Sinensetin suppresses breast cancer cell progression via Wnt/β-catenin pathway inhibition
Highlight box
Key findings
• Sinensetin inhibits breast cancer cell survival, metastasis, and epithelial-mesenchymal transition via inhibiting Wnt/β-catenin pathway.
What is known and what is new?
• Researches on the antitumor activity of polymethoxyflavones (PMFs) have mostly focused on nobiletin. 5,6,7,8,3,4'-hexacycloethoxyflavones damage several cancer cell activities in vitro and in vivo.
• This study investigated whether sinensetin suppressed the breast cancer cell progression via inhibition of Wnt/β-catenin pathway.
What is the implication, and what should change now?
• Importantly, sinensetin inhibited the viability of breast cancer cells and promoted their apoptosis through inhibiting the Wnt/β-catenin signaling pathway.
Introduction
Compounds obtained from natural products have clinical potential as therapeutic agents. Over the past 60 years, almost 70% of the novel anti-cancer drugs derived from natural products or based on data obtained from natural products were approved (1). A large number of natural product drugs have been approved, including paclitaxel, homoharringtonine, ingenol mebutate and so on (2). With the application of traditional biological activity-guided separation strategies and advanced analytical techniques in the discovery of drugs from natural products, the discovery capabilities of modern drugs derived from natural products have been enhanced (2). These preliminary foundations and advances provide broad prospects for the application of natural products in tumors.
In recent years, a new research direction emerged in the study of flavonoids as anticancer drugs after the approval of alvocidib by the Food and Drug Administration (FDA) for the treatment of acute myeloid leukemia in 2014 (3-6). Flavonoids are widely present in the plant kingdom, and epidemiological studies have shown that dietary flavonoids have a chemopreventive effect on cancer (7-9). Polymethoxyflavones (PMFs) are flavonoids that are replaced by two or more methoxy groups. Studies of diverse medicinal plants and citrus have shown a high structural variability of PMF, such as the smaller methoxyflavones and structural isomers. The structural isomers include 5,6,7,4'-tetramethoxyflavone, tangeretin, 3,5,6,7,3',4'-hexamethoxyflavone, nobiletin, 3,5,6,7,8,3',4'-heptamethoxyflavone, and sinensetin. While several studies indicate that composition and structure affected profoundly the antitumor capacity of flavonoids, few studies have been published on the role of these little-known compounds (7). 5,6,7,4'-tetramethoxyflavone represses the growth of triple-negative breast cancer (TNBC) by inhibiting the MAPK and Akt signaling pathways and causing cell cycle arrest (10). Nobiletin also inhibits TNBC (11). Targretin inhibits human breast carcinoma cells via a variety of mechanism (12). 3,5,6,7,3',4'-hexamethoxyflavone and 3,5,6,7,8,3',4'-heptamethoxyflavone also play an important role in breast cancer (10). Sinensetin showed strong sensitization in HepG2 cells, which caused the cell viability curve to decrease sharply (13-15). The current study suggests that if more toxicological and in vivo studies are conducted, sinensetin have the potential to become a candidate for the treatment of gallbladder adenocarcinoma (16). Sinensetin also targets cancer stem cells (CSCs) and enhances the anti-proliferative effect of 5-fluorouracil in a 3D cell model of colorectal cancer (17). A recent study reported that sinensetin ability to resist proliferation was enhanced by CYP1 family enzymes. These flavonoids are superior to polyhydroxylated flavonoids because they possess high metabolic stability and oral bioavailability, which increases their chemopreventive activity in cancer (18). However, the role and underlying mechanism of sinensetin in breast cancer remain unknown.
Breast cancer is one of the most common tumors in the world. The pathogenesis of breast cancer is very complicated. Many signal transduction pathways are involved (19). Cyclin-dependent kinases (CDKs) promote breast cancer cell growth by regulating breast cancer cell cycle. In breast cancer, CDKs are overactive or CDK inhibitory proteins are dysfunctional (20). In addition, Notch signaling, SHH signaling, BRK pathway, HER signaling and others pathways are reported to be involved in the occurrence and development of breast cancer (21). Wnt signaling has been shown to be active in several tumors, including breast cancer (22). The canonical Wnt protein activates transcription of β-catenin/T-cell factor (TCF)/lymphatic enhancing factor (LEF) target genes by transducing signaling β-catenin (23,24). Some researchers have demonstrated that Wnt antagonists with frequent genetic and epigenetic mutations in breast cancer led to β-catenin-mediated activation of Wnt target genes, suggesting that the Wnt-catenin-β1 (CTNNB1) signaling pathway plays an important role in the oncogenic process (25,26). Similarly, it has been reported in breast cancer that several cytosolic and nucleus components of the Wnt/β-catenin pathway is deregulated, such as DKK3, Dickkopf (DKK)-1, APC, Wnt inhibitor factor 1 (WIF1), SFRP5, MCC, glycogen synthase kinase-3β (GSK3B), and CTNNBIP1 (27-29). Activation of the Wnt/β-catenin signaling pathway is required for epithelial-mesenchymal transition (EMT) to regulate breast cancer drug resistance (30). The Wnt signaling pathway is an important molecular node that maintains CSC properties and promotes epithelial-mesenchymal transformation cell programming (31). Therefore, key regulators targeting the Wnt pathway can be used as effective targets for breast cancer chemotherapy and as effective biomarkers for the prognosis of breast cancer patients.
In this study, we aimed to explore the potential anticancer role of sinensetin in breast cancer cells. We found that sinensetin inhibited the viability of breast cancer cells and promoted their apoptosis through inhibiting the Wnt/β-catenin signaling pathway. Our study was the first to demonstrate that sinensetin suppressed the malignant progression of breast cancer via inhibition of Wnt/β-catenin signaling. We present this article in accordance with the MDAR reporting checklist (available at https://tcr.amegroups.com/article/view/10.21037/tcr-23-1317/rc).
Methods
Cell culture
The MCF-10A (cat. no. CRL-10317, Manassas, Virginia, USA), MCF7 (cat. no. CL-0149, Manassas) and MDA-MB-231 (cat. no. CL-0150B, Manassas) cell lines were obtained from the American Type Culture Collection through commercial channels. MCF7 cells were cultured in DMEM (PM150210, Procell, Wuhan, China) containing 10% fetal bovine serum (FBS) (Procell), penicillin (50 U/mL, 10378016, Gibco, Waltham, MA, USA), and streptomycin (50 µg/mL, 10378016, Gibco) in 5% CO2. MDA-MB-231 cells were cultured in F15 medium (PM150110, Procell) containing 15% FBS, penicillin (50 U/mL), and streptomycin (50 µg/mL) in 5% CO2. MCF7 and MDA-MB-231 cells were cultured in sinensetin (HY-N0297, MedChemExpress, New Jersey, USA) at different concentrations (0, 30, 60, 120, 180, and 240 µM) for 24 h. Cells were treated with SKL2001 (SKL101085, MedChemExpress) for one hour before the exposure of sinensetin according to the previous study (32). The concentration of 40 µM SKL2001 was in accordance with the previous study (33).
Cell viability assay
Cell viability was analyzed according to the manufacturer’s protocol using the Cell Counting Kit-8 (CCK-8) assay (C0037, Beyotime Biotechnology, Shanghai, China). Cells were seeded at a density of 5×103 cells/well in 96-well plates for the CCK-8 assay. After sinensetin treatment for 24 h, cells were incubated with 10 µL CCK-8 solution for 1 h at 5% CO2 and 37 ℃ incubator. Data from the optical density (OD) at 450 nm were measured by a microplate reader (BioTek, Winooski, VT, USA). The calculating methodology was as follow: Cell viability (%) = (measured value -blank value)/(control value − blank value) × 100%. MCF7 and MDA-MB-231 cells treated with 0 µM sinensetin acted as control. MCF-10A cells treated with 120 µM sinensetin for 0 h also served as control. CCK-8 results were obtained from more than three replicate experiments performed by two operators under the same laboratory conditions. The same reagents and instrument systems were used for all experiments. All methods have mentioned the control in each experiment.
Western blotting
MCF-10A, MCF7 and MDA-MB-231 cells were lysed with radio immunoprecipitation assay (RIPA) (P0013B, Beyotime Biotechnology) containing 50 mM Tris (pH 7.4), 150 mM NaCl, 1% Triton X-100, 1% sodium deoxycholate, 0.1% sodium dodecyl sulfate (SDS), sodium orthovanadate, sodium fluoride, ethylene diamine tetraacetic acid (EDTA), leupeptin, phenylmethanesulfonyl fluoride (PMSF) and so on. After full lysis, the cells were centrifuged at 10,000–14,000 g for 3–5 min and the supernatant was collected. Cells without any substance acted as control. Total proteins were extracted from MCF-10A, MCF7 and MDA-MB-231 cells treated as described above. Aliquots containing 30 µg of proteins were electrophoresed on 8–12% sodium dodecyl sulfate-polyacrylamide gel electrophoresis (SDS-PAGE) gels and transferred to polyvinylidene fluoride (PVDF) membranes (1620256, Bio-Rad Laboratories, Hercules, CA, USA). Membranes were blocked with 5% non-fat milk for 2 h, and then incubated with primary antibodies against caspase 9 (9509, CST, Danvers, MA, USA), caspase 3 (9662, CST), Bax (50599-2-Ig, Proteintech, Chicago, USA), E-cadherin (20874-1-AP, Proteintech), Bcl-2 (26593-1-AP, Proteintech), Slug (9585, CST), Snail (13099-1-AP, Proteintech), Vimentin (10366-1-AP, Proteintech), Twist (25465-1-AP, Proteintech), Zeb1 (21544-1-AP, Proteintech), Zeb2 (14026-1-AP, Proteintech), E47 (12258, CST), β-catenin (8480, CST, USA), LEF1 (14972-1-AP, Proteintech), TCF1/TCF7 (14464-1-AP, Proteintech) and β-actin (81115-1-RR, Proteintech). Membranes were then incubated in secondary horseradish peroxidase (HRP)-conjugated goat anti-mouse or goat anti-rabbit IgG antibody (A0216 and A0208, Beyotime Biotechnology). Protein expressions from Western blotting images were quantitatively analyzed by image J (Version 1.52v). ChemiDocTM Touch Imaging System (Bio-Rad) was used to image and chemiluminescence detection (34080; Thermo Fisher Scientific, Waltham, MA, USA and 1705061; Bio-Rad). Western blotting results were obtained from more than three replicate experiments performed by two operators under the same laboratory conditions. The same reagents and instrument systems were used for all experiments. All methods have mentioned the control in each experiment.
Quantitative real-time polymerase chain reaction (qRT-PCR)
Total RNA was extracted according to the manufacturer’s instructions using Trizol (R0016, Beyotime Biotechnology). Trizol volume was added to the cells at a ratio of 10 cm2/mL. They were allowed to dissociate fully at room temperature for 5 min. After collecting the lysate, the precipitate was discarded by centrifugation at 12,000 rpm for 5 min. Chloroform was added at 200 µL (chloroform)/mL (Trizol), mixed with shaking and left for 15 min at room temperature. The cells were centrifuged at 4 ℃ and 12,000 g for 15 min. Drain the upper aqueous phase into another centrifuge tube. Isopropanol was added and mixed, and the mixture was placed at room temperature for 10 min. We centrifuged the mixture at 12,000 g for 10 min at 4 ℃, and the supernatant was discarded; 75% ethanol was added at 1 mL of 75% (ethanol)/mL (Trizol), the centrifuge tube was gently shaken, and the precipitate was suspended. The samples were centrifuged at 4 ℃ 8,000 g for 5 min, and the supernatant was discarded as much as possible. Allow to dry at room temperature for 5–10 min. RNA samples were dissolved using pure water. The final measurement quantified RNA concentration. RNA was converted into cDNA using the one-step qRT-PCR SYBRGreen Kit (DBI, Ludwigshafen, Germany) and the ABI 7500 Fast Real-Time PCR System (Life Tech, Carlsbad, CA, USA). Cells without any substance acted as control. Primers are listed in Table 1. The 2−∆∆Ct method was used to quantify gene expression normalized to GAPDH. RT-PCR results were obtained from more than three replicate experiments performed by two operators under the same laboratory conditions. The same reagents and instrument systems were used for all experiments. All methods have mentioned the control in each experiment.
Table 1
Gene | Forward 5'-3' | Reverse 5'-3' |
---|---|---|
E-cadherin | CTCATGAGTGTCCCCCGGTAT | CAGCCGCTTTCAGATTTTCATC |
Vimentin | GACGCCATCAACACCGAGTT | CTTTGTCGTTGGTTAGCTGGT |
Snail | TCGGAAGCCTAACTACAGCGA | AGATGAGCATTGGCAGCGAG |
Slug | CGAACTGGACACACATACAGTG | CTGAGGATCTCTGGTTGTGGT |
twist | GCCTAGAGTTGCCGACTTATG | TGCGTTTCCTGTTAAGGTAGC |
Zeb1 | TGATCTGGCCATTTTCACCTGT | GACTTGCCAGGACAGCTTGC |
Zeb2 | CACAGGTATGAGTGACTTTGCC | TGGCTGTGTCATGCCATTTC |
E47 | CCACTT CACTG AGTCGC ACAG | GTCTCT CCCGAA GGAGG CATA |
β-catenin | ATGTCCAGCGTTTGGCTGAA | TGGTCCTCGTCATTTAGCAGTT |
LEF-1 | AATGCACGTGAAGCCT | GAATCTGGTTGATAGCTGC |
TCF-1 | TTGATGCTAGGTTCTGGTGTACC | CCTTGGACTCTGCTTGTGTC |
TCF-3 | GTAACATCTGCTCCGCGTTAAT | TCGAGGGAGACCCAACTTCA |
GAPDH | AACAGGAGGTCCCTACTCCC | GCCATTTTGCGGTGGAAATG |
qPCR, quantitative polymerase chain reaction.
Clone formation assay
Approximately 1,000 cells were seeded in 6-well plates and cultured in medium without sinensetin for the first 2 days, then in medium containing 120 µM sinensetin for the experimental group until 2 weeks at 5% CO2 and 37 ℃ incubator. Cells without any substance acted as control. Briefly, cells were fixed in 4% paraformaldehyde (P0099, Beyotime Biotechnology) and then washed three times. Cells were cultured with 0.1% crystal violet (C0121, Beyotime Biotechnology) and washed with water. Photographs were taken to assess the relative number of clones. The results of clone formation assay were obtained from more than three replicate experiments performed by two operators under the same laboratory conditions. The same reagents and instrument systems were used for all experiments. All methods have mentioned the control in each experiment.
Transwell invasion assay
The experiment were carried out by 24-well Transwell plates (3422-ND, Corning, NY, USA) Matrigel® (C0372, Beyotime Biotechnology) with a membrane pore size of 8 µm. Approximately 1×105 MCF7 and MDA-MB-231 cells were cultured with serum-free medium in the upper chamber. Medium supplemented with 10% FBS and 120 µM sinensetin in the lower chamber served as the chemo-attractant. Cells above the Matrigel layer were swabbed after incubation for 24 h, and 4% paraformaldehyde were used to fix MCF7 and MDA-MB-231 cells below the membrane. Subsequently, cells were stained with 0.1% crystal violet for 15 min and photographs were taken to assess the relative number of invaded cells for each well. Cells without any substance acted as control. The results of Transwell invasion assay were obtained from more than three replicate experiments performed by two operators under the same laboratory conditions. The same reagents and instrument systems were used for all experiments. All methods have mentioned the control in each experiment.
TUNEL apoptosis assay
Cells were seeded into coverslips and exposed to various treatments. Briefly, cells were fixed with 4% paraformaldehyde, washed with phosphate buffered saline (PBS) (C0221A, Beyotime Biotechnology) twice for 3 min, and then treated with 0.3% Triton X-100 for 10 min. Aliquots of 50 µL TUNEL solution (C1090, Beyotime Biotechnology) were placed in coverslips and cells were cultured for 1 h. Coverslips were washed with tris buffered saline tween (TBST) (ST673, Beyotime Biotechnology) three times. Cells without any substance acted as control. Photographs were taken by microscopy (Olympus BX51). The results of TUNEL were obtained from more than three replicate experiments performed by two operators under the same laboratory conditions. The same reagents and instrument systems were used for all experiments. All methods have mentioned the control in each experiment.
Statistical analysis
Data were presented as the mean ± standard deviation, and statistical analyses were performed using SPSS 20.0 (SPSS, Chicago, IL, USA). The Student’s test was used to calculate the differences between two groups. One-way analysis of variance (ANOVA) was used for comparison between groups, followed by Tukey multiple comparisons. All P values were two-sided, and ***, P<0.001, **, P<0.01, *, P<0.05 was considered to be statistically significant. All experiments were performed in triplicate.
Results
Sinensetin significantly inhibits breast cancer cell viability and promotes apoptosis of breast cancer cell
The CCK-8 assay was performed to investigate the inhibitory impact of sinensetin in MCF7 and MDA-MB-231 cells. As shown in Figure 1A, sinensetin showed a concentration-dependent inhibitory effect on MCF7 and MDA-MB-231 cell viability. The IC50 (half maximal inhibitory concentration) of sinensetin in MCF7 and MDA-MB-231 cells were 131.5 and 97.45 µM, respectively. Therefore, a concentration of 120 µM sinensetin was used to treat MCF7 and MDA-MB-231 cells in subsequent experiments. CCK-8 was used to assess the impact of sinensetin on normal breast cells (MCF-10A) before subsequent experiments. The results showed that 120 µM sinensetin began to suppress the activity of MCF-10A cells after 72 h. This slight toxicity after 72 h might be related to the purity of sinensetin. Importantly, treatment with 120 µM sinensetin for 24 h showed no significant toxicity to normal mammary cells (Figure 1B).
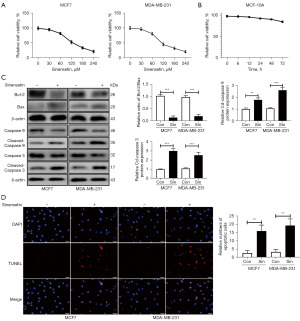
After treatment of MCF7 and MDA-MB-231 cells with 120 µM sinensetin for 24 h, the expression of apoptotic markers was detected by Western blotting. Sinensetin upregulated the protein level of cleaved-caspase 9 and cleaved-caspase 3 in MCF7 and MDA-MB-231 cells. The ratio of Bcl-2/Bax was also decreased after sinensetin treatments. Decreased ratio of Bcl-2/Bax indicated an increase in apoptosis. These results demonstrated that sinensetin increased apoptosis of MCF7 and MDA-MB-231 cells (Figure 1C). The results of the TUNEL assay confirmed that sinensetin increased apoptosis of MCF7 and MDA-MB-231 cells (Figure 1D). These results showed that sinensetin inhibited the viability and increased apoptosis of MCF7 and MDA-MB-231 cells.
Sinensetin decreases metastasis and epithelial-mesenchymal transition (EMT) of MCF7 and MDA-MB-231 cell
To explore the inhibitory effect of sinensetin on metastasis and EMT, Western blotting, Clone formation assay, and Transwell assay were performed. The results of the Clone formation assay showed that sinensetin suppressed obviously the proliferation property, tumor formation and self-renewal of MCF7 and MDA-MB-231 cells (Figure 2A). Moreover, sinensetin inhibited MCF7 and MDA-MB-231 cell invasion as determined by the Transwell assay (Figure 2B). Sinensetin downregulated the protein expression of Vimentin, Slug, Snail, and Twist in MCF7 and MDA-MB-231 cells, while E-cadherin protein expression was upregulated (Figure 2C). Moreover, the results of qRT-PCR showed that sinensetin remarkably decreased the mRNA expression of Vimentin, Slug, Snail, and Twist in MCF7 and MDA-MB-231 cells. E-cadherin mRNA expression was increased after sinensetin treatments (Figure 2D). Other EMT markers including Zeb1, Zeb2, and E47 were also downregulated by sinensetin in MCF7 and MDA-MB-231 cells (Figure 3A,3B). The results of qRT-PCR showed that sinensetin remarkably decreased the mRNA expression of Zeb1, Zeb2, and E47 in MCF7 and MDA-MB-231 cells (Figure 3C). These results indicated that sinensetin suppressed the proliferation, migration, invasion, and EMT of MCF7 and MDA-MB-231 cells.
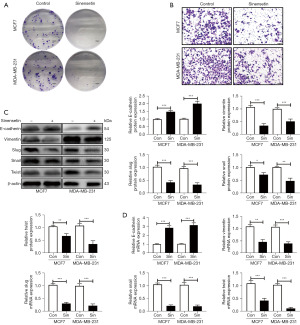
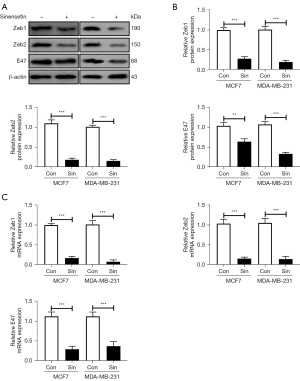
Sinensetin may exert anticancer effects on breast cancer cells through inhibiting the Wnt/β-catenin signaling pathway
The activation of Wnt/β-catenin signaling pathway can promote malignant phenotype of cancer (34,35). The Wnt/β-catenin signaling pathway is involved in regulating the effect of drugs derived from natural products (36,37). To determine whether sinensetin had anticancer effects in breast cancer cell via inhibiting the Wnt/β-catenin signaling pathway, we performed Western blotting to detect the expression of related proteins in MCF7 and MDA-MB-231 cells exposed to sinensetin. Sinensetin downregulated the protein expression of β-catenin, LEF1, TCF1/TCF7, and TCF3/TCF7L1 in MCF7 and MDA-MB-231 cells (Figure 4A,4B). Since the Wnt/β-catenin pathway plays a pivotal role in processes like cell fate determination, proliferation, and migration during mammary gland development and tissue homeostasis in adult tissue (38), alterations in its target genes could affect normal cells. Therefore, the inhibitory effect of sinensetin to Wnt/β-catenin on normal breast cell (MCF-10A) was investigated. There was not no significant difference in the protein expression of β-catenin, LEF1, TCF1/TCF7, and TCF3/TCF7L1 of MCF-10A cells (Figure 4C). Gene expression analysis of markers related to the Wnt/β-catenin pathway by qRT-PCR showed that β-catenin, LEF1, TCF1/TCF7, and TCF3/TCF7L1 mRNA expression were significantly downregulated by sinensetin (Figure 4D). These results indicated that sinensetin suppressed the Wnt/β-catenin signaling pathway in MCF7 and MDA-MB-231 cells.
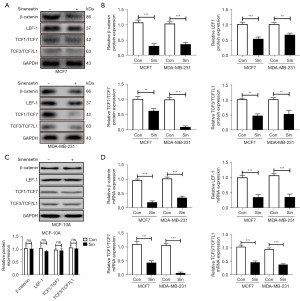
The Wnt agonist SKL2001 reverses the anticancer effect of sinensetin on breast cancer cells
To confirm the role of the Wnt/β-catenin signaling pathway in the anticancer effect of sinensetin on breast cells, cells were treated with the Wnt agonist SKL2001 (40 µM) (33) for 1 h before exposure to sinensetin (32). The results showed that SKL2001 increased the protein levels of β-catenin, LEF1, and TCF1/TCF7 in MCF cells (Figure 5A). SKL2001 increased the Bcl-2/Bax ratio (Figure 5B) and inhibited breast cancer cell apoptosis induced by sinensetin (Figure 5C). In addition, SKL20001 upregulated Vimentin and downregulated E-cadherin expression in MCF7 cells (Figure 5B). Transwell assay results showed that SKL20001 promoted the invasion of MCF7 cells exposed to sinensetin (Figure 5D). These results demonstrated that the Wnt agonist SKL20001 reversed the anticancer effect of sinensetin on breast cancer cells, suggesting that sinensetin suppressed breast cancer cell growth by inhibiting the Wnt/β-catenin signaling pathway.
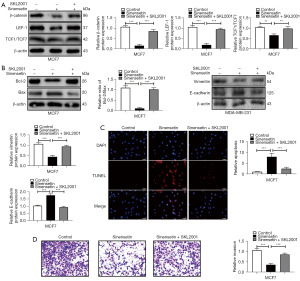
Discussion
Despite significant advances in the diagnosis and treatment of breast cancer, metastasis to distant organs remains the leading cause of death (39,40). Metastatic breast cancer spreads to other parts of the body. Because of its non-specific effect, it can only be treated by systemic chemotherapy, which is associated with toxicity and other side effects. Drugs targeting the estrogen receptor, progesterone receptor, or human epidermal growth factor receptor 2 (HER2) are only used for tumors that express the corresponding receptors (40). However, a common breast cancer subtype, TNBC, lacks these three receptors and is not suitable for existing targeted therapies. TNBC patients account for 12% of all breast cancer patients. Although this disease initially responds to primary chemotherapy, the recurrence rate is high and the survival rate is low (39,40).
The present study demonstrated that sinensetin derived from citrus species repressed the viability of breast cancer cells (MCF7 and MDA-MB-231 cell lines). Sinensetin is a PMF isomer that plays an important role in various cancers. Therefore, we explored the potential anticancer effect of sinensetin on breast cancer. The results showed that sinensetin increased apoptosis of MCF7 and MDA-MB-231 cells. Sinensetin upregulated the expression of apoptotic markers including cleaved-caspase 3 and cleaved-caspase 9 and decreased the Bcl-2/Bax ratio. To investigate whether sinensetin could inhibit the metastasis of breast cancer cells, we performed Western blotting, Clone formation assays, and Transwell invasion assays to analyze proliferation, migration, invasion, and EMT. The results showed that sinensetin suppressed proliferation, migration, and invasion by affecting EMT.
The IC50 of a drug is generally related to the purity of the drug, experimental conditions, cell type, culture medium type and other factors. Compared with the study by Androutsopoulos et al. (41), different cell lines were used in our study. Sinensetin used in this study also come from a different manufacturer. Meanwhile, Manthey et al. have reported that the IC50 of flavonoids occurred below 10 µM in many cases including breast cancer cells (42). Compared to their study (41,42), CCK-8 was used to determine IC50 rather than MTT [3-(4,5-dimethyl-2-thiazolyl)-2,5-diphenyl-2-H-tetrazolium bromide, Thiazolyl Blue Tetrazolium Bromide] in this study. Interestingly, Kim et al. demonstrated that the IC50 of sinensetin was more than 100 µM in Hepatocellular Carcinoma HepG2 Cells (43). Sinensetin used in their study also come from MedChemExpress. These might be the reasons why our IC50 differed from previous studies. The result obtained from the clone formation assay could be due to an effect on the cell survival rather than an effect on cell proliferation. In fact, the results in Figure 2A showed that cell proliferation was suppressed obviously by 120 µM sinensetin under these conditions. Therefore, we hypothesized that sinensetin was able to inhibit the proliferation of breast cancer cells. Due to experimental conditions, we could not perform flow cytometry to detect breast cancer cell cycle analysis. These results of proliferation needed to be confirmed by follow-up studies.
Natural drugs and compounds can act against the aggressiveness of breast cancer, inhibiting cancer cell proliferation and regulating cancer-related pathways (44). For example, 3,3'-diindolylmethane, which is obtained from broccoli, cauliflower, and cabbage, can decrease hypoxia inducible factor (HIF)-1α expression and activity in breast cancer under a hypoxic environment (45). 3,3'-diindolylmethane causes breast cancer apoptosis by downregulating Bcl-2 and cdc25A and upregulating p21 (WAF1) expression (46). Jasmonates restrain the growth of breast cancer cells though the mTOR signaling pathway (47). Xanthohumol inhibits breast cancer cell survival by decreasing the activity of the Notch signaling pathway (48). The Wnt/β-catenin signaling pathway is involved many cancer processes. Its involvement in cell migration, embryonic development, and polarization has been reported in recent years (49). The activity of this pathway is closely related to various aspects of human carcinogenesis, such as cell viability, cell cycle regulation, EMT, and stem cell maintenance. β-catenin accumulation in the nucleus of cancer cells results in the continuous activation of TCF/LEF transcription factors (50). The Wnt/β-catenin signaling pathway is activated in breast cancer, which increases the metastasis of breast cancer (41). Small molecules derived from natural products can be used to target the Wnt/β-catenin pathway to inhibit the metastasis of cancer (51). Astragaloside, a natural drug, attenuates Wnt/β-catenin signaling through frizzled-related protein-4 secreted by Wnt antagonists, thereby inhibiting breast CSC-like cells (52). To explore whether sinensetin inhibits breast cancer cells by suppressing Wnt/β-catenin signaling, we detected the expression of proteins related to the Wnt/β-catenin signaling pathway. The results showed that sinensetin decreased the mRNA and protein expression of β-catenin, LEF1, TCF1/TCF7, and TCF3/TCF7L1 in MCF7 and MDA-MB-231 cells. Furthermore, treatment of MCF7 cells with the Wnt agonist SKL20001 for 1 h before sinensetin treatment increased the protein expression of β-catenin, LEF1, and TCF1/TCF7 in MCF7 cells exposed to sinensetin. SKL20001 also increased the survival and invasion of MCF7 cells exposed to sinensetin. These results indicated that sinensetin exerted anticancer effects on breast cancer cells through the Wnt/β-catenin pathway.
At present, there are many treatments for breast cancer including surgery, radiotherapy, chemotherapy, estrogen receptor modulators, estrogen receptor antagonists, sulfatase inhibitors, immune checkpoint inhibitors, and so on (53-58). Task of treating breast cancer remains daunting. On the other hand, compounds obtained from natural products have clinical potential as therapeutic agents (59). Androutsopoulos et al. (41) have firstly reported that sinensetin inhibited strongly in vitro proliferation of MDA-MB-468 cells at submicromolar and micromolar concentrations by CYP1. Compared with their study, our study demonstrated that sinensetin also suppressed the progression of MCF7 and MDA-MB-231 cells via Wnt/β-catenin pathway inhibition. Two studies complement each other and together confirm the inhibitory effect of sinensetin on breast cancer.
Conclusions
In summary, the present study demonstrates that sinensetin inhibits breast cancer cell viability and decreases cell proliferation and invasion by affecting EMT. The effects of sinensetin on breast cancer cells may be mediated by the inhibition of the Wnt/β-catenin signaling pathway. The present study is the first to demonstrate that sinensetin exerts anticancer effects on breast cancer cells, suggesting the therapeutic potential of sinensetin for the treatment of breast cancer.
Acknowledgments
Funding: None.
Footnote
Reporting Checklist: The authors have completed the MDAR reporting checklist. Available at https://tcr.amegroups.com/article/view/10.21037/tcr-23-1317/rc
Data Sharing Statement: Available at https://tcr.amegroups.com/article/view/10.21037/tcr-23-1317/dss
Peer Review File: Available at https://tcr.amegroups.com/article/view/10.21037/tcr-23-1317/prf
Conflicts of Interest: All authors have completed the ICMJE uniform disclosure form (available at https://tcr.amegroups.com/article/view/10.21037/tcr-23-1317/coif). The authors have no conflicts of interest to declare.
Ethical Statement: The authors are accountable for all aspects of the work in ensuring that questions related to the accuracy or integrity of any part of the work are appropriately investigated and resolved.
Open Access Statement: This is an Open Access article distributed in accordance with the Creative Commons Attribution-NonCommercial-NoDerivs 4.0 International License (CC BY-NC-ND 4.0), which permits the non-commercial replication and distribution of the article with the strict proviso that no changes or edits are made and the original work is properly cited (including links to both the formal publication through the relevant DOI and the license). See: https://creativecommons.org/licenses/by-nc-nd/4.0/.
References
- Lin J, Yin X, Zeng Y, et al. Progress and prospect: Biosynthesis of plant natural products based on plant chassis. Biotechnol Adv 2023;69:108266. [Crossref] [PubMed]
- Newman DJ, Cragg GM. Natural Products as Sources of New Drugs over the Nearly Four Decades from 01/1981 to 09/2019. J Nat Prod 2020;83:770-803. [Crossref] [PubMed]
- Montané X, Kowalczyk O, Reig-Vano B, et al. Current Perspectives of the Applications of Polyphenols and Flavonoids in Cancer Therapy. Molecules 2020;25:3342. [Crossref] [PubMed]
- de Luna FCF, Ferreira WAS, Casseb SMM, et al. Anticancer Potential of Flavonoids: An Overview with an Emphasis on Tangeretin. Pharmaceuticals (Basel) 2023;16:1229. [Crossref] [PubMed]
- Torello CO, Alvarez MC, Olalla Saad ST. Polyphenolic Flavonoid Compound Quercetin Effects in the Treatment of Acute Myeloid Leukemia and Myelodysplastic Syndromes. Molecules 2021;26:5781. [Crossref] [PubMed]
- Cotoraci C, Ciceu A, Sasu A, et al. The Anti-Leukemic Activity of Natural Compounds. Molecules 2021;26:2709. [Crossref] [PubMed]
- Xu Y, Lv X, Yang G, et al. Simultaneous separation of six pure polymethoxyflavones from sweet orange peel extract by high performance counter current chromatography. Food Chem 2019;292:160-5. [Crossref] [PubMed]
- Kubatka P, Koklesova L, Mazurakova A, et al. Cell plasticity modulation by flavonoids in resistant breast carcinoma targeting the nuclear factor kappa B signaling. Cancer Metastasis Rev 2023; Epub ahead of print. [Crossref] [PubMed]
- Melfi F, Carradori S, Mencarelli N, et al. Natural products as a source of new anticancer chemotypes. Expert Opin Ther Pat 2023; Epub ahead of print. [Crossref] [PubMed]
- Borah N, Gunawardana S, Torres H, et al. 5,6,7,3’,4’,5’-Hexamethoxyflavone inhibits growth of triple-negative breast cancer cells via suppression of MAPK and Akt signaling pathways and arresting cell cycle. Int J Oncol 2017;51:1685-93. [Crossref] [PubMed]
- Kim E, Kim YJ, Ji Z, et al. ROR activation by Nobiletin enhances antitumor efficacy via suppression of IκB/NF-κB signaling in triple-negative breast cancer. Cell Death Dis 2022;13:374. [Crossref] [PubMed]
- Fan S, Xu H, Liu H, et al. Inhibition of cancer cell growth by Tangeretin flavone in drug-resistant MDA-MB-231 human breast carcinoma cells is facilitated via targeting cell apoptosis, cell cycle phase distribution, cell invasion and activation of numerous Caspases. J BUON 2019;24:1532-7. [PubMed]
- Sun Y, Duan X, Wang F, et al. Inhibitory effects of flavonoids on glucose transporter 1 (GLUT1): From library screening to biological evaluation to structure-activity relationship. Toxicology 2023;488:153475. [Crossref] [PubMed]
- Li X, Li Y, Wang Y, et al. Sinensetin suppresses angiogenesis in liver cancer by targeting the VEGF/VEGFR2/AKT signaling pathway. Exp Ther Med 2022;23:360. [Crossref] [PubMed]
- Kim SM, Rampogu S, Vetrivel P, et al. Transcriptome analysis of sinensetin-treated liver cancer cells guided by biological network analysis. Oncol Lett 2021;21:355. [Crossref] [PubMed]
- Huang B, Zhai M, Qin A, et al. Sinensetin flavone exhibits potent anticancer activity against drug-resistant human gallbladder adenocarcinoma cells by targeting PTEN/PI3K/AKT signalling pathway, induces cellular apoptosis and inhibits cell migration and invasion. J BUON 2020;25:1251-6. [PubMed]
- Pereira CV, Duarte M, Silva P, et al. Polymethoxylated Flavones Target Cancer Stemness and Improve the Antiproliferative Effect of 5-Fluorouracil in a 3D Cell Model of Colorectal Cancer. Nutrients 2019;11:326. [Crossref] [PubMed]
- Chen P, Chen F, Guo Z, et al. Recent advancement in bioeffect, metabolism, stability, and delivery systems of apigenin, a natural flavonoid compound: challenges and perspectives. Front Nutr 2023;10:1221227. [Crossref] [PubMed]
- Lawton TJ. Update on the Use of Molecular Subtyping in Breast Cancer. Adv Anat Pathol 2023;30:368-73. [Crossref] [PubMed]
- Gomes I, Abreu C, Costa L, et al. The Evolving Pathways of the Efficacy of and Resistance to CDK4/6 Inhibitors in Breast Cancer. Cancers (Basel) 2023;15:4835. [Crossref] [PubMed]
- Shivnani P, Shekhawat S, Prajapati A. Cancer Cachexia and breast cancer stem cell signalling – A crosstalk of signalling molecules. Cell Signal 2023;110:110847. [Crossref] [PubMed]
- Doloi R, Gupta SM. MicroRNAs: The key players regulating the crosstalk between Hippo and Wnt/β-catenin pathways in breast cancer. Life Sci 2023;329:121980. [Crossref] [PubMed]
- Yokoyama N, Nakayama H, Iwabuchi K. Novel Insights into the Role of Kras in Myeloid Differentiation: Engaging with Wnt/β-Catenin Signaling. Cells 2023;12:322. [Crossref] [PubMed]
- Koelman EMR, Yeste-Vázquez A, Grossmann TN. Targeting the interaction of β-catenin and TCF/LEF transcription factors to inhibit oncogenic Wnt signaling. Bioorg Med Chem 2022;70:116920. [Crossref] [PubMed]
- Moon SJ, Choi HJ, Kye YH, et al. CTTN Overexpression Confers Cancer Stem Cell-like Properties and Trastuzumab Resistance via DKK-1/WNT Signaling in HER2 Positive Breast Cancer. Cancers (Basel) 2023;15:1168. [Crossref] [PubMed]
- Mandal S, Gamit N, Varier L, et al. Inhibition of breast cancer stem-like cells by a triterpenoid, ursolic acid, via activation of Wnt antagonist, sFRP4 and suppression of miRNA-499a-5p. Life Sci 2021;265:118854. [Crossref] [PubMed]
- Kasprzak A. Angiogenesis-Related Functions of Wnt Signaling in Colorectal Carcinogenesis. Cancers (Basel) 2020;12:3601. [Crossref] [PubMed]
- Gao Y, Chen N, Fu Z, et al. Progress of Wnt Signaling Pathway in Osteoporosis. Biomolecules 2023;13:483. [Crossref] [PubMed]
- Hsueh YC, Hodgkinson CP, Gomez JA. The role of Sfrp and DKK proteins in cardiomyocyte development. Physiol Rep 2021;9:e14678. [Crossref] [PubMed]
- Wu XL, Lin SG, Mao YW, et al. Wnt/β-catenin signalling pathway in breast cancer cells and its effect on reversing tumour drug resistance by alkaloids extracted from traditional Chinese medicine. Expert Rev Mol Med 2023;25:e21. [Crossref] [PubMed]
- Castagnoli L, Tagliabue E, Pupa SM. Inhibition of the Wnt Signalling Pathway: An Avenue to Control Breast Cancer Aggressiveness. Int J Mol Sci 2020;21:9069. [Crossref] [PubMed]
- Xu WN, Zheng HL, Yang RZ, et al. Mitochondrial NDUFA4L2 attenuates the apoptosis of nucleus pulposus cells induced by oxidative stress via the inhibition of mitophagy. Exp Mol Med 2019;51:1-16. [Crossref] [PubMed]
- Liao S, Chen H, Liu M, et al. Aquaporin 9 inhibits growth and metastasis of hepatocellular carcinoma cells via Wnt/β-catenin pathway. Aging (Albany NY) 2020;12:1527-44. [Crossref] [PubMed]
- Lazarian G, Friedrich C, Quinquenel A, et al. Stabilization of β-catenin upon B-cell receptor signaling promotes NF-kB target genes transcription in mantle cell lymphoma. Oncogene 2020;39:2934-47. [Crossref] [PubMed]
- Zhang X, Yu X. Crosstalk between Wnt/β-catenin signaling pathway and DNA damage response in cancer: a new direction for overcoming therapy resistance. Front Pharmacol 2023;14:1230822. [Crossref] [PubMed]
- Shen X, Gao C, Li H, et al. Natural compounds: Wnt pathway inhibitors with therapeutic potential in lung cancer. Front Pharmacol 2023;14:1250893. [Crossref] [PubMed]
- Pandey P, Khan F, Seifeldin SA, et al. Targeting Wnt/β-Catenin Pathway by Flavonoids: Implication for Cancer Therapeutics. Nutrients 2023;15:2088. [Crossref] [PubMed]
- Mukherjee N, Panda CK. Wnt/β-Catenin Signaling Pathway as Chemotherapeutic Target in Breast Cancer: An Update on Pros and Cons. Clin Breast Cancer 2020;20:361-70. [Crossref] [PubMed]
- Muñoz JP, Pérez-Moreno P, Pérez Y, et al. The Role of MicroRNAs in Breast Cancer and the Challenges of Their Clinical Application. Diagnostics (Basel) 2023;13:3072. [Crossref] [PubMed]
- Miziak P, Baran M, Błaszczak E, et al. Estrogen Receptor Signaling in Breast Cancer. Cancers (Basel) 2023;15:4689. [Crossref] [PubMed]
- Androutsopoulos VP, Ruparelia K, Arroo RR, et al. CYP1-mediated antiproliferative activity of dietary flavonoids in MDA-MB-468 breast cancer cells. Toxicology 2009;264:162-70. [Crossref] [PubMed]
- Manthey JA, Guthrie N. Antiproliferative activities of citrus flavonoids against six human cancer cell lines. J Agric Food Chem 2002;50:5837-43. [Crossref] [PubMed]
- Kim SM, Ha SE, Lee HJ, et al. Sinensetin Induces Autophagic Cell Death through p53-Related AMPK/mTOR Signaling in Hepatocellular Carcinoma HepG2 Cells. Nutrients 2020;12:2462. [Crossref] [PubMed]
- Mitra S, Dash R. Natural Products for the Management and Prevention of Breast Cancer. Evid Based Complement Alternat Med 2018;2018:8324696. [Crossref] [PubMed]
- Riby JE, Firestone GL, Bjeldanes LF. 3,3’-diindolylmethane reduces levels of HIF-1alpha and HIF-1 activity in hypoxic cultured human cancer cells. Biochem Pharmacol 2008;75:1858-67. [Crossref] [PubMed]
- Rahman KW, Li Y, Wang Z, et al. Gene expression profiling revealed survivin as a target of 3,3’-diindolylmethane-induced cell growth inhibition and apoptosis in breast cancer cells. Cancer Res 2006;66:4952-60. [Crossref] [PubMed]
- Bömer M, Pérez-Salamó I, Florance HV, et al. Jasmonates induce Arabidopsis bioactivities selectively inhibiting the growth of breast cancer cells through CDC6 and mTOR. New Phytol 2021;229:2120-34. [Crossref] [PubMed]
- Sun Z, Zhou C, Liu F, et al. Inhibition of breast cancer cell survival by Xanthohumol via modulation of the Notch signaling pathway in vivo and in vitro. Oncol Lett 2018;15:908-16. [PubMed]
- Martin-Orozco E, Sanchez-Fernandez A, Ortiz-Parra I, et al. WNT Signaling in Tumors: The Way to Evade Drugs and Immunity. Front Immunol 2019;10:2854. [Crossref] [PubMed]
- Yamaguchi K, Nagatoishi S, Tsumoto K, et al. Discovery of chemical probes that suppress Wnt/β-catenin signaling through high-throughput screening. Cancer Sci 2020;111:783-94. [Crossref] [PubMed]
- Liu D, Chen L, Zhao H, et al. Small molecules from natural products targeting the Wnt/β-catenin pathway as a therapeutic strategy. Biomed Pharmacother 2019;117:108990. [Crossref] [PubMed]
- Bhuvanalakshmi G. Breast Cancer Stem-Like Cells Are Inhibited by Diosgenin, a Steroidal Saponin, by the Attenuation of the Wnt β-Catenin Signaling via the Wnt Antagonist Secreted Frizzled Related Protein-4. Front Pharmacol 2017;8:124. [Crossref] [PubMed]
- Abdel-Razeq H. Surgical options for patients with early-stage breast cancer and pathogenic germline variants: an oncologist perspectives. Front Oncol 2023;13:1265197. [Crossref] [PubMed]
- Kaidar-Person O, Offersen BV, Tramm T, et al. The King is in the altogether: Radiation therapy after oncoplastic breast surgery. Breast 2023;72:103584. [Crossref] [PubMed]
- Tarekegn K, Keskinkilic M, Kristoff TJ, et al. The role of immune checkpoint inhibition in triple negative breast cancer. Expert Rev Anticancer Ther 2023;23:1095-106. [Crossref] [PubMed]
- Ferro A, Generali D, Caffo O, et al. Oral selective estrogen receptor degraders (SERDs): The new emperors in breast cancer clinical practice? Semin Oncol 2023;50:90-101. [Crossref] [PubMed]
- Anbar HS, Isa Z, Elounais JJ, et al. Steroid sulfatase inhibitors: the current landscape. Expert Opin Ther Pat 2021;31:453-72. [Crossref] [PubMed]
- Mohamed A, Kruse M, Tran J. Progress in immune checkpoint inhibition in early-stage triple-negative breast cancer. Expert Rev Anticancer Ther 2023;23:1071-84. [Crossref] [PubMed]
- Newman DJ, Cragg GM. Natural products as sources of new drugs over the last 25 years. J Nat Prod 2007;70:461-77. [Crossref] [PubMed]