Fusion of NY-ESO-1 epitope with heat shock protein 70 enhances its induced immune responses and antitumor activity against glioma in vitro
Highlight box
Key findings
• HSP70/NY-ESO-1 p86-94 protein can significantly enhance the specific immune response against NY-ESO-1+ U251 human glioma cells in vitro.
What is known and what is new?
• There are some studies on immunotherapy against some malignant tumors except human glioma with HSP70 or NY-ESO-1, but there are few related studies in glioma, especially peptide antigen vaccine.
• There are few related studies in glioma, especially peptide antigen vaccine. Our works suggest that NY-ESO-1 p86-94 antigen peptide could be used as a new immunotherapy target.
What is the implication, and what should change now?
• Combining 5-Aza-CdR treatment with the fusion of HSP70 to NY-ESO-1 p86-94 is a promising strategy for immunotherapy against glioma with poor cancer testis antigen expression.
• We will conduct experiments to further verify this conclusion, including other glioma cell lines and in vivo.
Introduction
Glioma is the most common form of primary tumor in the central nervous system with poor prognosis; thus, new effective treatment strategies for glioma are urgently needed. Recent studies have shown that glioblastoma cells express or can be induced to express some known cancer testis antigens (CTAs) (1,2). CTAs are potential targets for cancer immunotherapy due to the limited expression in various tumors and normal tissues (3,4). Although more than 200 CTA group proteins have been documented in the CT database (www.cta.lncc.br) (5), only a limited number of them can induce both humoral and cellular immune responses.
New York esophageal squamous cell carcinoma 1 (NY-ESO-1) is a known CTA with prevalent expression in many cancer types (6,7). Because it can elicit robust humoral and cellular immune responses and has restricted expression patterns, NY-ESO-1 is an attractive candidate target for cancer immunotherapy (8). Multiple immunotherapeutic methods incorporating NY-ESO-1 have been developed recently (9-11). NY-ESO-1 vaccines have been tested to treat the NY-ESO-1-expressing tumors, including lung cancer, esophageal cancer, myeloma (12), and melanoma (6,13). A preclinical mouse model study on lung cancer immunization with a dendritic cell (DC)-targeting integration-deficient lentiviral vector showed promising results (14). However, only a tiny proportion of gliomas express NY-ESO-1 protein (15); consequently, basic CTA-based immunotherapy is unsuitable for treating brain tumors. To overcome this limitation and stimulate strong immune responses against gliomas, we need new strategies for CTA induction.
Epigenetic alteration, such as the hypermethylation of promoter CpG islands, is an important contributor to carcinogenesis in humans. The low NY-ESO-1 expression in gliomas could be the result of a lack of epigenetic regulation. Demethylating agents can increase the expression of tumor-associated antigens (TAAs), which consequently improves the presentation of TAAs to T cells (16). The DNA demethylating agent 5-Aza-2'-deoxycytidine (5-Aza-CdR) can be incorporated into DNA during replication and may deregulate the DNA methylation level. 5-Aza-CdR can activate CTA transcription by silencing the hypermethylation of CTA promoters (17-19). Thus, 5-Aza-CdR treatment may enhance antitumor immune responses and 5-Aza-CdR can be a new target for cancer immunotherapy.
Tumor antigen peptides that are bound by heat shock proteins (HSPs), which can act as critical molecular chaperones of tumor antigens, may induce a stronger immune response compared with unbound tumor antigen peptides. The addition of HSP as a strategy to enhance the efficacy of cancer vaccines has been widely explored in tumor immunotherapy (20-22). HSP-peptide complexes were reported to successfully mediate the cross-presentation of antigen on human leukocyte antigen (HLA) class I- and class II-restricted epitopes (23).
Cytotoxic T lymphocytes (CTLs) are the main effector cells in anti-tumor immunity. CTLs can specifically kill target cells by recognizing antigenic peptide-MHC complexes on the surface of target cells. Cytotoxicity assays are used to determine the killing ability of CTLS.
Here, by prokaryotic expression and protein purification techniques, HSP70 and NY-ESO-1 p86-94 peptide were fused to stimulate peripheral blood monocyte DCs. NY-ESO-1 expression in U251 glioma cells was then induced by 5-Aza-CdR treatment, and the killing efficiency against these cells was measured. We aimed to determine if HSP70/NY-ESO-1 p86-94 could enhance the specific immune response against NY-ESO-1+ U251 cells in vitro. We present this article in accordance with the MDAR reporting checklist (available at https://tcr.amegroups.com/article/view/10.21037/tcr-23-1476/rc).
Methods
The cell line and plasmid
The human hepatocellular carcinoma (HCC) cell line MHCC-97H and human glioma cell line U251 were obtained from the Cell Bank of the Chinese Academy of Science (Shanghai, China). Prokaryotic expression plasmid pET30a (Promega, Madison, WI, USA) was kept in our laboratory. Cells were routinely cultured in humidified incubator with 5% CO2 at 37 ℃.
Expression and purification of human HSP70 protein
The construction of human HSP70 prokaryotic expression plasmid
RNA was extracted from HCC cell line MHCC-97H using Trizol (Invitrogen, Carlsbad, CA, USA). HSP70 gene was cloned from RNA by reverse transcription-polymerase chain reaction (RT-PCR). The human HSP70 gene sequence (GenBank: NM_005345.5) was used as a reference for primer design. The full length HSP70 gene was amplified by forward (5'-cggaattcatggccaaagccgcggc-3') and reverse (5'-cccaagcttttaatctacctcctcaatgg-3') primers flanked by EcoR1 and Hind III restriction sites. The reaction program was composed of denaturation for 5 min at 95 ℃, 10 s at 98 ℃, 15 s at 61 ℃ and 2 min at 72 ℃ for 30 cycles, and a final extension for 10 min at 72 ℃. The obtained PCR product was cloned into pET30a vector, yielding the recombinant expression vector pET30a-HSP70, which was then verified by enzymatic digestion and DNA sequencing.
The purification of human HSP70 protein
The recombinant pET30a-HSP70 vector was transformed into Escherichia coli Rosetta (DE3) (Novagen, Madison, WI, USA). After the bacterial culture grew to an optical density of about 0.5 at 600 nm, 0.1 mM isopropyl β-d-thiogalactoside (IPTG) (Sigma, St Louis, MO, USA) was added to the medium to induce protein expression. After incubation for 4 h at 32 ℃, the bacteria were collected by centrifugation. NP-40 was added to the cell suspension with final concentration of 0.1%, and lysozyme was added to the cell paste with final concentration of 45 kU/g. The resulting cell spheres were treated to suspend the cells and cut the DNA. Because the target protein had a His-tag, an affinity chromatography column with His-tag was used in accordance with the manufacturer’s instructions (BugBuster Master Mix; Merck KGaA, Darmstadt, Germany) to purify the HSP70 protein. The quantification of HSP70 protein was determined by bicinchoninic acid (BCA).
Synthesis of HSP70 and NY-ESO-1 p86-94 complex
In previous experiments, CTLs induced by DCs loaded with NY-ESO-1 polypeptide p86-94 (RLLEFYLAM) had a strong killing ability for both HLA-A2-positive and NY-ESO-1-positive MHCC-97h HCC cells and on SW480 colon cancer cells. We selected NY-ESO-1 p86-94 to connect with HSP70 in vitro. Polypeptide NY-ESO-1 p86-94 was synthesized at GL Biochem Ltd. (Shanghai, China). The aggregation of HSP70 and NY-ESO-1 p86-94 epitope (HSP70/NY-ESO-1 p86-94) was synthesized according to the method of Li et al. (24). NY-ESO-1 p86-94 (1 mg/mL) and purified HSP70 protein (1.198 mg/mL) were mixed in a phosphate-buffered saline (PBS) solution containing 2 mM MgCl2 at 37 ℃ for 1 h at a molar ratio of about 70:1. Then 100 mM ADP (final concentration of 0.5 mM) was added, and the mixture was allowed to react for 1 h at 37 ℃. Finally, HSP70/NY-ESO-1 p86-94 was purified and concentrated by using an ultrafiltration centrifuge (Millipore, Billerica, MA, USA).
Induction and identification of DCs
Peripheral blood mononuclear cells (PBMCs) were obtained from healthy staff in our laboratory by Ficoll-Hypaque (Amersham Biosciences, Uppsala, Sweden) method according to the manufacturer’s instruction. The study was conducted in accordance with the Declaration of Helsinki (as revised in 2013). The study was approved by the Ethics Committee of Fujian Medical University (No. [2019(45)]). Informed consent was taken from all the donors. The expression of HLA-A2 on the surface of PBMCs was detected by flow cytometry, and HLA-A2 positive PBMCs were selected for DC induction and culture. The cells were inoculated at the density of 2–3×106 cells/mL on a 6-well plate for 2 h. Non-adherent cells were removed, and adherent cells were maintained in RPMI-1640 medium containing 20% fetal bovine serum (FBS), 1,000 U/mL GM-CSF (PeproTech, Rocky Hill, NJ, USA), and 500 U/mL interleukin (IL)-4 (PeproTech). The next day, fresh medium was added, and after 5 days of culture, 1/3 of the cells were collected as immature DCs (imDCs). The remaining cells were treated with HSP70/NY-ESO-1 p86-94 or 50 µg/mL IL-4 for 24 h, followed by 10 ng/mL of TNF-α for 48 hours to induce the formation of mature DCs (mDCs). The DCs were evaluated for the expression of HLA-DR, CD83, and CD86 by flow cytometry. The function of DCs was identified by performing mixed lymphocyte reaction assay.
Mixed lymphocyte reaction
T cells were separated from PBMCs using magnetic beads and then incubated in RPMI-1640 medium supplemented with 20% FBS, 100 U/mL IL-2 (PeproTech), and 20 µg/mL phytohaemagglutinin (PHA). T cells were mixed with either imDCs or mDCs at a 20:1 ratio for 72 h. T-cell proliferation was monitored by MTT assay. The absorbance at 570 nm was measured with a Multi-Well Plate Reader (Beckman Coulter, Brea, CA, USA). The proliferation index (PI) was calculated according to the formula: PI = mixed lymphocyte reaction/lymphocyte reaction. The experiment was conducted 3 times.
Flow cytometry
The levels of HLA-A2 expression on PBMCs and HLA-DR, CD83, and CD86 expression on DCs were detected by flow cytometry performed in accordance with the instructions from BioLegend (San Diego, CA, USA). Briefly, 1×106 cells/mL suspended in PBS were incubated with the following anti-human antibodies (2 µg/106 cells): PE-HLA-A2, FITC-CD83, PE-CD86, and APC-HLA-DR (BioLegend). The cells were analyzed at room temperature in the dark for 30 min using the FACS Calibur software (Becton Dickinson, Franklin Lakes, NJ, USA). Fluorescein-conjugated isotype-matched unrelated antibodies were used as negative controls.
Induction of NY-ESO-1 in U251 human glioma cells
We confirmed that the human glioma cell line U251 does not express NY-ESO-1 by RT-PCR and immunohistochemistry. In order to induce NY-ESO-1 expression, U251 was cultured for 6 days in RPMI-1640 containing 1, 5, or 10 µmol/L 5-Aza-CdR (Sigma). Replace with fresh medium every 2–3 days. The expression level of NY-ESO-1 on U251 cells was detected by performing RT-PCR and immunocytochemical assays. Total RNA was extracted and reverse-transcribed into cDNA with the First Strand cDNA synthesis kit (MBI Fermentas, Vilnius, Lithuania). The following primers were used for amplification: NY-ESO-1 forward (exon 1; 5'-cgcctgcttgagttctacctc-3') and NY-ESO-1 reverse (exon 3; 5'-agggaaagctgctggagacag-3'). The reaction was carried out for 35 cycles under the following conditions: 5 min at 95 ℃, 30 s at 94 ℃, 1 min at 60 ℃, and 45 s at 72 ℃, and a final 10 min extension at 72 ℃. β-actin was used as the positive control. The experiment was conducted at least three biological replicates.
Immunocytochemical staining
A suspension of U251 human glioma cells (1×105 cells/mL) was dropped onto each slide and incubated overnight at 37 ℃ and 5% CO2, after which the cells were washed with PBS and fixed with cold acetone for 10 min. Then immunocytochemical staining was performed using streptavidin-peroxidase-complex method. The slides were incubated for 16 h at 4 ℃ in the presence of a mouse anti-human NY-ESO-1 monoclonal antibody (clonal code: E978; Zymed, San Francisco, CA, USA; dilution: 3 µg/mL). The primary antibody was visualized using EnVision+ System-HRP (Dako, Carpinteria, CA, USA) for 30 min with diaminobenzidine (DAB), and counterstained with hematoxylin. Normal testicular tissue was used as positive control. The sections treated with PBS in place of the primary antibody were served as negative controls.
Cytotoxicity assays
The cytotoxic function of activated CTLs was determined by performing a standard lactate dehydrogenase (LDH) cytotoxicity assay (Pierce, Waltham, MA, USA). T cells stimulated by mDCs were used as effector cells and divided into four groups. PBS, HSP70, NY-ESO-1, and HSP70/NY-ESO-1 p86-94. U251 (NY-ESO-1+ or NY-ESO-1−) cells were used as target cells. Effector cells were co-cultured in 96-well plates with 1×105 target cells at ratios of 5:1, 10:1, or 20:1 in a final volume of 200 µL at 37 ℃ for 6 h. Then 50 µL of supernatant per well was transferred to a new plate, and 50 µL of substrate mixture was added to each well. The plate was incubated in the dark at room temperature for 30 min. Fifty µL of stop solution was added to each well, and the optical densities were detected at a wavelength of 490 nm. For each effector: target cell ratio, the cytotoxicity was calculated as follows: specific lytic percentage = (effector/target release − spontaneous release)/(maximum release − spontaneous release) × 100%.
Statistical analyses
Statistical analyses were performed using the SPSS 23.0 software package (IBM, Armonk, NY, USA). Continuous variables with a normal distribution were presented as the mean ± standard deviations (SDs). Student’s t-test was used to determine the significance of differences between two experimental groups. The one-way analysis of variance, followed by Bonferroni post-hoc test, were used to determine statistical differences among three or four experimental groups. Differences were considered significant with a P value of <0.05.
Results
Expression and purification of HSP70 protein
We expressed recombinant full-length HSP70 to determine if NY-ESO-1 and HSP70 fusion protein can be effective for vaccination against tumors expressing NY-ESO-1. A 2,000-bp gene fragment was amplified by RT-PCR and then cloned into pET30a to construct the HSP70 prokaryotic expression plasmid pET30a-HSP70 (Figure 1A). Successful construction of the pET30a-HSP70 vector was confirmed by double enzyme digestion followed by DNA sequencing (Figure 1B). Two base mutations (221st base T mutated to C; 1,695th base G mutated to C) were found compared with the NCBI BLAST HSP70 gene sequence (number: NM_005345.5), but they were synonymous mutations that did not change the translated HSP70. A high level of HSP70 expression was induced by IPTG, and a protein of the expected size (72 kD) was purified by His affinity chromatography (Figure 1C). A BCA assay revealed that the protein concentration was 1.198 mg/mL.
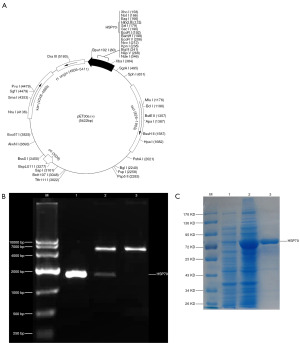
Induction and identification of DCs
HLA-A2-positive PBMCs collected using flow cytometry were treated and incubated for 5 days. Dendritic-like cells were then obtained and examined by light and electron microscopy and were observed to have irregular morphology and protrusions (Figure 2). After 24 h of incubation with HSP70/NY-ESO-1 p86-94 or NY-ESO-1 and 48 h after the addition of a tumor necrosis factor (TNF)-α, mDCs were induced and flow cytometry was performed. The levels of CD83, CD86, and HLA-DR expressed on mDCs surface induced by NY-ESO-1 (74.81%, 76.59%, 81.85%) or HSP70/NY-ESO-1 p86-94 (73.91%, 79.84%, 88.42%) were significantly higher than those in imDC group (2.50%, 17.58%, 32.66%) (P<0.01, Figure 3).
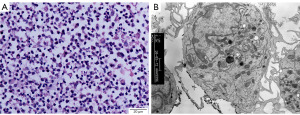
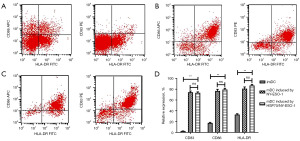
To determine the ability of mDCs on inducing T cells proliferation, the PIs of T cells incubated for 72 h with mDCs were calculated. PI of T cells incubated with HSP70/NY-ESO-1 p86-94-pulsed mDCs (3.60±0.86) was higher than that of T cells incubated with similarly treated imDCs (1.24±0.26) (P<0.05), indicating that HSP70/NY-ESO-1 p86-94-pulsed mDCs were more effective than imDCs at stimulating T-lymphocyte proliferation.
Induction of NY-ESO-1 expression by U251 glioma cells
In order to induce NY-ESO-1 expression by the glioma cell line U251, the cells were treated with a range of concentrations (1, 5, or 10 µmol/L) of 5-Aza-CdR. There were no significant differences in the tumor cell morphology or growth rate of 5-Aza-CdR-treated U251 cells compared with untreated cells. The results of RT-PCR indicated that NY-ESO-1 was not detected in 5-Aza-CdR-untreated U251 cells, whereas NY-ESO-1 was detected in 5-Aza-CdR-treated U251 cells. And the level of NY-ESO-1 in cells treated with 5 or 10 µmol/L 5-Aza-CdR was significantly higher than that in cells after 5-Aza-CdR (1 µmol/L) stimulation (P<0.01, Figure 4A,4B). Immunohistochemistry showed that 5-Aza-CdR-untreated U251 cells did not express NY-ESO-1 (Figure 4C), whereas positive expression of NY-ESO-1 in the cytoplasm of 5-Aza-CdR-untreated U251 was detected by immunohistochemistry (Figure 4D). This suggested that treatment with 5-Aza-CDR can induce the expression of NY-ESO-1 in tumor cells through demethylation.
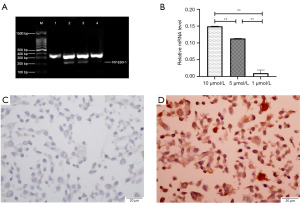
Increased induction of NY-ESO-1-specific T-cell immune responses by HSP70/NY-ESO-1 p86-94
To determine whether HSP70 can enhance T-cell-mediated NY-ESO-1-specific immune responses, the cytotoxicity of CTLs (E) against U251 cells (T) was determined by performing LDH release assays with the following four groups: PBS group (T cells treated with PBS but not stimulated by mDCs), HSP70 group, NY-ESO-1 group, and HSP70/NY-ESO-1 p86-94 group (T cells stimulated by mDCs loaded with HSP70, NY-ESO-1, or HSP70/NY-ESO-1 p86-94, respectively). U251 cells (T) were divided into two groups: U251 cells treated with 5-Aza-CdR to induce NY-ESO-1 expression (NY-ESO-1+ U251 cells) and untreated (NY-ESO-1− U251 cells). Regardless of the target cell type (NY-ESO-1+ or NY-ESO-1− U251 cells), the percentage of NY-ESO-1-specific lysis by CTLs was less than 20% for PBS or HSP70-stimulated T cells. In contrast, with NY-ESO-1+ U251 cells as target cells, the percentages of lysis by NY-ESO-1-stimulated and HSP70/NY-ESO-1 p86-94-stimulated T cells were higher than those of the controls (P<0.01); notably, there was more CTL lysis in the HSP70/NY-ESO-1 p86-94-stimulated group compared with the NY-ESO-1-stimulated group at the effector: target ratio of 10:1 and 20:1 (P<0.01) (Figure 5A). Moreover, the percentages of lysis of NY-ESO-1+ U251 target cells by NY-ESO-1-stimulated and HSP70/NY-ESO-1 p86-94-stimulated T cells were higher than those of NY-ESO-1− U251 target cells (P<0.01) (Figure 5B). These results showed that mDC stimulation with NY-ESO-1 protein induced a specific immune response and that mDC stimulation with the fusion protein HSP70/NY-ESO-1 p86-94 induced an enhanced specific immune response.
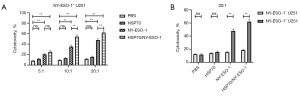
Discussion
NY-ESO-1 has strong immunogenicity, and cancer immunotherapy targeting NY-ESO-1 has achieved good results. We previously identified that NY-ESO-1 loaded DCs can stimulate antigen specific T cell responses against HCC (25). This study aimed to make a fusion protein of HSP70 and NY-ESO-1, and investigated the therapeutic potential of the fusion protein HSP70/NY-ESO-1 p86-94 for glioma. We first constructed a prokaryotic plasmid containing the gene for human HSP70, then purified the expressed HSP70 protein, and finally synthesized the fusion protein HSP70/NY-ESO-1 p86-94. The NY-ESO-1-specific lysis activity against NY-ESO-1+ U251 glioma cells in vitro was assessed. It was demonstrated that HSP70/NY-ESO-1 p86-94 can induce a stronger immune response against NY-ESO-1-expressing glioma cells compared to using NY-ESO-1 alone. Thus, combining HSPs with CTAs could be used as a strategy for increasing the immunogenicity of glioma.
Research on various NY-ESO-1-based vaccines (8,26), such as NY-ESO-1 protein, polypeptide, and DNA, has progressed rapidly in recent years. Because the frequency of HLA-A2 phenotype is high in China, we synthesized several HLA-A2-restrictive NY-ESO-1 polypeptides and performed preliminary experiments to identify their specific lysis activity against tumor cells in vitro. The nine-peptide fragment p86-94 (RLLEFYLAM) was found to elicit NY-ESO-1-specific immune responses, so HSP70/NY-ESO-1 p86-94 was used in our studies for glioma immunotherapy.
Because of the restricted expression of NY-ESO-1 on gliomas, we need to explore new induction strategies. 5-Aza-CdR is a methylation inhibitor and has been successfully used to reverse the methylation status of multiple gene promoters (27,28). Here, 5-Aza-CdR successfully induced NY-ESO-1 expression in glioma cell line U251 lacking NY-ESO-1 protein. This finding confirmed that NY-ESO-1 expression is related to gene hypermethylation and that 5-Aza-CdR is an effective inducer of NY-ESO-1 expression.
HSP70 serves as an adjuvant to augment tumor-specific immune responses. To study the ability of HSP70 to act as an adjuvant for enhancing an NY-ESO-1-based vaccine, we generated the fusion protein HSP70/NY-ESO-1 p86-94 and assessed its effectiveness in inducing a specific CTL response against glioma. The data demonstrated that, compared to vaccination with NY-ESO-1 alone, vaccination with HSP70/NY-ESO-1 p86-94 results in a greater CTLs response against NY-ESO-1+ U251 cells.
Various strategies have been developed to target DCs in tumor immunotherapy (29,30). The specific immune response against glioma induced by our HSP70/NY-ESO-1 p86-94 DC-based vaccine was significantly enhanced in vitro. Thus, it is worthwhile to further investigate the anti-tumor activity of DCs stimulated by the polypeptide complex HSP70/NY-ESO-1 p86-94.
Conclusions
In comparison to NY-ESO-1, the fusion protein HSP70/NY-ESO-1 p86-94 can induce a stronger NY-ESO-1-specific immune response in vitro. Thus, combining demethylation with the fusion of HSP70 to tumor antigens is a promising strategy for designing NY-ESO-1-based vaccines for immunotherapy against malignant tumors expressing NY-ESO-1 antigens.
Acknowledgments
We would like to thank Katie Oakley, PhD, at Liwen Bianji, Edanz Editing China (www.liwenbianji.cn/ac), for editing the English text of an early version of this manuscript. We also thank Ms. Wenwen Liu, from School of Basic Medical Science, Fujian Medical University for her excellent technical assistance.
Funding: The work was supported by grants from
Footnote
Reporting Checklist: The authors have completed the MDAR reporting checklist. Available at https://tcr.amegroups.com/article/view/10.21037/tcr-23-1476/rc
Data Sharing Statement: Available at https://tcr.amegroups.com/article/view/10.21037/tcr-23-1476/dss
Peer Review File: Available at https://tcr.amegroups.com/article/view/10.21037/tcr-23-1476/prf
Conflicts of Interest: All authors have completed the ICMJE uniform disclosure form (available at https://tcr.amegroups.com/article/view/10.21037/tcr-23-1476/coif). All authors report that the work was supported by grants from National Natural Science Foundation of China (No. 81472830) and Natural Science Foundation of Fujian Province (Nos. 2018J01835, 2022J01202). The authors have no other conflicts of interest to declare.
Ethical Statement: The authors are accountable for all aspects of the work in ensuring that questions related to the accuracy or integrity of any part of the work are appropriately investigated and resolved. The study was conducted in accordance with the Declaration of Helsinki (as revised in 2013). The study was approved by the Ethics Committee of Fujian Medical University (No. [2019(45)]). Informed consent was taken from all the donors.
Open Access Statement: This is an Open Access article distributed in accordance with the Creative Commons Attribution-NonCommercial-NoDerivs 4.0 International License (CC BY-NC-ND 4.0), which permits the non-commercial replication and distribution of the article with the strict proviso that no changes or edits are made and the original work is properly cited (including links to both the formal publication through the relevant DOI and the license). See: https://creativecommons.org/licenses/by-nc-nd/4.0/.
References
- Li X, Yan J, Fan R, et al. Serum immunoreactivity of cancer/testis antigen OY-TES-1 and its tissues expression in glioma. Oncol Lett 2017;13:3080-6. [Crossref] [PubMed]
- Ma R, Rei M, Woodhouse I, et al. Decitabine increases neoantigen and cancer testis antigen expression to enhance T-cell-mediated toxicity against glioblastoma. Neuro Oncol 2022;24:2093-106. [Crossref] [PubMed]
- Krishnadas DK, Bai F, Lucas KG. Cancer testis antigen and immunotherapy. Immunotargets Ther 2013;2:11-9. [Crossref] [PubMed]
- Seifi-Alan M, Shamsi R, Ghafouri-Fard S. Application of cancer-testis antigens in immunotherapy of hepatocellular carcinoma. Immunotherapy 2018;10:411-21. [Crossref] [PubMed]
- Ren S, Zhang Z, Li M, et al. Cancer testis antigen subfamilies: Attractive targets for therapeutic vaccine Int J Oncol 2023;62:71. (Review). [Crossref] [PubMed]
- Szender JB, Papanicolau-Sengos A, Eng KH, et al. NY-ESO-1 expression predicts an aggressive phenotype of ovarian cancer. Gynecol Oncol 2017;145:420-5. [Crossref] [PubMed]
- Tessari A, Pilla L, Silvia D, et al. Expression of NY-ESO-1, MAGE-A3, PRAME and WT1 in different subgroups of breast cancer: An indication to immunotherapy? Breast 2018;42:68-73. [Crossref] [PubMed]
- Thomas R, Al-Khadairi G, Roelands J, et al. NY-ESO-1 Based Immunotherapy of Cancer: Current Perspectives. Front Immunol 2018;9:947. [Crossref] [PubMed]
- Maruta M, Ochi T, Tanimoto K, et al. Direct comparison of target-reactivity and cross-reactivity induced by CAR- and BiTE-redirected T cells for the development of antibody-based T-cell therapy. Sci Rep 2019;9:13293. [Crossref] [PubMed]
- Mastaglio S, Genovese P, Magnani Z, et al. NY-ESO-1 TCR single edited stem and central memory T cells to treat multiple myeloma without graft-versus-host disease. Blood 2017;130:606-18. [Crossref] [PubMed]
- Nagasawa DT, Yang J, Romiyo P, et al. Bioengineered recombinant vault nanoparticles coupled with NY-ESO-1 glioma-associated antigens induce maturation of native dendritic cells. J Neurooncol 2020;148:1-7. [Crossref] [PubMed]
- Rapoport AP, Stadtmauer EA, Binder-Scholl GK, et al. NY-ESO-1-specific TCR-engineered T cells mediate sustained antigen-specific antitumor effects in myeloma. Nat Med 2015;21:914-21. [Crossref] [PubMed]
- Kerkar SP, Wang ZF, Lasota J, et al. MAGE-A is More Highly Expressed Than NY-ESO-1 in a Systematic Immunohistochemical Analysis of 3668 Cases. J Immunother 2016;39:181-7. [Crossref] [PubMed]
- Albershardt TC, Campbell DJ, Parsons AJ, et al. LV305, a dendritic cell-targeting integration-deficient ZVex(TM)-based lentiviral vector encoding NY-ESO-1, induces potent anti-tumor immune response. Mol Ther Oncolytics 2016;3:16010. [Crossref] [PubMed]
- Oi S, Natsume A, Ito M, et al. Synergistic induction of NY-ESO-1 antigen expression by a novel histone deacetylase inhibitor, valproic acid, with 5-aza-2'-deoxycytidine in glioma cells. J Neurooncol 2009;92:15-22. [Crossref] [PubMed]
- Dudzik P, Trojan SE, Ostrowska B, et al. The Epigenetic Modifier 5-Aza-2-deoxycytidine Triggers the Expression of CD146 Gene in Prostate Cancer Cells. Anticancer Res 2019;39:2395-403. [Crossref] [PubMed]
- Klar AS, Gopinadh J, Kleber S, et al. Treatment with 5-Aza-2'-Deoxycytidine Induces Expression of NY-ESO-1 and Facilitates Cytotoxic T Lymphocyte-Mediated Tumor Cell Killing. PLoS One 2015;10:e0139221. [Crossref] [PubMed]
- Natsume A, Wakabayashi T, Tsujimura K, et al. The DNA demethylating agent 5-aza-2'-deoxycytidine activates NY-ESO-1 antigenicity in orthotopic human glioma. Int J Cancer 2008;122:2542-53. [Crossref] [PubMed]
- Zhang QM, Shen N, Xie S, et al. MAGED4 expression in glioma and upregulation in glioma cell lines with 5-aza-2'-deoxycytidine treatment. Asian Pac J Cancer Prev 2014;15:3495-501. [Crossref] [PubMed]
- Baldin AV, Zamyatnin AA Jr, Bazhin AV, et al. Advances in the Development of Anticancer HSP-based Vaccines. Curr Med Chem 2019;26:427-45. [Crossref] [PubMed]
- SenGupta D, Norris PJ, Suscovich TJ, et al. Heat shock protein-mediated cross-presentation of exogenous HIV antigen on HLA class I and class II. J Immunol 2004;173:1987-93. [Crossref] [PubMed]
- Taha EA, Ono K, Eguchi T. Roles of Extracellular HSPs as Biomarkers in Immune Surveillance and Immune Evasion. Int J Mol Sci 2019;20:4588. [Crossref] [PubMed]
- Gong J, Zhang Y, Durfee J, et al. A heat shock protein 70-based vaccine with enhanced immunogenicity for clinical use. J Immunol 2010;184:488-96. [Crossref] [PubMed]
- Li J, Li KN, Gao J, et al. Heat shock protein 70 fused to or complexed with hantavirus nucleocapsid protein significantly enhances specific humoral and cellular immune responses in C57BL/6 mice. Vaccine 2008;26:3175-87. [Crossref] [PubMed]
- Chen Y, Huang A, Gao M, et al. Potential therapeutic value of dendritic cells loaded with NY-ESO-1 protein for the immunotherapy of advanced hepatocellular carcinoma. Int J Mol Med 2013;32:1366-72. [Crossref] [PubMed]
- Xue W, Metheringham RL, Brentville VA, et al. SCIB2, an antibody DNA vaccine encoding NY-ESO-1 epitopes, induces potent antitumor immunity which is further enhanced by checkpoint blockade. Oncoimmunology 2016;5:e1169353. [Crossref] [PubMed]
- Pan FP, Zhou HK, Bu HQ, et al. Emodin enhances the demethylation by 5-Aza-CdR of pancreatic cancer cell tumor-suppressor genes P16, RASSF1A and ppENK. Oncol Rep 2016;35:1941-9. [Crossref] [PubMed]
- Sheng Y, Wang H, Liu D, et al. Methylation of tumor suppressor gene CDH13 and SHP1 promoters and their epigenetic regulation by the UHRF1/PRMT5 complex in endometrial carcinoma. Gynecol Oncol 2016;140:145-51. [Crossref] [PubMed]
- Wculek SK, Cueto FJ, Mujal AM, et al. Dendritic cells in cancer immunology and immunotherapy. Nat Rev Immunol 2020;20:7-24. [Crossref] [PubMed]
- Wu L, Zhang H, Jiang Y, et al. Induction of antitumor cytotoxic lymphocytes using engineered human primary blood dendritic cells. Proc Natl Acad Sci U S A 2018;115:E4453-62. [Crossref] [PubMed]