LncRNA HEIH modulates the proliferation, migration, and invasion of hepatocellular carcinoma cells by regulating the miR-193a-5p/CDK8 axis
Highlight box
Key findings
• Silencing long non-coding RNA (lncRNA) high expression in hepatocellular carcinoma (HEIH) restrained the proliferation, migration, and invasion of hepatocellular carcinoma (HCC) cells via the microRNA 193a-5p (miR-193a-5p)/cyclin-dependent kinase 8 (CDK8) axis.
What is known, and what is new?
• Silencing lncRNA HEIH inhibits the growth and metastasis of HCC cells. MiR-193a-5p overexpression hinders the progression of HCC.
• LncRNA HEIH binds to miR-193a-5p to promote CDK8 expression in HCC cells. Silencing HEIH inhibits HCC progression via the miR-193a-5p/CDK8 axis.
What is the implication, and what should change now?
• This study provides a useful perspective for interventions in HCC progression. LncRNA HEIH could be used as a potential therapeutic target in HCC therapy in the future.
Introduction
Primary hepatocellular carcinoma (HCC) is a prevailing malignant tumor, and represents a major health-care challenge in the population (1). Previous research suggests that primary HCC is associated with genetic factors, alcoholism, obesity, viral infections, and metabolic diseases (2,3). Currently, surgical treatment is the main treatment strategy for primary HCC. However, most primary HCC is diagnosed at advanced stages, and many patients with HCC miss the opportunity for surgical treatment (4). Currently, there are no efficient methods for treating advanced HCC (5,6). Thus, further research determining the pathogenesis of HCC and identifying the causative factors in the progression of HCC is of critical significance for the development of therapeutic drugs for HCC.
Long non-coding RNA (lncRNA) is discovered as mRNA-like transcripts that do not encode proteins, ranging in length from 200 nt to 100 kb (7). There is increasing evidence that lncRNAs exert pivotal functions in the pathological processes of tumorigenesis (8,9). Multiple lncRNAs have been shown to be involved in the progression and development of HCC. For example, lncRNA-taurine upregulated 1 (TUG1) promotes the growth and metastasis of HCC (10). LncRNA CEBPA divergent transcript (CEBPA-DT) contributes to HCC metastasis via discoidin domain receptor tyrosine kinase 2 (DDR2)/beta-catenin activation (11). Further, lncRNA high expression in hepatocellular carcinoma (HEIH) has been shown to play a pro-tumor role in diverse cancer types (12,13). For example, HEIH accelerates the malignant development of gastric cancer by regulating glycolysis and autophagy (14). A high expression of HEIH is associated with a poor prognosis in non-small cell lung cancer, and contributes to the diagnosis of the disease (15). Zhao et al. demonstrated that HEIH inhibition impedes cell viability, migration and invasion, and enhances cell apoptosis in breast cancer (16). In addition, a recent study has shown that silencing HEIH suppresses HCC cell growth and metastasis (17). However, the specific action and the regulatory mechanism of HEIH in HCC have not been fully elucidated.
MicroRNA (miRNA or miR) is a non-coding RNA with a length of 20–24 nt that can regulate the stability and translation efficiency of target messenger RNA (mRNA) (18). MiRNA mediates a variety of biologic processes, such as cell growth, apoptosis, metabolic signal transduction and differentiation (19). Extensive research has been conducted on the abnormal expression of miRNA in normal and tumor tissues, and this research has emphasized the correlation between miRNA expression and cancer development (20). For example, miR-29a controls hypoxia inducible factor 1 subunit alpha (HIF-1α)/angiopoietin (ANGPT) signaling thereby inhibiting the incidence of HCC (21). MiR-1-494p has also been shown to downregulate basic helix-loop-helix ARNT like 1 (BMAL1) to promote lipid biosynthesis, resulting in the growth and metastasis of HCC (22). MiR-3180 inhibits lipid synthesis and uptake to suppress HCC progression (23). MiR-193a-5p has been shown to play an anti-tumor (ovarian cancer) or pro-tumor role (renal cell carcinoma, pancreatic cancer) in several malignant tumors, including HCC (24-28). MiR-193a-5p has been identified as a novel biomarker in the recurrence and prognosis of HCC (27). It has been reported that miR-193a-5p overexpression hampers cell viability and invasion in HCC (28). Additionally, miR-193a-5p is highly expressed in HCC tissues, and accelerates the proliferation of and inhibits the apoptosis of HCC cells (29). These studies show the important function of miR-193a-5p in HCC. However, the regulatory relationship and between HEIH and miR-193a-5p remains unverified.
In this study, a sequence of in vitro and in vivo studies were performed to examine the potential action mechanisms of HEIH, miR-193a-5p, and cyclin-dependent kinase 8 (CDK8) in HCC. It also sought to examine the underlying mechanism of HCC and identify a novel therapeutic target for HCC treatment. We present this article in accordance with the MDAR and ARRIVE reporting checklists (available at https://tcr.amegroups.com/article/view/10.21037/tcr-23-2228/rc).
Methods
Specimens
In total, 59 pairs of HCC tissues and adjacent normal tissues were acquired from HCC patients at the Second Affiliated Hospital of Hainan Medical University. None of the patients underwent chemotherapy or radiotherapy before the surgery. All the samples were stored in liquid nitrogen awaiting research. The current investigation was approved by the Ethics Committee of the Second Affiliated Hospital of Hainan Medical University (No. LW20210018). Informed consent was obtained from all the patients. This study was conducted in accordance with the Declaration of Helsinki (as revised in 2013).
Cell culture and transfection
Normal human HCC cell lines (huh7, hep3B, smmc-7721, and sk-Hep-1) and human hepatocytes (L02) were obtained from the Cell Ba 133-138nk of Type Culture Collection (CBTCC, Shanghai, China). The experimental cells were incubated in Dulbecco’s Modified Eagle Medium (DMEM; Gibco, Grand Island, NY, USA) with 10% fetal bovine serum (FBS; Gibco) at 37 ℃, 5% CO2 in a humidified incubator.
Short interfering RNA against HEIH (si-HEIH-1 and si-HEIH-2), the negative control (si-NC), the pcDNA3.1 vector targeting HEIH, and the empty vector were obtained from the RiboBio Company (Beijing, China). The miR-193a-5p mimics/inhibitor was designed by GenePharma (Shanghai, China). Hep3B and sk-Hep-1 were transfected with these plasmids using Lipofectamine 3000 (Invitrogen, Carlsbad, CA, USA). At 48 hours post-transfection, quantitative real-time polymerase chain reaction (qRT-PCR) was used to determine the efficiency of the transfection.
qRT-PCR
The total RNA was extracted from the prepared cells, and the HCC and adjacent tissues using TRIzol (Invitrogen). A Revert Aid First Strand Complementary DNA Synthesis Kit (Thermo Fisher Scientific, Waltham, MA, USA) was used to reverse transcribe the RNA into cDNA. MRNA expression was detected using SYBR Green PCR Master Mix (Takara, Dalian, China) on an ABI-Prism 7300 System (Applied Biosystem Foster City, CA, USA). The reaction conditions were as follows: 95 ℃ for 5 minutes, followed by 40 cycles of 94 ℃ for 30 s, and 60 ℃ for 30 s. The primer sequences were as follows: glyceraldehyde-3-phosphate dehydrogenase (GAPDH), forward 5'-CATCTTCTTTTGCGTCGCCA-3', reverse 5'-TTAAAAGCAGCCCTGGTGACC-3'; HEIH, forward 5'-CCTCTTGTGCCCCTTTCTT-3', reverse 5'-ATGGCTTCTCGCATCCTAT-3'; miR-193a-5p, forward 5'-GATAGCCTGATGTTATCA-3', reverse 5'-GTCTGGTGAGTTGGCG-3'; CDK8, forward 5'-GCCGGTTGTCAAATCCCTTAC-3', reverse 5'-TGTGACTGCTGTCTTGATTCCCT-3'; U6, forward 5'-CAAATTCGTGAAGCGTTCCATA-3', reverse 5'-AGTGCAGGGTCCGAGGTATTC-3'. All the data were analyzed using the 2−ΔΔCt method with GAPDH as the endogenous control.
3-(4,5-dimethylthiazol-2-yl)-2,5-diphenyl-2H-tetrazolium bromide (MTT) assays
A total of 1×106 transfected Hep3B and sk-Hep-1 cells were inoculated in 96-well plates for 24, 48, 72, and 96 hours. MTT (Solarbio, Beijing, China) was appended to the wells of 96-well plates and hatched for four hours at 37 ℃. After incubation, the samples were dissolved in dimethyl sulfoxide (Solarbio). The absorbance was estimated at 450 nm by a microplate reader (Thermo Fisher Scientific).
Wound healing assays
The transfected Hep3B and sk-Hep-1 cells (1×106) were seeded in 6-well plates and incubated overnight. After the cells reached 80–90% confluence, a horizontal line was drawn using a 10-µL pipette tip. After being incubated with serum-free medium for eight hours, the width closure was captured by a microscope (Olympus, Tokyo, Japan) at the time points of 0 and 24 hours.
Transwell assays
The transfected Hep3B and sk-Hep-1 cells were incubated in the upper chambers with Matrigel (BD Biosciences, Franklin Lakes, NJ, USA). Next, DMEM medium (with 10% FBS) was added to the lower chambers. After 24 hours, the invasive cells in the lower chambers were fixed with 4% paraformaldehyde, and the cells in the upper chambers were cleared out. Next, the cells in the lower chambers were dyed with crystal violet dye and rinsed with phosphate buffered saline. The images were obtained by a microscope (Olympus), and the numbers of invasive cells were counted in f random fields.
Dual luciferase reporter (DLR) gene assays
The potential binding sites of HEIH and miR-193a-5p were predicted using starBase 3.0 (https://rnasysu.com/encori/), and the possible target region between binding sites and miR-193a-5p was predicted by the TargetScan (https://www.targetscan.org/vert_80/). The wide-type (WT) fragment of HEIH containing the miR-193a-5p binding sites or mutant (mut) sequences were cloned into pmirGLO dual luciferase vectors (Promega, Madison, WI, USA) to generate pmirGLO-WT-HEIH or pmirGLO-Mut-HEIH. Next, the Hep3B and sk-Hep-1 cells were co-transfected with pmirGLO-WT-HEIH/pmirGLO-Mut-HEIH and miR-193a-5p mimics/NC using Lipofectamine 3000 (Invitrogen). The luciferase activity was assessed using the Dual Luciferase Reporter System (Promega).
RNA immunoprecipitation (RIP) assays
The RIP assays were conducted using the imprint RIP kit (Sigma-Aldrich, St. Louis, MO, USA). In brief, the Hep3B and sk-Hep-1 cells were lysed by RIP lysis buffer (Solarbio). Next, the lysates were hatched with Argonaute2 antibody (Ago2, ab32381, Abcam, Cambridge, MA, USA) or immunoglobulin G (IgG) antibody (negative control, ab150077, Abcam). Then, protein A magnetic beads were added to isolate the immunoprecipitation complex. RNA was transcribed using an AmpliScribe T7-Flash Biotin-RNA Transcription Kit (Epicenter, Sausalit, CA, USA). Finally, the relative enrichment of HEIH and miR-193a-5p was examined by qRT-PCR.
Western blot assays
The proteins were obtained by RIPA buffer (Thermo Fisher Scientific). Next, the obtained proteins were isolated via sodium dodecyl-sulfate polyacrylamide gel electrophoresis and transferred onto a polyvinylidene fluoride membrane. Subsequently, the membrane was hatched with appropriate primary antibodies, CDK8, ab224828 (Abcam), and β-actin (ab8227, Abcam). Next, the membrane was hatched with the corresponding secondary antibody (ab150077, Abcam). The protein blots were developed using an enhanced chemiluminescence kit (Amersham, Little Chalfont, UK).
Animal study
BALB/c nu/nu mice (6 weeks old, 18–20 g) were obtained from SPF Biotechnology Co., Ltd (Beijing, China). The experimental mice were divided into the following three groups (n=8): Blank, si-NC, and si-HEIH-1 groups. The mice in the si-NC group and si-HEIH group received a retro-orbital intravenous (i.v.) injection of si-NC or si-HEIH transfected Hep3B cells (1×106), respectively. The mice in the blank group received no treatment. Tumor volume was measured every seven days as and calculated as follows: tumor volume = (length × width2)/2. Four weeks later, all the mice were anesthetized by isoflurane inhalation and executed by cervical dislocation. The tumors were carefully excised for weighing. A protocol was prepared before the study without registration. All animal experiments were approved by the Ethics Committee of the Second Affiliated Hospital of Hainan Medical University (No. LW20210018), in compliance with national guidelines for the care and use of animals.
Statistical analysis
All the data are presented as the mean ± standard deviation and were analyzed using SPSS 22.0 statistical software (SPSS Inc., Chicago, IL, USA). Comparisons between two groups were analyzed by the t-test. Comparisons among multiple groups were analyzed by a one-way analysis of variance followed by Tukey’s test. A P value <0.05 was considered statistically significant.
Results
HEIH expression was upregulated in HCC tissues
As Figure 1A shows, HEIH expression was significantly more increased in the HCC tissues than the adjacent tissues (P<0.001). The clinicopathological features of the HCC patients are set out in Table 1. A high expression of HEIH was significantly correlated with advanced TNM stage and an absence of vascular invasion. Additionally, the qRT-PCR results showed that HEIH expression was significantly more elevated in TNM stage III/IV than TNM stage I/II (P<0.01, Figure 1B). Consistent with the data in the clinical samples, HEIH expression was significantly more increased in the HCC cell lines (Hep3B, sk-Hep-1, Huh7, and Smmc-7721) than the normal liver cell line (L02) (P<0.01, Figure 1C). Due to their relatively higher HEIH expression, the Hep3B and sk-Hep-1 cells were used in the subsequent experiments.
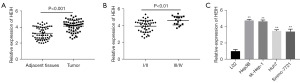
Table 1
Variable | Total | HEIH expression | P value | |
---|---|---|---|---|
Low (N=24) | High (N=35) | |||
Age | 0.372 | |||
<50 years | 23 | 11 | 12 | |
≥50 years | 36 | 13 | 23 | |
Gender | 0.109 | |||
Male | 39 | 13 | 26 | |
Female | 20 | 11 | 9 | |
Tumor size | 0.291 | |||
<5 cm | 32 | 15 | 17 | |
≥5 cm | 27 | 9 | 18 | |
AFP | 0.341 | |||
<400 ng/mL | 29 | 10 | 19 | |
≥400 ng/mL | 30 | 14 | 16 | |
TNM stage | 0.036* | |||
I/II | 43 | 21 | 22 | |
III/IV | 16 | 3 | 13 | |
Vascular invasion | 0.004** | |||
Present | 42 | 22 | 20 | |
Absent | 17 | 2 | 15 |
*, P<0.05; **, P<0.01. HEIH, high expression in hepatocellular carcinoma; HCC, hepatocellular carcinoma; AFP, alpha-fetoprotein; TNM, tumor-node-metastasis.
Silencing HEIH suppressed the viability, migration, and invasion of HCC cells in vitro, and hampered HCC tumorigenesis in vivo
To detect the function of HEIH in HCC, HEIH was inhibited by transfecting si-HEIH into the Hep3B and sk-Hep-1 cells. As expected, the qRT-PCR results indicated that HEIH expression was significantly reduced by si-HEIH-1 and si-HEIH-2 in both the Hep3B and sk-Hep-1 cells (P<0.01, Figure 2A). As it had lower HEIH expression, si-HEIH-1 was chosen for the subsequent experiments. The cell viability, wound-healing rate, and number of invasion cells were all significantly reduced by HEIH silencing in the Hep3B cells, and similar outcomes were observed in the sk-Hep-1 cells (P<0.01, Figure 2B-2D). To further validate the functional role of HEIH in vivo, xenograft models were built by inoculating Hep3B cells stably transfected with si-NC or si-HEIH into nude mice, and tumor growth was examined after 4 weeks. As Figure 2E,2F show, the tumor volume and tumor weight were significantly more decreased in the si-HEIH-1 group than the si-NC group (P<0.05). Taken together, all the above results showed that silencing HEIH impeded the viability, migration, and invasion of HCC cells in vitro, and suppressed xenograft tumor growth in vivo.
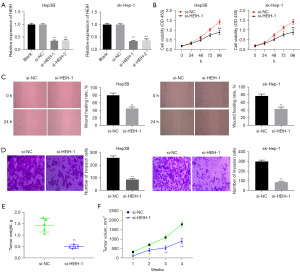
MiR-193a-5p was a target gene of HEIH
The interaction between HEIH and miR-193a-5p in HCC was examined. First, starBase was used to predict the putative binding sites of miR-193a-5p in HEIH (Figure 3A). Si-HEIH-1 significantly induced the increase of the miR-193a-5p levels in both cells (P<0.01, Figure 3B). Additionally, the binding was further confirmed by dual luciferase reporter (DLR) assays. The results showed that the relative luciferase activity was significantly decreased in the Hep3B and sk-Hep-1 cells co-transfected with miR-193a-5p mimics and HEIH-WT; however, no significant change was observed in the Hep3B and sk-Hep-1 cells co-transfected with miR-NC and HEIH-WT or HEIH-Mut (P<0.01, Figure 3C). The RIP assays indicated that HEIH and miR-193a-5p were both enriched in the precipitation of the Ago2 antibody but not in the precipitation of the IgG antibody, which suggests that they coexist in the RNA-induced silencing complex in the Hep3B and sk-Hep-1 cells (P<0.01, Figure 3D). Further, miR-193a-5p was significantly under expressed in the HCC tissues (P<0.001, Figure 3E). Subsequently, the correlation between HEIH and miR-193a-5p was examined by a Spearman’s analysis. An inverse correlation was detected between the expression of HEIH and miR-193a-5p (r=−0.0402, P=0.0016, Figure 3F). MiR-193a-5p expression was reduced in the HCC cell lines, especially in the Hep-3B and sk-Hep-1 cells (P<0.01, Figure 3G).
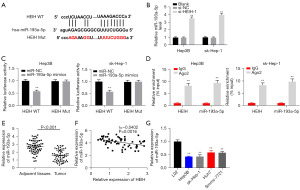
MiR-193a-5p overexpression restrained cell viability, migration, and invasion in HCC
To examine the involvement of miR-193a-5p in the functional actions of HEIH in HCC, miR-193a-5p was overexpressed via the transfection of miR-193a-5p mimics and inhibited via the transfection of a miR-193a-5p inhibitor. As expected, the level of miR-193a-5p was significantly increased in the miR-193a-5p mimics group, and significantly decreased in the miR-193a-5p inhibitor group (P<0.01, Figure 4A). The cell viability, wound-healing rate, and number of invasion cells were all significantly more reduced in the miR-193a-5p mimics group than the miR-NC group (P<0.01, Figure 4B-4D). Above all, miR-193a-5p overexpression restrained cell viability, migration, and invasion in HCC.
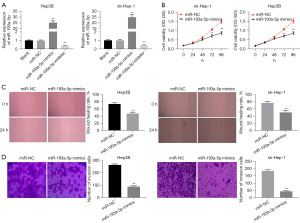
MiR-193a-5p directly targeted CDK8
As Figure 5A shows, TargetScan predicted the possible target region between CDK8 and miR-193a-5p. The DLR assay results showed that miR-193a-5p overexpression clearly decreased the luciferase activity of the cells co-transfected with CDK8 WT but not that of those co-transfected with CDK8 Mut (P<0.01). HEIH overexpression partially attenuated the reduction action of miR-193a-5p mimics on the luciferase activity of CDK8 WT (P<0.05, Figure 5B). In the RIP assays, both the expression of miR-193a-5p and CDK8 were more highly enriched in the AGO2 immunoprecipitate than the IgG immunoprecipitate (P<0.01, Figure 5C). Further, CDK8 expression was significantly more enhanced in the HCC tissues than the adjacent tissues (P<0.001, Figure 5D). In terms of the expression level, a negative correlation was observed between miR-193a-5p and CDK8 (r=−0.3419, P=0.008, Figure 5E), while a positive correlation was observed between CDK8 and HEIH (r=0.2934, P=0.0241, Figure 5F). Additionally, CDK8 was highly expressed in the Hep3B, sk-Hep-1, Huh7, and Smmc-7721 cells (P<0.01, Figure 5G).
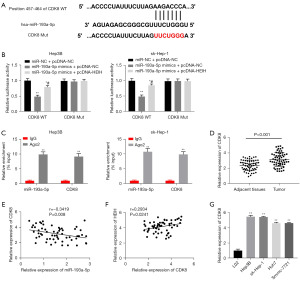
Silencing HEIH-1 impeded cell viability, migration, and invasion in HCC via the miR-193a-5p/CDK8 axis
To examine the interactions among HEIH, miR-193a-5p, and CDK8 in HCC progression, the Hep3B cells were co-transfected with miR-193a-5p mimics/miR-NC and pcDNA-NC/pcDNA-HEIH. The Western blot results revealed that the protein expression of CDK8 was significantly decreased by miR-193a-5p overexpression, and the downregulated CDK8 was attenuated by HEIH overexpression (P<0.05, Figure 6A). As Figure 6B shows, cell viability was decreased by HEIH silencing, and the decreased viability was attenuated by CDK8 overexpression or miR-193a-5p downregulation (P<0.05). Both the wound-healing rate and the number of invasion cells were significantly inhibited by HEIH-1 silencing, and miR-193a-5p inhibition or CDK8 overexpression partly reversed the decreased wound-healing rate and the number of invasion cells (P<0.05, Figure 6C,6D). Thus, silencing HEIH impeded cell viability, migration, and invasion in HCC by modulating the miR-193a-5p/CDK8 axis.
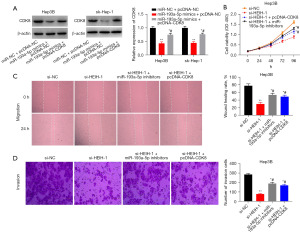
Discussion
The regulatory effects of lncRNA in multiple cancers have been investigated in recent years (30,31). This research showed that HEIH knockdown hampered the viability, migration, and invasion of HCC cells. Additionally, miR-193a-5p was a target molecular of HEIH and directly targeted CDK8. These discoveries will inform further investigations of HCC.
Thousands of lncRNAs have been identified by sequencing human transcriptomes (32). Numerous studies have shown that cancer-related lncRNAs play a crucial role in the initiation and development of HCC (10,33-35). HEIH is a newly identified lncRNA and has been explored in multiple cancers, such as cholangiocarcinoma (36), endometrial cancer (37), and breast cancer (38), in recent years. The current investigation showed that HEIH was significantly upregulated in HCC tissues and cells. Moreover, the increased expression of HEIH was related to an advanced TNM stage and the absence of vascular invasion. These results imply that HEIH could be used to predict the poor prognosis of HCC patients. A recent study also illustrate that HEIH is highly upregulated in HCC tissues (17). Another report revealed that HEIH is significantly overexpressed in HCC tissues and cells, and that silencing HEIH inhibits viability, migration, and invasion in HCC (39). The findings of the present study are consistent with these previous studies. Further, this study showed that the knockdown of HEIH impeded cell viability, migration, and invasion in HCC. The in vivo experiments showed that HEIH silencing repressed HCC tumor growth in mice. All these data suggest that HEIH exerts an oncogenic effect in HCC tumorigenesis.
There is increasing evidence that lncRNAs can function as competing endogenous RNAs (ceRNAs) to sponge miRNAs, thus affecting the biological function of miRNAs (40). LncRNA SNHG7 has been shown to accelerate the progression of HCC via the miR-122-5p/forkhead box K2 (FOXK2) axis (41). Wang et al. show that the downregulation of lncRNA HEIH suppresses the progression of esophageal squamous cell carcinoma via miR-4458/PBX homeobox 3 (PBX3) (42). In HCC, the knockdown of HEIH suppresses cell viability, migration and invasion via miR-199a-3p sponging (17). The present study shows that HEIH acts as a ceRNA to sponge miR-193a-5p.
Previous studies have explored the effects of miR-193a-5p in various cancers. For example, miR-193a-5p is down-regulated in colorectal cancer, and its downregulation is associated with metastasis and poor prognosis (43). MiR-193a-5p is observed to be downregulated in HCC, and the overexpression of miR-193a-5p is shown to restrain HCC cell growth and invasion (28). Consistent with previous studies, this study showed that miR-193a-5p was downregulated in HCC, and its overexpression impeded the viability, migration, and invasion of HCC cells. Further, miR-193a-5p expression was inversely modulated by HEIH in HCC. These findings suggest that HEIH has a pro-tumor role in HCC progression possibly by negatively regulating miR-193a-5p expression.
CDK8 is a transcription factor and has a pro-tumor role in diverse human cancers, and is thus considered a potential drug target for cancer therapy (44). Research has shown that CDK8 and CDK19 are highly expressed in prostate cancer, and their inhibitors can curb the metastasis of prostate cancer (45). In a previous report, a novel CDK8 inhibitor was shown to suppress the metastasis of prostate cancer in vivo and in vitro (46). Additionally, LINC01224 is reported to contribute to the malignant process of gastric cancer via the miR-193a-5p/CDK8 axis (47). Yin et al. report that CDK8 is significantly upregulated in HCC, and directly targets miR-152-3p (48). Similarly, the present study showed that CDK8 expression was strongly enhanced in HCC, and miR-193a-5p directly targeted CDK8. More importantly, it also showed that the inhibitory function of HEIH silencing on the viability, migration, and invasion of HCC cells was partially rescued by miR-193a-5p inhibition or CDK8 overexpression, suggesting that HEIH exerted a pro-tumor effect in the progression of HCC likely by regulating the miR-193a-5p/CDK8 axis.
Conclusions
In summary, the results showed that HEIH was prominently upregulated in HCC, and HEIH expression was closely related to TNM stage and vascular invasion. Additionally, silencing HEIH inhibited the viability, migration, and invasion of HCC cells in vitro, and impeded HCC tumor growth in vivo. The anti-tumor effect of silenced HEIH might be exerted via the targeting of miR-193a-5p and CDK8. More research needs to be conducted to explore the effects of HEIH on other cells related to HCC; however, the current research provides a helpful perspective on interference of HEIH with HCC.
Acknowledgments
Funding: The project was supported by
Footnote
Reporting Checklist: The authors have completed the MDAR and ARRIVE reporting checklists. Available at https://tcr.amegroups.com/article/view/10.21037/tcr-23-2228/rc
Data Sharing Statement: Available at https://tcr.amegroups.com/article/view/10.21037/tcr-23-2228/dss
Peer Review File: Available at https://tcr.amegroups.com/article/view/10.21037/tcr-23-2228/prf
Conflicts of Interest: All authors have completed the ICMJE uniform disclosure form (available at https://tcr.amegroups.com/article/view/10.21037/tcr-23-2228/coif). The authors have no conflicts of interest to declare.
Ethical Statement: The authors are accountable for all aspects of the work in ensuring that questions related to the accuracy or integrity of any part of the work are appropriately investigated and resolved. This study was conducted in accordance with the Declaration of Helsinki (as revised in 2013). The study was approved by the Ethics Committee of The Second Affiliated Hospital of Hainan Medical University (No. LW20210018). Informed consent was taken from all the patients. All animal experiments were approved by the Ethics Committee of the Second Affiliated Hospital of Hainan Medical University (No. LW20210018), in compliance with national guidelines for the care and use of animals.
Open Access Statement: This is an Open Access article distributed in accordance with the Creative Commons Attribution-NonCommercial-NoDerivs 4.0 International License (CC BY-NC-ND 4.0), which permits the non-commercial replication and distribution of the article with the strict proviso that no changes or edits are made and the original work is properly cited (including links to both the formal publication through the relevant DOI and the license). See: https://creativecommons.org/licenses/by-nc-nd/4.0/.
References
- Vogel A, Meyer T, Sapisochin G, et al. Hepatocellular carcinoma. Lancet 2022;400:1345-62. [Crossref] [PubMed]
- Sy AM, Ferreira RD, John BV. Hepatocellular Carcinoma in Primary Biliary Cholangitis. Clin Liver Dis 2022;26:691-704. [Crossref] [PubMed]
- Yang TH, Chan C, Yang PJ, et al. Genetic Susceptibility to Hepatocellular Carcinoma in Patients with Chronic Hepatitis Virus Infection. Viruses 2023;15:559. [Crossref] [PubMed]
- Liccioni A, Reig M, Bruix J. Treatment of hepatocellular carcinoma. Dig Dis 2014;32:554-63. [Crossref] [PubMed]
- Crocetti L, Bargellini I, Cioni R. Loco-regional treatment of HCC: current status. Clin Radiol 2017;72:626-35. [Crossref] [PubMed]
- Cong WM, Wu MC. New insights into molecular diagnostic pathology of primary liver cancer: Advances and challenges. Cancer Lett 2015;368:14-9. [Crossref] [PubMed]
- Quinn JJ, Chang HY. Unique features of long non-coding RNA biogenesis and function. Nat Rev Genet 2016;17:47-62. [Crossref] [PubMed]
- Shao T, Xie Y, Shi J, et al. Surveying lncRNA-lncRNA cooperations reveals dominant effect on tumor immunity cross cancers. Commun Biol 2022;5:1324. [Crossref] [PubMed]
- Shaath H, Vishnubalaji R, Elango R, et al. Long non-coding RNA and RNA-binding protein interactions in cancer: Experimental and machine learning approaches. Semin Cancer Biol 2022;86:325-45. [Crossref] [PubMed]
- Farzaneh M, Ghasemian M, Ghaedrahmati F, et al. Functional roles of lncRNA-TUG1 in hepatocellular carcinoma. Life Sci 2022;308:120974. [Crossref] [PubMed]
- Cai Y, Lyu T, Li H, et al. LncRNA CEBPA-DT promotes liver cancer metastasis through DDR2/β-catenin activation via interacting with hnRNPC. J Exp Clin Cancer Res 2022;41:335. [Crossref] [PubMed]
- Sun JY, Ni MM. Long non-coding RNA HEIH: a novel tumor activator in multiple cancers. Cancer Cell Int 2021;21:558. [Crossref] [PubMed]
- Wang X, Chen Z, Zhou H, et al. LncRNA HEIH expression in cancer prognosis: A review and meta-analysis. Medicine (Baltimore) 2023;102:e33970. [Crossref] [PubMed]
- Zhang H, Shen X, Xiong S, et al. HEIH Promotes Malignant Progression of Gastric Cancer by Regulating STAT3-Mediated Autophagy and Glycolysis. Dis Markers 2022;2022:2634526. [Crossref] [PubMed]
- He C, Huang D, Yang F, et al. High Expression of lncRNA HEIH is Helpful in the Diagnosis of Non-Small Cell Lung Cancer and Predicts Poor Prognosis. Cancer Manag Res 2022;14:503-14. [Crossref] [PubMed]
- Zhao J, Meng R, Yao Q, et al. Long non-coding RNA HEIH promotes breast cancer development via negative modulation of microRNA-200b. Pharmazie 2019;74:471-6. [PubMed]
- Ma Y, Cao D, Li G, et al. Silence of lncRNA HEIH suppressed liver cancer cell growth and metastasis through miR-199a-3p/mTOR axis. J Cell Biochem 2019;120:17757-66. [Crossref] [PubMed]
- Shukla GC, Singh J, Barik S. MicroRNAs: Processing, Maturation, Target Recognition and Regulatory Functions. Mol Cell Pharmacol 2011;3:83-92. [PubMed]
- He L, Hannon GJ. MicroRNAs: small RNAs with a big role in gene regulation. Nat Rev Genet 2004;5:522-31. [Crossref] [PubMed]
- Hayes J, Peruzzi PP, Lawler S. MicroRNAs in cancer: biomarkers, functions and therapy. Trends Mol Med 2014;20:460-9. [Crossref] [PubMed]
- Huang YH, Lian WS, Wang FS, et al. MiR-29a Curbs Hepatocellular Carcinoma Incidence via Targeting of HIF-1α and ANGPT2. Int J Mol Sci 2022;23:1636. [Crossref] [PubMed]
- Yang Y, Yang T, Zhao Z, et al. Down-regulation of BMAL1 by MiR-494-3p Promotes Hepatocellular Carcinoma Growth and Metastasis by Increasing GPAM-mediated Lipid Biosynthesis. Int J Biol Sci 2022;18:6129-44. [Crossref] [PubMed]
- Hong J, Liu J, Zhang Y, et al. MiR-3180 inhibits hepatocellular carcinoma growth and metastasis by targeting lipid synthesis and uptake. Cancer Cell Int 2023;23:66. [Crossref] [PubMed]
- Wang Y, Li N, Zhao J, et al. MiR-193a-5p serves as an inhibitor in ovarian cancer cells through RAB11A. Reprod Toxicol 2022;110:105-12. [Crossref] [PubMed]
- Liu Q, Zhao E, Geng B, et al. Tumor-associated macrophage-derived exosomes transmitting miR-193a-5p promote the progression of renal cell carcinoma via TIMP2-dependent vasculogenic mimicry. Cell Death Dis 2022;13:382. [Crossref] [PubMed]
- Li M, Wu P, Yang Z, et al. miR-193a-5p promotes pancreatic cancer cell metastasis through SRSF6-mediated alternative splicing of OGDHL and ECM1. Am J Cancer Res 2020;10:38-59. [PubMed]
- Zhang X, Zhang D, Bu X, et al. Identification of a novel miRNA-based recurrence and prognosis prediction biomarker for hepatocellular carcinoma. BMC Bioinformatics 2022;23:479. [Crossref] [PubMed]
- Li P, Xiao Z, Luo J, et al. MiR-139-5p, miR-940 and miR-193a-5p inhibit the growth of hepatocellular carcinoma by targeting SPOCK1. J Cell Mol Med 2019;23:2475-88. [Crossref] [PubMed]
- Wang JT, Wang ZH. Role of miR-193a-5p in the proliferation and apoptosis of hepatocellular carcinoma. Eur Rev Med Pharmacol Sci 2018;22:7233-9. [PubMed]
- Li Y, Jiang T, Zhou W, et al. Pan-cancer characterization of immune-related lncRNAs identifies potential oncogenic biomarkers. Nat Commun 2020;11:1000. [Crossref] [PubMed]
- Park EG, Pyo SJ, Cui Y, et al. Tumor immune microenvironment lncRNAs. Brief Bioinform 2022;23:bbab504. [Crossref] [PubMed]
- Liao HT, Huang JW, Lan T, et al. Identification of The Aberrantly Expressed LncRNAs in Hepatocellular Carcinoma: A Bioinformatics Analysis Based on RNA-sequencing. Sci Rep 2018;8:5395. [Crossref] [PubMed]
- Huang Z, Zhou JK, Peng Y, et al. The role of long noncoding RNAs in hepatocellular carcinoma. Mol Cancer 2020;19:77. [Crossref] [PubMed]
- Xu K, Xia P, Gongye X, et al. A novel lncRNA RP11-386G11.10 reprograms lipid metabolism to promote hepatocellular carcinoma progression. Mol Metab 2022;63:101540. [Crossref] [PubMed]
- Li Y, Hu J, Guo D, et al. LncRNA SNHG5 promotes the proliferation and cancer stem cell-like properties of HCC by regulating UPF1 and Wnt-signaling pathway. Cancer Gene Ther 2022;29:1373-83. [Crossref] [PubMed]
- Wan T, Wang H, Gou M, et al. LncRNA HEIH promotes cell proliferation, migration and invasion in cholangiocarcinoma by modulating miR-98-5p/HECTD4. Biomed Pharmacother 2020;125:109916. [Crossref] [PubMed]
- Guo JL, Tang T, Li JH, et al. LncRNA HEIH Enhances Paclitaxel-Tolerance of Endometrial Cancer Cells via Activation of MAPK Signaling Pathway. Pathol Oncol Res 2020;26:1757-66. [Crossref] [PubMed]
- Nafea H, Youness RA, Abou-Aisha K, et al. LncRNA HEIH/miR-939-5p interplay modulates triple-negative breast cancer progression through NOS2-induced nitric oxide production. J Cell Physiol 2021;236:5362-72. [Crossref] [PubMed]
- Shen Q, Jiang S, Wu M, et al. LncRNA HEIH Confers Cell Sorafenib Resistance in Hepatocellular Carcinoma by Regulating miR-98-5p/PI3K/AKT Pathway. Cancer Manag Res 2020;12:6585-95. [Crossref] [PubMed]
- Chan JJ, Tay Y. Noncoding RNA:RNA Regulatory Networks in Cancer. Int J Mol Sci 2018;19:1310. [Crossref] [PubMed]
- Zhao Z, Gao J, Huang S. LncRNA SNHG7 Promotes the HCC Progression Through miR-122-5p/FOXK2 Axis. Dig Dis Sci 2022;67:925-35. [Crossref] [PubMed]
- Wang D, You D, Pan Y, et al. Downregulation of lncRNA-HEIH curbs esophageal squamous cell carcinoma progression by modulating miR-4458/PBX3. Thorac Cancer 2020;11:1963-71. [Crossref] [PubMed]
- Zhang P, Ji DB, Han HB, et al. Downregulation of miR-193a-5p correlates with lymph node metastasis and poor prognosis in colorectal cancer. World J Gastroenterol 2014;20:12241-8. [Crossref] [PubMed]
- Wu D, Zhang Z, Chen X, et al. Angel or Devil ? - CDK8 as the new drug target. Eur J Med Chem 2021;213:113043. [Crossref] [PubMed]
- Offermann A, Joerg V, Becker F, et al. Inhibition of Cyclin-Dependent Kinase 8/Cyclin-Dependent Kinase 19 Suppresses Its Pro-Oncogenic Effects in Prostate Cancer. Am J Pathol 2022;192:813-23. [Crossref] [PubMed]
- Ho TY, Sung TY, Pan SL, et al. The study of a novel CDK8 inhibitor E966-0530-45418 that inhibits prostate cancer metastasis in vitro and in vivo. Biomed Pharmacother 2023;162:114667. [Crossref] [PubMed]
- Sun H, Yan J, Tian G, et al. LINC01224 accelerates malignant transformation via MiR-193a-5p/CDK8 axis in gastric cancer. Cancer Med 2021;10:1377-93. [Crossref] [PubMed]
- Yin T, Liu MM, Jin RT, et al. miR-152-3p Modulates hepatic carcinogenesis by targeting cyclin-dependent kinase 8. Pathol Res Pract 2019;215:152406. [Crossref] [PubMed]