PD-1-induced encephalopathy: a report of 2 cases on neurological toxicities with immune checkpoint inhibitors
Highlight box
Key findings
• Immune checkpoint inhibitors (ICIs) may induce encephalitis.
What is known and what is new?
• ICIs have been applied to an increasing number of cancers but can cause adverse reactions.
• To diagnose programmed cell death protein 1-induced encephalopathy needs to exclude paraneoplastic neurological syndromes.
What is the implication, and what should change now?
• For complications of the nervous system, a diagnosis might be made based on the sufficient collection of disease manifestations combined with imaging and cerebrospinal fluid examinations, especially the detection of paraneoplastic antibodies before treatment.
Introduction
Antigens from cancer cells are readily recognized by the immune system. However, a variety of mechanisms exist that allow these cells to evade detection by the immune system, including the expression of inhibitory ligands for lymphocyte immune checkpoint molecules (1). Under normal physiological conditions, immune checkpoints prevent autoimmunity by maintaining immunologic homeostasis (2). Immune checkpoint inhibitor (ICI) therapies, such as programmed cell death protein 1 (PD-1) blockade therapy and cytotoxic T-lymphocyte-associated antigen-4 (CTLA-4) blockade therapy, have shown promising anti-tumor effects (3). In recent times, there has been the development of antagonistic antibodies targeting CTLA-4 (e.g., ipilimumab) and PD-1 (e.g., pembrolizumab and nivolumab). These antibodies have undergone testing for their effectiveness in prospective clinical trials involving various types of malignancies, including renal-cell carcinoma (4), non-small cell lung cancer (5-7), and metastatic melanoma (8,9). Although ICIs enhance anti-tumor immune reactivity resulting in tumor regression, they also reduce immune tolerance towards self-antigen and predispose to the development of autoimmunity. The side effects caused by ICIs are called immune-related adverse effects (irAEs) (10). Immunotherapy commonly affects the digestive, hepatic, dermatologic, endocrine, and pulmonary systems (11). irAEs may be pronounced and contribute to drug discontinuation, morbidity and mortality (12,13). Contrary to this, Hara and colleagues have reported that patients with upper gastrointestinal cancer who were treated with nivolumab had a better prognosis if they developed irAEs compared to those who did not. It is crucial to continue nivolumab in the long-term by detecting irAEs early and responding to them appropriately (14). In addition, there was also evidence from another study that malignancies with sarcopenia at the time of immunotherapy did not increase the risk of irAEs, and sarcopenia was not associated with irAEs (15).
In the last few years, clinical trials revealed that drugs targeting PD-1/programmed cell death ligand 1 (PD-L1) are superior in efficacy and tolerability to chemotherapy, enhancing disease control, overall survival, and quality of life in both first- and second-line settings (16-19). The anti-PD-1 monoclonal antibody is a humanized immunoglobulin G4 (IgG4) anti-PD-1 antibody that blocks the PD-1/PD-L1 pathway, and enhances the anti-tumor capacities of the host T cells (20,21). However, the initial clinical trials supporting the use of PD-(L)1 inhibitors may have underreported irAEs (22), possibly due to their delayed onset. There is limited information on the occurrence of neurological adverse reactions to ICIs. In prospective trials evaluating anti-CTLA-4 and/or anti-PD-1 antibodies, the incidence of any grade neurological irAE was 3.8% for anti-CTLA-4 antibodies, 6.1% for anti-PD-1 antibodies, and 12.0% for a combination of both. The occurrence of high-grade (i.e., grade 3–4) neurological irAEs appears to be higher in patients treated with anti-CTLA-4 therapy (0.7%) than in those treated with anti-PD-1 therapy (0.4%). Furthermore, trials assessing the combination of both antibodies found a similar incidence of high-grade neurological irAEs as with anti-CTLA-4 therapy alone (0.7%). The most prevalent symptom reported by patients was constitutional fatigue. Fatigue was observed in approximately 20–25% of patients, with grade 3 fatigue reported in 1.1–2.2% of cases. Other symptoms included asthenia (1.7–4.5% of patients) and decreased appetite (8.5–9.8% of patients), both of which were observed in varying grades (11).
irAEs of the central nervous system are often of a high grade. Neurological adverse events resulting from ICIs are rare, especially encephalopathies; however, their recognition is important due to their potential severity (23,24). Notably, it is difficult to differentiate and diagnose encephalopathy caused by immunotherapy and autoimmune encephalitis caused by paraneoplastic syndromes (PNSs), a group of diseases related to malignant tumors. The pathogenesis of PNSs is mediated by immunity. Primary systemic tumors express proteins that are normally expressed only in neurons, and the body’s immune response produces anti-neuronal antibodies, resulting in clinical symptoms. Serum and cerebrospinal fluid (CSF) can both detect these antibodies. These antibodies can be used as biomarkers to identify primary tumors, which is helpful in diagnosing specific types of tumors (25). Cancer patients treated with ICIs, especially those with small cell lung cancer (SCLC), are at risk of immune-mediated PNSs (e.g., melanoma and renal-cell carcinoma). Similarly, anti-CTLA-4 antibody treatment in mice resulted in paraneoplastic cerebellar degeneration on the following day (26). Thus, a PNS could be caused by the treatment itself in the case of SCLC patients undergoing an ICI.
In the near future, ICIs will be used by a progressively greater number of patients due to their undisputed clinical efficacy. However, currently, there is limited information available on the incidence, characteristics, and outcomes of neurologic irAEs associated with ICI treatment. Most of this information has been obtained from isolated case reports or basic safety reports conducted during clinical trials. Consequently, there is significant variation in the available information regarding the diagnosis and treatment approaches. Moreover, it is important to consider the impact of case selection and reporting bias. In this article, we report 2 cases of patients with PD-1-induced encephalopathy to extend understandings of the nature, timing, and management of the complication. We present this article in accordance with the CARE reporting checklist (available at https://tcr.amegroups.com/article/view/10.21037/tcr-23-2043/rc).
Case presentation
All procedures performed in this study were in accordance with the ethical standards of the institutional and/or national research committee(s) and with the Declaration of Helsinki (as revised in 2013). Written informed consent was obtained from the patients for publication of this case report and accompanying images. A copy of the written consent is available for review by the editorial office of this journal. The 2 cases reported in this article provided by Guangdong Sanjiu Brain Hospital from 2019–2020.
Case 1
In March 2020, an olfactory neuroblastoma in a 24-year-old man was diagnosed by pathology. His initial complaints were nasal obstruction and a loss of smell in January 2020. Epstein-Barr virus (EBV)-encoded small RNAs (EBERs) was negative, and the stage was C (Figure 1). Magnetic resonance imaging (MRI) on February 26, 2020, showed a bilateral nasal space-occupying lesion of approximately 7.8 cm × 5.8 cm × 7.7 cm, involving the surrounding tissue (Figure 2A).
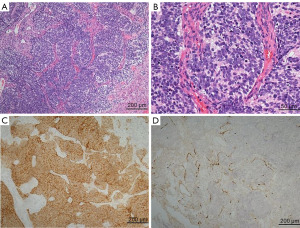
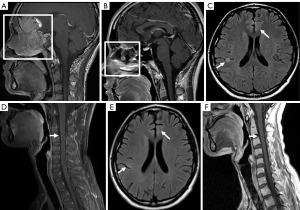
On March 9, 2020, the patient was treated with etoposide (100 mg/m2, d1–3) and cisplatin (25 mg/m2, d1–3; Q21d) and then received the anti-PD-1 monoclonal antibody tislelizumab (200 mg) every 3 weeks. After 4 cycles of treatment, he developed hand-and-foot paralysis that improved through symptomatic treatment (details unknown).
On June 24, 2020, grand mal epilepsy occurred during the 6th course of application of tislelizumab and the treatment was terminated. The epilepsy was controlled with oxcarbazepine (0.3 g, bid) and sodium valproate (500 mg, bid). An MRI re-examination on July 2, 2020 showed that the primary tumor had shrunk (Figure 2B) and multiple abnormal signals in the right parietal sulcus and the left frontal lobe (Figure 2C). Linear enhancement of spinal cord membranes observed on T1-enhanced, fat-suppressed MRI imaging (Figure 2D).
The patient received local radiotherapy on July 30, 2020. The prescription doses were gross tumor volume (GTV; 68.10 Gy/30 f), clinical target volume (CTV1: 60 Gy/30 f, and CTV2: 54 Gy/30 f). After 6 rounds of radiotherapy, grand mal epilepsy, which lasted for 1 min, occurred again.
The patient was diagnosed with anti-PD-1 monoclonal antibody-induced autoimmune encephalopathy. His symptoms were alleviated by high doses of glucocorticoid and immunosorbent therapy on August 18 and August 20, 2020.
MRI review showed that the intracranial and spinal enhancement lesions had largely disappeared in axial T2 FLAIR images (Figure 2E) and sagittal T1 enhancement images (Figure 2F). Serum paraneoplastic antibody anti-Hu (Table 1: from Guangzhou Goldfield Diagnostic Laboratory) was mildly positive. The CSF was strongly positive for neuronal antibodies, which were considered to be anti-Hu. Radiotherapy was continued without further seizures. The patient’s diagnosis and treatment schedule are shown in Figure 3.
Table 1
Antibody | Test method | Result | Reference interval |
---|---|---|---|
Anti-GAD65 | BLOT | Negative (–) | Negative (–) |
Anti-Zic4 | BLOT | Negative (–) | Negative (–) |
Anti-Tr (DNER) | BLOT | Negative (–) | Negative (–) |
Anti-SOX1 | BLOT | Negative (–) | Negative (–) |
Anti-Ma2 | BLOT | Negative (–) | Negative (–) |
Anti-Ma1 | BLOT | Negative (–) | Negative (–) |
Anti-Amphiphysin | BLOT | Negative (–) | Negative (–) |
Anti-CV2 | BLOT | Negative (–) | Negative (–) |
Anti-Ri | BLOT | Negative (–) | Negative (–) |
Anti-Yo | BLOT | Negative (–) | Negative (–) |
Anti-Hu | BLOT | Positive (+) | Negative (–) |
BLOT, blotting method.
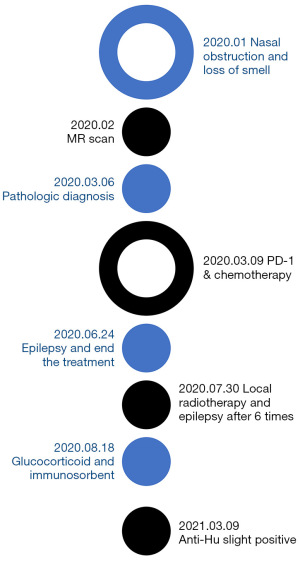
Case 2
A 67-year-old female was diagnosed with NSCLC and multiple bone metastases on July 29th, 2019. The clinical stage of her tumor was IVB. Large cell adenocarcinoma was confirmed by pathologic examination. Tissue PCR was positive for the L858R mutation in exon 21 of the epidermal growth factor receptor (EGFR) (Figure 4). Brain MRI showed negative imaging findings on T1-weighted, gadolinium-enhanced sequences (Figure 5A).
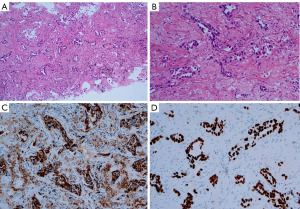
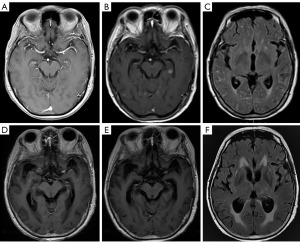
Once diagnosed with EGFR exon21 L858R mutation, the patient received afatinib (40 mg, qd) for 12 months. In August 2020, she developed symptoms of headache and double vision. Chest computed tomography (CT) showed that the lung lesions were larger than before, brain MRI showed multiple intracranial lesions (Figure 5B,5C), and her CSF cytologic examination results were positive. She was diagnosed with meningeal metastasis of lung adenocarcinoma.
The blood genetic tests did not suggest driver gene mutations. Her therapy was changed to pemetrexed (500 mg/m2, d1), bevacizumab (7.5 mg/kg, d1), and carilizumab (200 mg, d1; Q21d). These treatments were recommended by the guidelines for NSCLC. However, her symptoms did not improve significantly. So these treatments had not continued. She received whole brain radiotherapy (WBRT) with a dose of 40 Gy/20 f plus temozolomide (75 mg/m2) on September 17, 2020. After 10 rounds of radiotherapy, her symptoms of headache and double vision were alleviated. However, her cognitive function gradually decreased after WBRT.
A brain MRI re-examination showed that the lesions had shrunk (Figure 5D,5E). Her lumbar puncture results indicated normal opening pressures (130 mmH2O), which did not support the progression of meningeal metastases. On October 15, 2020, she started to use bevacizumab (5 mg/kg d1; Q21d), and her cognitive function improved. On October 20, 2020, she received pemetrexed (500 mg/m2) after folic acid pre-treatment. The process was smooth. On October 22, 2020, she was treated with 500 mg of durvalumab. Durvalumab was approved by FDA for maintenance treatment of NSCLC. But on October 23, 2020, her headache and cognitive function were worse than before. The treatment had not continued.
A brain MRI re-examination on October 26, 2020, revealed aggravation of cerebral oedema, and dehydration was ineffective (Figure 5F). The patient had a history of hypothyroidism and thyroxin replacement. During the PD-1 treatment, her thyroid function continued to decline, and her serum cortisol level gradually decreased. On October 30, 2020, cortisol at 23.5 µg/L was detected (Table 2). After prednisone (5 mg qd) was administered, the patient developed psycho-behavioral abnormalities, including suspicion and a delusion of being killed. Her cognitive function was tested by Montreal Cognitive Assessment (MoCA) (Table 3). She developed a fever on November 16, 2020, with a temperature of 38.5 ℃. Chest CT showed diffuse exudation in both lungs. Combined with her medical history, immune pneumonia was not ruled out. The patient was treated with methylprednisolone (40 mg), after which her temperature returned to normal, but her mental symptoms did not improve significantly. The patient’s diagnosis and treatment timeline are illustrated in Figure 6.
Table 2
Test | Ref. | Sep 11, 2020 | Oct 15, 2020 | Oct 30, 2020 | Nov 05, 2020 |
---|---|---|---|---|---|
FT4, pmol/L | 12–22 | 14.1 | 14.1 | 10.1 | 12 |
ACTH, ng/L | 7.2–63.3 | 8.2 | 2.9 | 1.8 | 2.1 |
Cortisol, µg/L | 62–194 | 63.4 | 13.6 | 23.5 | 10.7 |
Total thyroxine, nmol/L | 66–181 | 88.3 | 78.2 | 58.1 | 53.2 |
FT3, pmol/L | 3.1–6.8 | 2.17 | 1.52 | 1.43 | 1.32 |
TSH, mIU/L | 0.27–4.2 | 5.26 | 0.57 | 0.72 | 0.3 |
FT4, free thyroxine; ACTH, adrenocorticotropic hormone; FT3, free triiodothyronine; TSH, thyroid stimulating hormone.
Table 3
Item | Score of Sep 16, 2020 | Score of Nov 05, 2020 |
---|---|---|
MoCA | 18 | 4 |
Immediate memory | 4 | 1 |
Delayed memory | 3 | 2 |
Language fluency | 24 | 2 |
Digital conversion | 30 | 0 |
MoCA, Montreal Cognitive Assessment.
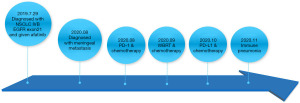
Discussion
In the above 2 cases, epilepsy, cognitive decline, and mental behavioral abnormalities occurred during PD-1 immunotherapy. The symptoms of encephalitis caused by ICIs are diverse and atypical, making diagnosis challenging. Thus, we sought to examine the diagnosis and treatment approaches. PD-1-induced encephalopathy requires timely differential diagnosis and treatment to effectively prevent tumor progression and prolong patient survival.
Immunotherapy showed good efficacy for solid tumors, and the commonly used efficacy predictors included PD-L1 and tumor mutation burden (TMB) (27). Patients with various cancers have greatly benefited from the use of ICIs, such as PD-1/PD-L1 inhibitors (28). The diagnostic assessment of response to immunotherapy was performed with response evaluation criteria in solid tumors (RECIST) 1.1 criteria (29). Based on a recent study conducted by Larkin et al., it has been found that immune-mediated encephalitis typically manifests around 55 days (with a range of 18–297 days) after undergoing ICI treatment (13). The occurrence of immune-mediated encephalitis in patients treated with PD-1 is estimated to be approximately 0.2%, and it includes various forms such as limbic encephalitis (LE), brainstem encephalitis, and necrotizing encephalitis (30-32). The main symptoms of immune-mediated encephalitis are headache, fever, insanity, memory impairment, sleepiness, hallucinations, seizures, neck strength, decreased mental state, impaired attention, and disorientation (33,34). However, these symptoms lack specificity. Cases of encephalitis caused by PD-1 need to be distinguished from infectious diseases, metabolic diseases, brain metastases, cerebrovascular diseases and meningeal metastases, especially LE in PNSs. When relevant symptoms are present, brain MRI and CSF examinations are required (35). MRI can reveal the limited diffusion of the limbic system (32). Additionally, the T2 FLAIR sequence can show a symmetrical high signal in the bilateral thalamus in some cases (36). MRI T1-sequence enhancement scans showed linear enhancement of both dura and floppy meninges in PD-1-induced encephalopathy (32,37). The CSF analysis results were abnormal in both two reported cases. The routine examinations of CSF showed an increase in cells and protein abundance (38). An increase in cell number is mainly due to lymphocytosis but may also involve neutrophilia. The appearance of neutrophils often suggests a necrotic process in the lesion. In addition, the increase in CSF IgG suggests that ICIs have an effect on B cell function and differentiation (39). However, when an autoimmune neurologic condition is suspected, practice involves testing of both serum and CSF with autoantibody panels. Importantly, newly identified neural autoantibodies are not included on paraneoplastic panels, such as IgLON5, a neural cell adhesion molecule (40). Antibodies against N-methyl-D-aspartate receptors, which are associated with autoimmune encephalitis, should be tested (41).
Notably, pathological findings are lacking in most cases. Following the elimination of bacterial or viral infections, high-dose steroids need to be applied (35). In practice, treatment is carried out according to the severity of the disease. Classification and management of ICI related encephalitis were shown in Table 4 (42). A number of paraneoplastic antibodies have been reported in cases of encephalitis, including antibodies to N-methyl-D-aspartate receptors and contactin-associated protein-like 2 or anti-Hu antibodies (34). These paraneoplastic-associated antibodies often cause LE. It has been shown that LE usually refers to a tumor anti-neuron antibody (such as anti-Hu) and cell surface antibody [alpha-amino-3-hydroxy-5-methyl-4-isoxazole propionic antibody (AMPAR)] (43). The clinical features of LE are short-term memory loss, seizures, irritability, depression, and cognitive decline in subacute onset. When anti-Hu is positive in SCLC patients, LE is rarely isolated and is usually complicated by diffuse and multifocal encephalomyelitis. Such encephalitis is mediated by cytotoxic T cells and thus has a poor response to treatment. Approximately 30% of LE cases positive for anti-Ma2 can be improved by tumor targeted therapy and immunotherapy (44). AMPAR-positive LE has obvious mental symptoms, of which 64% are related to paraneoplastic tumors (45). Anti-GABA-B-associated LE is usually accompanied by severe seizures in the early stage, approximately half of which are associated with paraneoplastic tumors (46).
Table 4
Severity | Representation | Management |
---|---|---|
G1 | Mild symptoms not limiting daily life | ICI treatment be maintained and the diagnostic process started. If there is no improvement or symptom deterioration, ICIs should be stopped permanently |
G2 | New moderate or severe neurological signs or symptoms | ICI treatment can be maintained temporarily, but symptoms and signs should be closely monitored. Antiviral drugs or antibiotics may empirically use as prevention |
G3–G4 | Severe limitations in daily life activities or life threatening or encephalitis with psycho-behavioral abnormalities | ICIs should be permanently stopped, and high-dose glucocorticoids (0.5–1 mg/kg/d) should be given if there is no infection |
ICI, immune checkpoint inhibitor.
In case 1, while epilepsy occurred after PD-1 treatment and was consistent with the time point of immunotherapy-mediated encephalitis. So, this case diagnosed PD-1-induced encephalitis. In some cases, PNSs may be associated with the initiation of the ICIs. It was reported that ICIs could induce both humoral and cell-mediated paraneoplastic neurologic syndromes (47). According to Müller-Jensen et al., there is an association between neuronal autoantibody profiles in 73 cancer patients, both with and without ICI-induced neurological immune adverse events. In their study, they found that ICI treatment could induce brain-reactive autoantibodies; however, these autoantibodies weren’t necessarily associated with neurological symptoms. In conclusion, patients with and without neurologically irAEs are both likely to develop brain-reactive autoantibodies after receiving ICIs (48). Therefore, despite the patient in case 1 subsequently showing a positive result for anti-Hu, an antibody associated with PNS, we established a conclusive diagnosis of encephalitis induced by PD-1. The limitation of the diagnostic and therapeutic process in Case 1 was that paraneoplastic-associated antibodies were not detected prior to immunotherapy, and IgG in the CSF was not further evaluated. Case 2 involved a patient diagnosed with NSCLC and meningeal metastasis. After PD-1 treatment, the patient presented with obvious mental symptoms and cognitive impairment. Yshii and the colleagues have shown that patients with lung cancer treated with ICIs are at higher risk for PNSs (26). Evidence has additionally demonstrated that endocrine irAEs are a prevailing complication of ICI treatment. It is imperative to attentively monitor endocrine function alongside ICI administration to promptly identify and manage such adverse events. Nonetheless, the exact mechanisms responsible for endocrine irAEs remain ambiguous and presumably entail a multifaceted interaction between T-cell activation and the regeneration of glands, which might be associated with inherent organ-specific autoimmunity (49). The limitation of Case 2 was that paraneoplasm-associated antibodies were not tested before or after immunotherapy, nor was IgG in the CSF tested. Immunotherapy for lung cancer often leads to abnormal thyroid function (50). In this case, thyroid function and cortisol decreased continuously, which can also lead to a decline in mental symptoms and cognitive function. High-dose hormone, gamma globulin and immunosorbent therapy were not used. However, there were also irregularities in the patient’s treatment. Thus, PD-1-induced encephalopathy could not be clearly diagnosed. Furthermore, if the above treatments did not improve, encephalopathic manifestations due to tumor progression should be considered rather than PD-1 encephalopathy.
Timely diagnosis and treatment are crucial, as PD-1-induced encephalopathy can lead to serious sequelae or death. However, stopping ICI treatment may reduce the therapeutic efficiency of drugs. Severe encephalopathy caused by PD-1 may also shorten the survival time of patients. Thus, a multidisciplinary team should participate in the management decisions for patients treated with PD-1 immunotherapy.
Conclusions
While ICIs have been increasingly used in cancer treatment, they can also lead to irAEs. This paragraph discusses two cases that provide detailed information about the clinical manifestations, differential diagnosis, treatment process of PD-1-induced encephalopathy. To diagnose PD-1-induced encephalopathy, it is important to rule out PNSs. However, it is worth noting that PNSs often occur during immunotherapy. Therefore, it is necessary to promptly test for PNS antibodies in both serum fluid and CSF.
Acknowledgments
Funding: This work was supported by
Footnote
Reporting Checklist: The authors have completed the CARE reporting checklist. Available at https://tcr.amegroups.com/article/view/10.21037/tcr-23-2043/rc
Peer Review File: Available at https://tcr.amegroups.com/article/view/10.21037/tcr-23-2043/prf
Conflicts of Interest: All authors have completed the ICMJE uniform disclosure form (available at https://tcr.amegroups.com/article/view/10.21037/tcr-23-2043/coif). A.R. reports stock options by IQVIA Holdings Inc. The other authors have no conflicts of interest to declare.
Ethical Statement: The authors are accountable for all aspects of the work in ensuring that questions related to the accuracy or integrity of any part of the work are appropriately investigated and resolved. All procedures performed in this study were in accordance with the ethical standards of the institutional and/or national research committee(s) and with the Declaration of Helsinki (as revised in 2013). Written informed consent was obtained from the patients for publication of this case report and accompanying images. A copy of the written consent is available for review by the editorial office of this journal.
Open Access Statement: This is an Open Access article distributed in accordance with the Creative Commons Attribution-NonCommercial-NoDerivs 4.0 International License (CC BY-NC-ND 4.0), which permits the non-commercial replication and distribution of the article with the strict proviso that no changes or edits are made and the original work is properly cited (including links to both the formal publication through the relevant DOI and the license). See: https://creativecommons.org/licenses/by-nc-nd/4.0/.
References
- Pardoll DM. The blockade of immune checkpoints in cancer immunotherapy. Nat Rev Cancer 2012;12:252-64. [Crossref] [PubMed]
- Zou W, Chen L. Inhibitory B7-family molecules in the tumour microenvironment. Nat Rev Immunol 2008;8:467-77. [Crossref] [PubMed]
- Friedman CF, Proverbs-Singh TA, Postow MA. Treatment of the Immune-Related Adverse Effects of Immune Checkpoint Inhibitors: A Review. JAMA Oncol 2016;2:1346-53. [Crossref] [PubMed]
- Motzer RJ, Escudier B, McDermott DF, et al. Nivolumab versus Everolimus in Advanced Renal-Cell Carcinoma. N Engl J Med 2015;373:1803-13. [Crossref] [PubMed]
- Borghaei H, Paz-Ares L, Horn L, et al. Nivolumab versus Docetaxel in Advanced Nonsquamous Non-Small-Cell Lung Cancer. N Engl J Med 2015;373:1627-39. [Crossref] [PubMed]
- Garon EB, Rizvi NA, Hui R, et al. Pembrolizumab for the treatment of non-small-cell lung cancer. N Engl J Med 2015;372:2018-28. [Crossref] [PubMed]
- Rizvi NA, Mazières J, Planchard D, et al. Activity and safety of nivolumab, an anti-PD-1 immune checkpoint inhibitor, for patients with advanced, refractory squamous non-small-cell lung cancer (CheckMate 063): a phase 2, single-arm trial. Lancet Oncol 2015;16:257-65. [Crossref] [PubMed]
- Eggermont AM, Chiarion-Sileni V, Grob JJ, et al. Adjuvant ipilimumab versus placebo after complete resection of high-risk stage III melanoma (EORTC 18071): a randomised, double-blind, phase 3 trial. Lancet Oncol 2015;16:522-30. [Crossref] [PubMed]
- Weber JS, D'Angelo SP, Minor D, et al. Nivolumab versus chemotherapy in patients with advanced melanoma who progressed after anti-CTLA-4 treatment (CheckMate 037): a randomised, controlled, open-label, phase 3 trial. Lancet Oncol 2015;16:375-84. [Crossref] [PubMed]
- Shohdy KS, Abdel-Rahman O. Neurological complications of anti-PD-1 antibodies: shall we be more concerned? Transl Cancer Res 2018;7:S436-8. [Crossref]
- Cuzzubbo S, Javeri F, Tissier M, et al. Neurological adverse events associated with immune checkpoint inhibitors: Review of the literature. Eur J Cancer 2017;73:1-8. [Crossref] [PubMed]
- Rapoport BL, van Eeden R, Sibaud V, et al. Supportive care for patients undergoing immunotherapy. Support Care Cancer 2017;25:3017-30. [Crossref] [PubMed]
- Larkin J, Chiarion-Sileni V, Gonzalez R, et al. Combined Nivolumab and Ipilimumab or Monotherapy in Untreated Melanoma. N Engl J Med 2015;373:23-34. Erratum in: N Engl J Med 2018;379:2185. [Crossref] [PubMed]
- Hara Y, Baba Y, Toihata T, et al. Immune-related adverse events and prognosis in patients with upper gastrointestinal cancer treated with nivolumab. J Gastrointest Oncol 2022;13:2779-88. [Crossref] [PubMed]
- Li S, Wang T, Lai W, et al. Prognostic impact of sarcopenia on immune-related adverse events in malignancies received immune checkpoint inhibitors: a systematic review and meta-analysis. Transl Cancer Res 2021;10:5150-8. [Crossref] [PubMed]
- Siegel RL, Miller KD, Jemal A. Cancer statistics, 2018. CA Cancer J Clin 2018;68:7-30. [Crossref] [PubMed]
- Herbst RS, Baas P, Kim DW, et al. Pembrolizumab versus docetaxel for previously treated, PD-L1-positive, advanced non-small-cell lung cancer (KEYNOTE-010): a randomised controlled trial. Lancet 2016;387:1540-50. [Crossref] [PubMed]
- Rittmeyer A, Barlesi F, Waterkamp D, et al. Atezolizumab versus docetaxel in patients with previously treated non-small-cell lung cancer (OAK): a phase 3, open-label, multicentre randomised controlled trial. Lancet 2017;389:255-65. Erratum in: Lancet 2017;389:e5. [Crossref] [PubMed]
- Brahmer JR, Rodríguez-Abreu D, Robinson AG, et al. Health-related quality-of-life results for pembrolizumab versus chemotherapy in advanced, PD-L1-positive NSCLC (KEYNOTE-024): a multicentre, international, randomised, open-label phase 3 trial. Lancet Oncol 2017;18:1600-9. [Crossref] [PubMed]
- Qin S, Finn RS, Kudo M, et al. RATIONALE 301 study: tislelizumab versus sorafenib as first-line treatment for unresectable hepatocellular carcinoma. Future Oncol 2019;15:1811-22. [Crossref] [PubMed]
- Li W, Wang H, Chen B, et al. Anti PD-1 monoclonal antibody induced autoimmune diabetes mellitus: a case report and brief review. Transl Lung Cancer Res 2020;9:379-88. [Crossref] [PubMed]
- Chen TW, Razak AR, Bedard PL, et al. A systematic review of immune-related adverse event reporting in clinical trials of immune checkpoint inhibitors. Ann Oncol 2015;26:1824-9. [Crossref] [PubMed]
- Zimmer L, Goldinger SM, Hofmann L, et al. Neurological, respiratory, musculoskeletal, cardiac and ocular side-effects of anti-PD-1 therapy. Eur J Cancer 2016;60:210-25. [Crossref] [PubMed]
- Kao JC, Liao B, Markovic SN, et al. Neurological Complications Associated With Anti-Programmed Death 1 (PD-1) Antibodies. JAMA Neurol 2017;74:1216-22. [Crossref] [PubMed]
- van Broekhoven F, de Graaf MT, Bromberg JE, et al. Human chorionic gonadotropin treatment of anti-Hu-associated paraneoplastic neurological syndromes. J Neurol Neurosurg Psychiatry 2010;81:1341-4. [Crossref] [PubMed]
- Yshii LM, Gebauer CM, Pignolet B, et al. CTLA4 blockade elicits paraneoplastic neurological disease in a mouse model. Brain 2016;139:2923-34. [Crossref] [PubMed]
- Xue J, Song Y, Xu W, et al. The CDK1-Related lncRNA and CXCL8 Mediated Immune Resistance in Lung Adenocarcinoma. Cells 2022;11:2688. [Crossref] [PubMed]
- Wang Z, Zhang H, Zhai Y, et al. Single-Cell Profiling Reveals Heterogeneity of Primary and Lymph Node Metastatic Tumors and Immune Cell Populations and Discovers Important Prognostic Significance of CCDC43 in Oral Squamous Cell Carcinoma. Front Immunol 2022;13:843322. [Crossref] [PubMed]
- Polverari G, Ceci F, Bertaglia V, et al. (18)F-FDG Pet Parameters and Radiomics Features Analysis in Advanced Nsclc Treated with Immunotherapy as Predictors of Therapy Response and Survival. Cancers (Basel) 2020;12:1163. [Crossref] [PubMed]
- Bossart S, Thurneysen S, Rushing E, et al. Case Report: Encephalitis, with Brainstem Involvement, Following Checkpoint Inhibitor Therapy in Metastatic Melanoma. Oncologist 2017;22:749-53. [Crossref] [PubMed]
- Leitinger M, Varosanec MV, Pikija S, et al. Fatal Necrotizing Encephalopathy after Treatment with Nivolumab for Squamous Non-Small Cell Lung Cancer: Case Report and Review of the Literature. Front Immunol 2018;9:108. [Crossref] [PubMed]
- Salam S, Lavin T, Turan A. Limbic encephalitis following immunotherapy against metastatic malignant melanoma. BMJ Case Rep 2016;2016:bcr2016215012. [Crossref] [PubMed]
- Hottinger AF. Neurologic complications of immune checkpoint inhibitors. Curr Opin Neurol 2016;29:806-12. [Crossref] [PubMed]
- Williams TJ, Benavides DR, Patrice KA, et al. Association of Autoimmune Encephalitis With Combined Immune Checkpoint Inhibitor Treatment for Metastatic Cancer. JAMA Neurol 2016;73:928-33. [Crossref] [PubMed]
- Astaras C, de Micheli R, Moura B, et al. Neurological Adverse Events Associated with Immune Checkpoint Inhibitors: Diagnosis and Management. Curr Neurol Neurosci Rep 2018;18:3. [Crossref] [PubMed]
- Oyanguren B, Sánchez V, González FJ, et al. Limbic encephalitis: a clinical-radiological comparison between herpetic and autoimmune etiologies. Eur J Neurol 2013;20:1566-70. [Crossref] [PubMed]
- Mandel JJ, Olar A, Aldape KD, et al. Lambrolizumab induced central nervous system (CNS) toxicity. J Neurol Sci 2014;344:229-31. [Crossref] [PubMed]
- Faje A. Immunotherapy and hypophysitis: clinical presentation, treatment, and biologic insights. Pituitary 2016;19:82-92. [Crossref] [PubMed]
- Okazaki T, Chikuma S, Iwai Y, et al. A rheostat for immune responses: the unique properties of PD-1 and their advantages for clinical application. Nat Immunol 2013;14:1212-8. [Crossref] [PubMed]
- Landa J, Gaig C, Plagumà J, et al. Effects of IgLON5 Antibodies on Neuronal Cytoskeleton: A Link between Autoimmunity and Neurodegeneration. Ann Neurol 2020;88:1023-7. [Crossref] [PubMed]
- Gresa-Arribas N, Titulaer MJ, Torrents A, et al. Antibody titres at diagnosis and during follow-up of anti-NMDA receptor encephalitis: a retrospective study. Lancet Neurol 2014;13:167-77. [Crossref] [PubMed]
- Shi J, Niu J, Shen D, et al. Clinical Diagnosis and Treatment Recommendations for Adverse Reaction in the Nervous System Related to Immunocheckpoint Inhibitor. Zhongguo Fei Ai Za Zhi 2019;22:633-8. [PubMed]
- Shirafuji T, Kanda F, Sekiguchi K, et al. Anti-Hu-associated paraneoplastic encephalomyelitis with esophageal small cell carcinoma. Intern Med 2012;51:2423-7. [Crossref] [PubMed]
- Dalmau J, Graus F, Villarejo A, et al. Clinical analysis of anti-Ma2-associated encephalitis. Brain 2004;127:1831-44. [Crossref] [PubMed]
- Nosadini M, Mohammad SS, Ramanathan S, et al. Immune therapy in autoimmune encephalitis: a systematic review. Expert Rev Neurother 2015;15:1391-419. [Crossref] [PubMed]
- Höftberger R, Titulaer MJ, Sabater L, et al. Encephalitis and GABAB receptor antibodies: novel findings in a new case series of 20 patients. Neurology 2013;81:1500-6. [Crossref] [PubMed]
- Graus F, Dalmau J. Paraneoplastic neurological syndromes in the era of immune-checkpoint inhibitors. Nat Rev Clin Oncol 2019;16:535-48. [Crossref] [PubMed]
- Müller-Jensen L, Knauss S, Ginesta Roque L, et al. Autoantibody profiles in patients with immune checkpoint inhibitor-induced neurological immune related adverse events. Front Immunol 2023;14:1108116. [Crossref] [PubMed]
- Rengarajan M, Gainor JF. Endocrine immune-related adverse events: a double-edged sword? Transl Lung Cancer Res 2021;10:13-7. [Crossref] [PubMed]
- Kazanietz V, Cayol F, Cerini M, et al. 1066P Thyroid toxicity as predictor of response to immunotherapy in lung cancer. Ann Oncol 2020;31:S725. [Crossref]
(English Language Editor: L. Huleatt)