical System, Palo Alto, CA, USA). The beam fields were divided into 9 fields equally. All W-IMRT plans were calculated by anisotropic analytic algorithm (AAA). The prescribed dose of 50.4 Gy (180 cGy/28 fraction) was delivered to the PTV. All plans met that at least 95% of the PTV would receive the prescribed dose, while the maximum dose would not exceed 110% of the prescribed dose. The dose constraints for OARs were as follows: bladder and rectum, mean dose less than 4,000 cGy and V40 <40% (the volume of the bladder or rectum covered by 40 Gy was less than 40%), bilateral femur-head mean dose less than 2,500 cGy and V40 <5% (the volume of the femur-head covered by 40 Gy is less than 5%). All plans were completed by experienced dosimetrists and reviewed and approved by senior physicists.
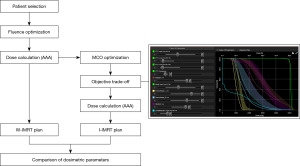
The individualizing IMRT (I-IMRT) plans with MCO were generated in the MCO program of Eclipse version 15.6, which offers tools for real-time exploration and visual evaluation of the range of trade-offs in target coverage and healthy tissue sparing for IMRT plans. The I-IMRT plan was developed by experienced dosimetrist based on the MCO “trade-off” to achieve both optimal PTV coverage and sufficiently OAR dose sparing while reducing the small bowel D2cc index to 5,200 cGy.
Dosimetric evaluation
Dose differences between the W-IMRT plans and the I-IMRT were analyzed based on multiple dosimetry parameters. For PTV, maximum dose (Dmax), minimum dose (Dmin), mean dose (Dmean), minimum dose in 2% of the PTV indicating the maximum dose (D2%) and minimum dose in 98% of the PTV indicating the minimum dose (D98%) were analyzed. Conformity index (CI) and homogeneity index (HI) were calculated for PTV according to the following equations (20):
where D5, D95 were the doses to 5% & 95% volume of the PTV
where VT denoted the volume of the PTV, VP represents the area covered by the prescribed dose, and VTP denoted the PTV area covered by the prescribed dose.
In addition to the D2cc index for the small bowel, the indicators of V40 and Dmean were also analyzed for rectum, bladder and bilateral femur-head.
Statistical analysis
Statistical analyses were performed on all data using Statistical Package for Social Sciences v20.0 software (SPSS Inc., Chicago, IL, USA). Differences in dose were analyzed using the Wilcoxon-signed rank tests for different plans. Differences were considered statistically significant when P<0.05 (2-tailed).
Results
A total of 25 cervical cancer patients who received IMRT radiotherapy were included in this study. The average age of the patients in this cohort was 57 years (range, 27–68 years; Table 1). The median volume of the PTV, rectum, and bladder were 901.5±180.27, 73.2±46.06, and 278.7±181.75 cm3, respectively (Table 1). The dose distribution of the two plans for representative cases is shown in Figure 2, and the corresponding dose-volume histogram (DVH) is shown in Figure 3.
Table 1
Variable | Value |
---|---|
Age, years | |
Median | 57 |
Range | 27–68 |
PTV volume, cm3 | |
Mean ± SD | 901.5±180.27 |
Range | 501.3–1,098.5 |
Rectum volume, cm3 | |
Mean ± SD | 73.2±46.06 |
Range | 19.9–220.3 |
Bladder volume, cm3 | |
Mean ±SD | 278.7±181.75 |
Range | 210.4–857.7 |
PTV, planning target volume; SD, standard deviation.
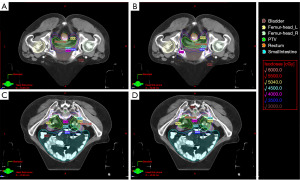
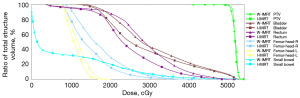
Target
The dosimetric parameters difference of PTV are summarized in Table 2. When compared to the W-IMRT plan, the I-IMRT plan resulted in significant improvement in the PTV. In the PTV, the I-IMRT plan generated a better HI from 1.05 to 1.03 in comparison to the W-IMRT plan, respectively. On the other hand, the CI for the I-IMRT plan was decreased from 0.91 to 0.87, which was slightly worse than the W-IMRT plan. With regard to the Dmax, the I-IMRT plan showed more increment than W-IMRT. The Dmax for I-IMRT plan increased from 5,398.5 to 5,470.0 cGy in comparison to the W-IMRT. Nevertheless, Dmax was less than 110% of the prescribed dose, which met the criteria for clinical treatment. The Dmean, Dmin, D98% and D2% of the I-IMRT plan did not show a statistically significant difference when compared with the W-IMRT plan (P=0.635, P=0.599, P=0.233, P=0.554).
Table 2
Dosimetry parameter | W-IMRT | I-IMRT | P value | |||
---|---|---|---|---|---|---|
Mean | SD | Mean | SD | |||
Dmax (cGy) | 5,398.5 | 48.75 | 5,470.0 | 54.64 | <0.001 | |
Dmean (cGy) | 5,206.6 | 44.79 | 5,213.2 | 41.38 | 0.635 | |
Dmin (cGy) | 4,074.4 | 133.82 | 4,110.5 | 246.27 | 0.599 | |
D98% (cGy) | 5,047.9 | 44.42 | 5,068.0 | 40.86 | 0.233 | |
D2% (cGy) | 5,325.5 | 48.07 | 5,332.1 | 43.03 | 0.554 | |
HI | 1.05 | 0.002 | 1.03 | 0.197 | <0.001 | |
CI | 0.91 | 0.018 | 0.87 | 0.015 | <0.001 |
PTV, planning target volume; W-IMRT, IMRT plans without MCO; I-IMRT, individualizing IMRT; IMRT, intensity-modulated radiation therapy; SD, standard deviation; D98%, minimum dose in 98% of the PTV indicating the minimum dose; D2%, minimum dose in 2% of the PTV indicating the maximum dose; HI, homogeneity index; CI, conformity index.
OARs
The dosimetry difference statistics for OARs are shown in Table 3. Figure 4 shows a violin plot of the average amount of these normal organs. In the dosimetry parameters of the bladder, compared with the W-IMRT plan, the Dmean dose of the I-IMRT plan was significantly reduced by 144.8 cGy, from 2,997.3 to 2,852.5 cGy, and the V40 was reduced by 1.45%, respectfully (Table 3, Figure 4A). For the rectum doses, compared with the W-IMRT plan, the Dmean dose of the I-IMRT plan was reduced by 43.9 cGy, from 2,938.1 to 2,894.2 cGy, and the V40 was significantly reduced by 2.7% (Table 3, Figure 4B). In bilateral femur heads, the Dmean of the I-IMRT plan decreased by more than 150 cGy from the values of W-IMRT plan (L: 1,602.2 to 1,450.3; R: 1,722.5 to 1,530.2), respectively (Table 3, Figure 4C,4D). On the other hand, The V40 of the I-IMRT plan did not show a statistically significant difference when compared with the W-IMRT plan (P=0.063, P=0.542).
Table 3
OARs | Dosimetry parameter | W-IMRT | I-IMRT | P value | |||
---|---|---|---|---|---|---|---|
Mean | SD | Mean | SD | ||||
Bladder | Dmean (cGy) | 2,997.3 | 305.11 | 2,852.5 | 428.07 | <0.001 | |
V40 (%) | 19.36 | 8.096 | 17.91 | 7.871 | <0.001 | ||
Rectum | Dmean (cGy) | 2,938.1 | 275.14 | 2,894.2 | 328.94 | <0.001 | |
V40 (%) | 19.3 | 9.41 | 16.6 | 8.92 | <0.001 | ||
Femur-head-L | Dmean (cGy) | 1,602.2 | 451.95 | 1,450.3 | 422.22 | <0.001 | |
V40 (%) | 0.12 | 0.32 | 0.02 | 0.09 | 0.063 | ||
Femur-head-R | Dmean (cGy) | 1,722.5 | 208.79 | 1,530.2 | 248.08 | <0.001 | |
V40 (%) | 0.11 | 0.31 | 0.08 | 0.23 | 0.542 | ||
Small bowel | D2cc (cGy) | 5,282.3 | 33.17 | 5,186.7 | 18.58 | <0.001 |
OARs, organs at risk; W-IMRT, IMRT plans without MCO; I-IMRT, individualizing IMRT; SD, standard deviation; IMRT, intensity-modulated radiation therapy; V40, the volume of the femur-head covered by 40 Gy; Dmean, mean dose; D2cc, the minimal dose to the 2 cm3 of the small bowel receiving the maximal dose.
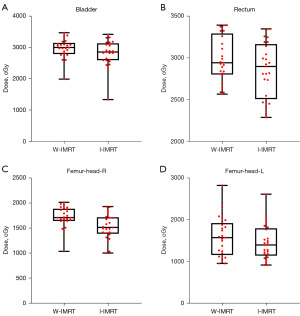
For D2cc of the small bowel, a high dose indicator that we focused on, the I-IMRT plan was significantly reduced by 95.6 cGy, from 5,282.3 to 5,186.7 cGy. In particular, the D2cc for all I-IMRT plans had been reduced to 5,200 cGy. Furthermore, we analyzed the overlapping area of the small bowel and PTV (S&P, Figure 5). The Dmean of S&P decreased, from 5,205.6 to 5,130.7 cGy. Also, the Dmean of S&P for all I-IMRT plans had greater than 5,040 cGy, respectively.
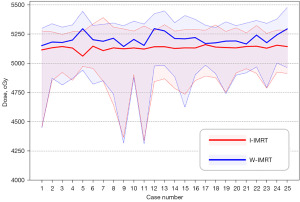
Discussion
Our study investigated dosimetry parameters differences between W-IMRT plan and I-IMRT plan—for 25 patients with cervical cancer. To our knowledge, we are the first to use the Eclipse MCO program to participate in the IMRT program of cervical cancer radiotherapy. In particular, we investigated dose changes in other normal tissues and organs when using the MCO approach to lower the high-dose index of the small bowel. In the trade-off of I-IMRT plan, particular emphasis was placed on maximizing PTV coverage and sparing of other OARs while lowering the high dose parameters (D2cc & Dmean of S&P) of the small bowel. The results showed a significant superiority of I-IMRT in dosimetry. With the same dose constraints, I-IMRT plan provided better OAR sparing through a trade-off, both in the rectum, bladder and bilateral femur-head (Table 2; Figure 4), while the high dose parameter of small bowel achieved a sufficiently optimistic level. In PTV, the better HI indices improved despite an increase in Dmax, and a slight deterioration in the CI index.
In external radiation therapy for cervical cancer, the potential effects of radiation on the bladder, rectum, bilateral femoral heads and other OARs are routinely considered. It has been reported that radiotherapy-related urogenital toxicity, often manifested as dysuria, rectal pain, or bleeding, significantly reduces patients’ quality of life (21-23). The incidence of acute genitourinary toxicity and late genitourinary toxicity was 31.9% and 28.0%, respectively. In recent years, research has gradually paid attention to the toxicity analysis of the small bowel. As reported in the National Comprehensive Cancer Network (NCCN) guidelines, the dose-volume effect relationship for predicting advanced small bowel morbidity suggests that the small bowel D2cc threshold should be maintained at 5,200 cGy when delivering 5,040 cGy to patients (24). Under computer optimization, IMRT for cervical cancer can obtain a high dose conformity and a steeper dose gradient, and its dose conformity can ensure that the tumor area receives a high dose of radiation, while the OARs receive less radiation dose, so it will reduce the incidence of acute and chronic radiation injury. Importantly, it is a critical step in determining the quality of an IMRT plan, in which seeking a balance between optimizing target coverage and preserving OARs. However, the D2cc index is an unclear optimization target compared to the maximum dose, which would consume a significant amount of time and effort until an acceptable and optimal clinical delivery plan can be created.
MCO is a useful tool for obtaining a satisfactory IMRT plan (25-28). It helps the treatment planning process be more efficient by allowing plan designers to explore multiple dose-target trade-offs for a given patient, including between target coverage and OARs. In recent years, MCO has been extensively studied and has been integrated into radiation therapy planning systems, which simplifies the complexity of MCO methods and avoids the disadvantage of requiring additional computational resources. Currently, the MCO algorithm is mainly implemented in two commercial treatment planning systems, RayStation (RaySearch Laboratories AB, Stockholm, Sweden) and Eclipse (Varian Medical System, Palo Alto, CA, USA) (29).
Existing researches have mainly focused on the MCO program of the RayStation treatment planning system (TPS), which was developed earlier. Craft et al. first provided concrete evidence for the superiority of the MCO program (30). In their study, the RayStation MCO-based IMRT plan was superior to the conventional trial-and-error-based IMRT plan in planning efficiency and dose distribution quality in all cases. Subsequent studies have confirmed that RayStation-based MCO planning is a promising and effective radiotherapy planning optimization technique. In the study of McGarry et al., the RayStation MCO-based plan showed equivalent or better target homogeneity with significantly lower rectal dose (490±280 cGy) (31). Similar results were also demonstrated by Guerrero et al. using the latest version of RayStation (version 6) (32). However, another widely used treatment planning system, Eclipse, was relatively late to offer an MCO option, and to our knowledge there is only one study evaluating the effectiveness of using MCO in Eclipse treatment planning. In the study by Park et al. in prostate cancer patients, the MCO program achieved a steeper dose drop across overlapping regions, resulting in lower dose parameters. There were significant reductions in intravesical doses (241 vs. 254 cGy; (P<0.001) and rectum (474 vs. 604 cGy, P<0.001) (29). Compared to the report in the Park et al. study, our results suggest that the sparing of the rectum and bladder is comparable. However, in our study, the mean dose to the bilateral femur heads was also reduced, not increased. This difference may be due not only to physician preferences weighing conflicting priorities, but also to the added indicator of small bowel D2cc in our study.
In conclusion, comparing to the conventional single-objective optimization, the MCO method has the flexibility, which can help dosimetrists and radiologists to complete the plan design process more efficiently without constant trial and error. Moreover, the MCO plan can better retain OARs. Of course, the application of the MCO method in the design of radiotherapy plans for other types of tumors remains to be further studied. At the same time, the application of MCO in new radiotherapy technologies such as protons and heavy ions should also attract our attention.
In this study, an analysis of dose differences between I-IMRT and W-IMRT reveals that the application of the MCO method in I-IMRT planning offers superior protection to adjacent OARs. This improvement is achieved while maintaining comparable PTV coverage. Furthermore, the MCO method effectively reduces the high-dose exposure to the small intestine in patients with cervical cancer. Notably, the MCO approach streamlines the planning process by eliminating time-consuming iterative calculations. Thus, it provides a swift and valuable tool for aiding physicians and dosimetrists in the creation of more advantageous IMRT treatment plans. However, this study also has some limitations. Firstly, the patient data for the study were collected retrospectively, with a lack of follow-up for overall survival. This implies a lack of clinical evidence for the association of lowering the small intestinal high dose index with translation into a significant reduction in toxicity in actual clinical practice. In the future, we will collect more case and prognostic information to obtain the correlation between dosimetric parameters and the toxicity of OARs. Secondly, as this is a retrospective study, planning efficiency metrics such as planning time were not evaluated in our study. In the future, we plan to collaborate more closely with clinicians to proactively gather parameters that capture planning efficiency and other relevant metrics. Finally, considering that the precision radiotherapy such as IMRT has inter-fraction and intra-fraction setup errors, respiratory motion, and uncertainties in bladder, rectal, and small bowel positions and filling degrees, the MCO method would be combined with adaptive radiotherapy to achieve more precise tumor treatment and minimize radiation damage to surrounding tissues in the future.
Conclusions
Dosimetric differences suggest that the I-IMRT plan using the MCO method provides better protection of other OARs and equivalently in PTV coverage, while lowering the high-dose index in the small bowel as much as possible for patients with cervical cancer, although its clinical utility requires further prospective studies to demonstrate.
Acknowledgments
Funding: This study was supported by
Footnote
Reporting Checklist: The authors have completed the MDAR reporting checklist. Available at https://tcr.amegroups.com/article/view/10.21037/tcr-22-2792/rc
Data Sharing Statement: Available at https://tcr.amegroups.com/article/view/10.21037/tcr-22-2792/dss
Peer Review File: Available at https://tcr.amegroups.com/article/view/10.21037/tcr-22-2792/prf
Conflicts of Interest: All authors have completed the ICMJE uniform disclosure form (available at https://tcr.amegroups.com/article/view/10.21037/tcr-22-2792/coif). The authors have no conflicts of interest to declare.
Ethical Statement:
Open Access Statement: This is an Open Access article distributed in accordance with the Creative Commons Attribution-NonCommercial-NoDerivs 4.0 International License (CC BY-NC-ND 4.0), which permits the non-commercial replication and distribution of the article with the strict proviso that no changes or edits are made and the original work is properly cited (including links to both the formal publication through the relevant DOI and the license). See: https://creativecommons.org/licenses/by-nc-nd/4.0/.
References
- Malikova H, Burghardtova M, Fejfarova K, et al. Advanced cervical cancer in young women: imaging study of late and very late radiation-related side effects after successful treatment by combined radiotherapy. Quant Imaging Med Surg 2021;11:21-31. [Crossref] [PubMed]
- Chino J, Annunziata CM, Beriwal S, et al. Radiation Therapy for Cervical Cancer: Executive Summary of an ASTRO Clinical Practice Guideline. Pract Radiat Oncol 2020;10:220-34. [Crossref] [PubMed]
- Wu Y, Chong Y, Han C, et al. Second primary malignancies associated with radiation therapy in cervical cancer patients diagnosed between 1975 and 2011: a population-based competing-risk study. Ann Transl Med 2021;9:1375. [Crossref] [PubMed]
- Fu Z, Wang C, Chen J, et al. The incidence and risk factors of acute radiation-induced dermatitis in gynecologic malignancies treated with intensity-modulated radiation therapy. Transl Cancer Res 2020;9:6062-9. [Crossref] [PubMed]
- Podder TK, Fredman ET, Ellis RJ. Advances in Radiotherapy for Prostate Cancer Treatment. Adv Exp Med Biol 2018;1096:31-47. [Crossref] [PubMed]
- Wang Y, Zhang L, Liu W, et al. Apatinib enhances the antitumor effects of radiation in HeLa cell line mouse model of invasive cervical cancer. Ann Transl Med 2022;10:459. [Crossref] [PubMed]
- Wang Z, Ren X, Liu Z, et al. Multimodality treatment for multiple recurrences of cervical cancer after radiotherapy: a case report. Transl Cancer Res 2022;11:943-51. [Crossref] [PubMed]
- Mazeron R, Fokdal LU, Kirchheiner K, et al. Dose-volume effect relationships for late rectal morbidity in patients treated with chemoradiation and MRI-guided adaptive brachytherapy for locally advanced cervical cancer: Results from the prospective multicenter EMBRACE study. Radiother Oncol 2016;120:412-9. [Crossref] [PubMed]
- Hwang CL, Masud ASM. Multiple objective decision making—methods and applications: a state-of-the-art survey. Springer Science & Business Media, 2012.
- Miettinen K. Nonlinear multiobjective optimization. Springer Science & Business Media, 2012.
- Monz M, Küfer KH, Bortfeld TR, et al. Pareto navigation: algorithmic foundation of interactive multi-criteria IMRT planning. Phys Med Biol 2008;53:985-98. [Crossref] [PubMed]
- Kurz C, Süss P, Arnsmeyer C, et al. Dose-guided patient positioning in proton radiotherapy using multicriteria-optimization. Z Med Phys 2019;29:216-28. [Crossref] [PubMed]
- Spalding M, Walsh A, Aland T. Evaluation of a new GPU-enabled VMAT multi-criteria optimisation plan generation algorithm. Med Dosim 2020;45:368-73. [Crossref] [PubMed]
- Harrer C, Ullrich W, Wilkens JJ. Prediction of multi-criteria optimization (MCO) parameter efficiency in volumetric modulated arc therapy (VMAT) treatment planning using machine learning (ML). Phys Med 2021;81:102-13. [Crossref] [PubMed]
- Kamal-Sayed H, Ma J, Tseung H, et al. Adaptive method for multicriteria optimization of intensity-modulated proton therapy. Med Phys 2018;45:5643-52. [Crossref] [PubMed]
- Spalding M, Walsh A, Clarke H, et al. Evaluation of a new hybrid VMAT-IMRT multi-criteria optimization plan generation algorithm. Med Dosim 2020;45:41-5. [Crossref] [PubMed]
- Small W Jr, Mell LK, Anderson P, et al. Consensus guidelines for delineation of clinical target volume for intensity-modulated pelvic radiotherapy in postoperative treatment of endometrial and cervical cancer. Int J Radiat Oncol Biol Phys 2008;71:428-34. [Crossref] [PubMed]
- Lim K, Small W Jr, Portelance L, et al. Consensus guidelines for delineation of clinical target volume for intensity-modulated pelvic radiotherapy for the definitive treatment of cervix cancer. Int J Radiat Oncol Biol Phys 2011;79:348-55. [Crossref] [PubMed]
- Small W Jr, Bosch WR, Harkenrider MM, et al. NRG Oncology/RTOG Consensus Guidelines for Delineation of Clinical Target Volume for Intensity Modulated Pelvic Radiation Therapy in Postoperative Treatment of Endometrial and Cervical Cancer: An Update. Int J Radiat Oncol Biol Phys 2021;109:413-24. [Crossref] [PubMed]
- Yoon M, Park SY, Shin D, et al. A new homogeneity index based on statistical analysis of the dose-volume histogram. J Appl Clin Med Phys 2007;8:9-17. [Crossref] [PubMed]
- Lin AJ, Kidd E, Dehdashti F, et al. Intensity Modulated Radiation Therapy and Image-Guided Adapted Brachytherapy for Cervix Cancer. Int J Radiat Oncol Biol Phys 2019;103:1088-97. [Crossref] [PubMed]
- Naik A, Gurjar OP, Gupta KL, et al. Comparison of dosimetric parameters and acute toxicity of intensity-modulated and three-dimensional radiotherapy in patients with cervix carcinoma: A randomized prospective study. Cancer Radiother 2016;20:370-6. [Crossref] [PubMed]
- Vargo JA, Kim H, Choi S, et al. Extended field intensity modulated radiation therapy with concomitant boost for lymph node-positive cervical cancer: analysis of regional control and recurrence patterns in the positron emission tomography/computed tomography era. Int J Radiat Oncol Biol Phys 2014;90:1091-8. [Crossref] [PubMed]
- Abu-Rustum NR, Yashar CM, Bean S, et al. NCCN Guidelines Insights: Cervical Cancer, Version 1.2020. J Natl Compr Canc Netw 2020;18:660-6. [Crossref] [PubMed]
- Nguyen D, McBeth R, Sadeghnejad Barkousaraie A, et al. Incorporating human and learned domain knowledge into training deep neural networks: A differentiable dose-volume histogram and adversarial inspired framework for generating Pareto optimal dose distributions in radiation therapy. Med Phys 2020;47:837-49. [Crossref] [PubMed]
- Wang J, Jin X, Zhao K, et al. Patient feature based dosimetric Pareto front prediction in esophageal cancer radiotherapy. Med Phys 2015;42:1005-11. [Crossref] [PubMed]
- Teichert K, Süss P, Serna JI, et al. Comparative analysis of Pareto surfaces in multi-criteria IMRT planning. Phys Med Biol 2011;56:3669-84. [Crossref] [PubMed]
- Zitzler E, Thiele L, Laumanns M, et al. Performance assessment of multiobjective optimizers: An analysis and review. IEEE Transactions on Evolutionary Computation 2003;7:117-32. [Crossref]
- Park J, Park J, Oh S, et al. Multi-criteria optimization for planning volumetric-modulated arc therapy for prostate cancer. PLoS One 2021;16:e0257216. [Crossref] [PubMed]
- Craft DL, Hong TS, Shih HA, et al. Improved planning time and plan quality through multicriteria optimization for intensity-modulated radiotherapy. Int J Radiat Oncol Biol Phys 2012;82:e83-90. [Crossref] [PubMed]
- McGarry CK, Bokrantz R, O'Sullivan JM, et al. Advantages and limitations of navigation-based multicriteria optimization (MCO) for localized prostate cancer IMRT planning. Med Dosim 2014;39:205-11. [Crossref] [PubMed]
- Guerrero M, Fellows Z, Mohindra P, et al. Multicriteria optimization: Site-specific class solutions for VMAT plans. Med Dosim 2020;45:7-13. [Crossref] [PubMed]