Architecture of head and neck squamous cell carcinoma tumor microenvironment revealed: can tertiary lymphoid structures predict post-surgery recurrence?
Over the past decade, a technology-driven examination of cancer etiology, progression and treatment has shed new light on the molecular nature of the relationship between tumor cells and the tumor microenvironment (TME). Indeed, the explosive growth in large datasets generated from high-throughput techniques such as single-cell RNA-sequencing (scRNA-seq) and spatial transcriptomics has revealed tumor heterogeneity as a leading cause of drug resistance. Moreover, it has offered strategies to enhance anti-tumor immunity and predict patient response to therapy based on immune landscape characteristics. Spurred by such studies, antibody-based immunotherapies aimed at boosting the host’s immune response against tumors (i.e., immune-checkpoint blockade), has become a major weapon in fighting cancer. However, low response rate is a persistent challenge of immune checkpoint blockade therapy in most cancers (1). This underscores the continued need for molecular and multi-omics based investigations into the TME and how it modulates tumor response to therapy, particularly in solid tumors such as head and neck squamous cell carcinoma (HNSCC), for which targeted therapy options are limited.
HNSCC ranks as the sixth most prevalent cancer globally, with an estimated annual incidence of around 890,000 cases and an estimated 50% mortality rate (2). HNSCC arises from epithelial cells lining the oral/nasal cavity, paranasal sinuses, larynx, or pharynx and is associated with risk factors such as tobacco, alcohol consumption and infection with human papillomavirus (HPV). Standard of care treatment for localized HNSCC includes surgery with adjuvant chemoradiation whereas recurrent and/or metastatic disease is targeted in a multidisciplinary manner with concomitant chemotherapy, targeted therapies, or immunotherapy (3). The recent approval of immune checkpoint inhibitors (ICIs) as a first-line treatment of patients with advanced HNSCC has shown some success, however, the heterogeneity and molecular complexity of this disease makes treatment failure a recurrent battle (4). This reinforces the need for novel predictive biomarkers to identify patients who might benefit from targeted immunotherapies. The recent study by Weed et al. takes a step in that direction by using single cell techniques to map out the HNSCC immunological landscape that may predict recurrence in patients following salvage surgery (5).
Weed et al. present their findings of an explorative, prospective clinical study in which the structures and cellular composition of the TME in tumors from HNSCC patients are investigated. Nine HPV-negative tumors were surgically resected from patients with tumor-node-metastasis (TNM) stage II, III, and IV HNSCC, with seven of these tumors being derived from the oral cavity. Five of these patients had a prior smoking history and/or previous radiation therapy while all patients enrolled were naïve to drug interventions. For six patients, this was not a first tumor event, and for those with new recurrences following surgery (half of the cohort), recurrence occurred within a year post-surgery (range, 0.35–0.92 years). The authors performed co-detection by indexing (CODEX) multiparametric imaging of these patient HNSCC tumor samples, a technique that allowed them to conduct multiplexed evaluation of 30 validated antibodies against marker proteins for the diverse cell populations in the TME. This, in turn led to the identification of 20 different cell types organized into 11 domains that were termed “cellular neighborhoods” (CN).
For their analysis, the authors defined the cells primarily by their immune signatures, whereas CNs were characterized by cellular composition and cell-cell contacts. Two CNs resembling tertiary lymphoid structures (TLSs) were identified, TLS1 and TLS2, with opposing immunomodulatory functions. The TLS2 and cold tumor CNs, marked by M2-like macrophages and regulatory T cells (Tregs), and neoplastic cells respectively, were two of the most prevalent CNs followed by the stromal CN. Additionally, the peritumoral CN, a domain characterized by capsule cells, was also prevalent in four out of five patients. Notably, in such tumors, capsule cells were observed encapsulating the clusters of neoplastic cells, forming an orderly barrier that delineated the boundary between the tumor and infiltrating leukocytes (Figure 1). Furthermore, these capsule cells were positive for PDL1 expression, suggesting they may suppress immune responses. It is tempting to suggest that these cells, given their proximity to tumor cells, might be a subset of cancer-associated fibroblasts (CAFs). This notion however is perplexing, given that CAFs are typically the most abundant cellular component within the stromal compartment unlike capsule cells (6). Furthermore, CAFs are associated with tumor stage and perineural invasion but no such correlations could be made in this study (7). If indeed these capsule cells represent tumor-enhancing CAFs reminiscent of the inflammatory CAFs identified in breast, pancreatic, and HNSCC cancers, their scarcity is rather unexpected (8-10). Future investigation of this cell type is thus clearly warranted.
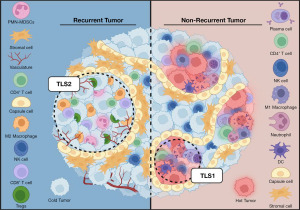
In this work, Weed et al. also fascinatingly showed that the organization of the TME is characterized by a hierarchal structure, with the cold tumor CN being at the center. Separating this domain from other CNs, are the peritumoral and hot tumor CNs, with polymorphonuclear myeloid-derived suppressor cell (PMN-MDSC)-rich and stroma CNs occupying the space between these nests. The natural kill (NK)-rich CN was found to be located distantly from the hot and cold tumor CNs while the neutrophil-rich CN interacted with the tumoral CNs. Not surprisingly, the frequency of the NK and cold tumor CNs were negatively correlated, suggesting neoplastic cells may hamper the expansion of the NK cell population, which are known for their antitumor immune responses. It has been shown that HNSCC tumor cells present surface ligands that inhibit the antitumor activity of NK cells (11). Consequently, their remote positioning from the neoplastic clusters, despite their role as a primary defense against cancer, hints at the presence of an immunosuppressive TME that might hinder the recruitment of NK cells.
The TLS1 CN, comprised of organized infiltrating immune cells, was negatively associated with the stroma CNs (a frequent domain composed mainly of CD90+ mesenchymal stem-like stromal cells). Indeed, others have shown that HNSCC tumors exhibit an increase in CD90+ stromal cells and that this cell population suppresses the infiltration of activated T lymphocytes (12). The stroma CN was also positively correlated with the peritumoral CN, though both cell populations exhibited similar marker expression profiles, with capsule cells appearing as a transition from stroma to tumoral CN. It is thus tempting to speculate that CD90+ cells might be a precursor to the aforementioned capsule cells. Furthermore, some specimens in this study exhibited well-differentiated morphology while others resembled more poorly-differentiated tumors. Unfortunately, the authors did not pursue further or discuss this aspect given that poorly differentiated tumors, lacking tissue organization, are generally considered to be more aggressive (13). It would therefore be quite interesting to see how capsule cells fit into poor and well differentiated subsets of HNSCC.
The dynamic interactions that occur between neighboring cells play a crucial role in shaping the functional state of the TME and influencing tumor behavior. In their study, Weed et al. provided meaningful insights into the nature of these cell-cell contacts and their prevalence within various CNs. Notably, some CNs, such as the cold tumor and stroma, were characterized by predominantly homotypic contacts (i.e., interaction between same cell types) while the peritumoral CN displayed a combination of both homotypic and heterotypic contacts. Within the hot tumor, TLS1, and TLS2 CNs, heterotypic contacts were more prevalent. Plasma cells and M2 macrophages were the primary contributors to these contacts in the TLS1 and TLS2 CN, respectively. In the TLS1 domain, plasma cells exhibited heterotypic contacts primarily with CD90+ cells, a finding consistent with recent studies showcasing the role of fibroblasts in organizing TLSs and facilitating the infiltration of plasma cells into tumor tissue (14). Conversely, in the TLS2 CN, CD90+ cells established contacts primarily with tumor-associated M2 macrophages. This interaction could potentially influence the functional state of these cells, as CD90+ stromal cells have been reported to secrete cytokines that induce M2 macrophage polarization, which promotes cancer cell growth and metastasis (15).
Another notable finding highlighted by the authors is the enrichment of the TLS1 CN within the tumors of patients who did not experience recurrence. This enrichment pattern is particularly significant given the positive correlation observed between disease-specific survival and the co-expression of CD38 and CD31 markers that define long lived, antibody-secreting plasma cells residing within the TLS1 CN. Prior investigations have also revealed the prevalence of TLSs in tumors of patients who respond favorably to ICIs (16,17). These findings prompted the authors to delve into transcriptomic data from HNSCC tumors from The Cancer Genome Atlas. Their analysis revealed an association between increased expression levels of CD38 and CD31 in tumors and improved disease-specific survival. In agreement, a multicenter study involving 310 cases of HNSCC have shown the predictive potential of TLSs for patient survival, regardless of TNM stage (18). It is important to note that a previous landmark study utilizing CODEX also identified CNs resembling TLSs in colorectal cancer patients, which were likewise found to be associated with improved prognosis when localized to the tumor invasive front (19). One note of caution is that although these findings reinforce the role of TLSs in nurturing adaptive anticancer immune responses, they do not establish a causal correlation between clinical parameters and the presence of the TLS1 CN. Such information could potentially offer invaluable insights into why some patients may lack the protective immunity required to combat cancer cells effectively.
Finally, the authors noted the effect of sample preparation on data interpretation, as exemplified by the observation that CD3+ lymphocytes were overestimated while PMN-MDSC populations were underrepresented following tissue dissociation for flow cytometry. It is worth emphasizing that the preparation of single-cell suspensions poses the biggest challenge for data reproducibility in scRNA-seq studies. CODEX overcomes some of these limitations by assaying protein profiles while preserving the tissue architecture. One challenge with CODEX, however, is the recapitulation of tumor heterogeneity in the 3D context when sampling sections. The authors attempted to overcome this barrier by using full specimen sections and acquiring over 40,000 images per sample. However, the downstream analysis of these CODEX images involved an unsupervised, clustering-based approach followed by manual annotation of cell types, which is prone to bias. This problem can be tackled by developing consensus clustering frameworks based on unsupervised machine learning methods that rely on differential protein expression and spatial neighborhood enrichment, and by using emerging artificial intelligence-based approaches for automatic feature extraction from tissue sections (20,21).
There are several facets of the study design which researchers must consider in order to generate results that are better representative of HNSCC tumors. Weed et al. highlight the necessity for examining a more extensive cohort of patients to fortify the robustness and reliability of their conclusions. The constraints of a small sample size compelled the authors to forego multivariate analyses, thus impeding their capacity to comprehensively evaluate potential influences of clinical factors, such as radiation therapy and smoking history on patient outcomes. For example, a substantial proportion of patients displayed tumor profiles characterized by cold tumor and TLS2 CNs, which could potentially be linked to a history of smoking. Notably, five out of nine patients in this study presented this clinical feature, but the limited sample size precluded any conclusive correlations between a history of smoking and the prevalence of the TLS1/TLS2 CNs.
Another crucial consideration is the fact that HNSCC is a complex and heterogeneous disease with broad classification into HPV− or HPV+ subtypes. Currently, HPV has emerged as a predominant etiological factor driving HNSCC, and represents a growing health concern, particularly in the United States and Western Europe (22). Given that HPV+ HNSCC significantly varies from its HPV− counterpart in terms of disease development, immune response, and treatment outcomes, the finding that the presence of the TLS1 CN positively correlates with recurrence-free survival is quite interesting. Indeed, the presence of tumor-infiltrating lymphocytes has been associated with more favorable outcomes in HPV+ HNSCC (23). Furthermore, de-escalation strategies are currently being investigated for maximizing outcomes in HPV+ patients following therapy while limiting drug-induced cytotoxic effects. However, the success of these measures heavily depend on risk assessment following accurate classification of patients based on clinical staging and parameters such as smoking history (24). For HPV− HNSCC patients, genomic and molecular approaches have been the primary focus, revealing several subtypes based on gene expression profiles (25,26). The existence of these subtypes might explain variations observed in the composition and structural aspects of the TME as observed by the authors. Two molecular subtypes of HNSCC, the mesenchymal and basal subtype, have an extensive stromal TME, with scRNA-seq data suggesting that the mesenchymal subtype may actually be based on the contribution of CAFs, myofibroblasts, and myocytes found within the tumor stroma (27). The four tumors shown by Weed et al. to contain the capsule cell-rich peritumoral CN, may thus fall into the mesenchymal/basal subtype. A better understanding of how the TME influences patient outcomes and the integration of molecular subtyping and tumor architecture will be key in the discovery and success of targeted therapies.
In sum, Weed et al. have offered an in-depth exploration of the HNSCC tumor architecture and its association with prognosis following surgery, which thus far, has not been fully explored. Their findings highlight CODEX as a powerful and comprehensive tool for delving into the complexities of the TME and for uncovering novel cellular interactions, particularly in the context of TLSs. Future studies with a larger and more diverse patient cohort will unravel the fundamental cellular interactions that underlie the dynamic and heterogeneous HNSCC landscape and likely reveal potential therapeutic avenues.
Acknowledgments
Funding: None.
Footnote
Provenance and Peer Review: This article was commissioned by the editorial office, Translational Cancer Research. The article has undergone external peer review.
Peer Review File: Available at https://tcr.amegroups.com/article/view/10.21037/tcr-23-2098/prf
Conflicts of Interest: All authors have completed the ICMJE uniform disclosure form (available at https://tcr.amegroups.com/article/view/10.21037/tcr-23-2098/coif). J.S. reports support from the National Institute of General Medical Science (No. R25 GM095459 and No. T32 GM144920). The other authors have no conflicts of interest to declare.
Ethical Statement: The authors are accountable for all aspects of the work in ensuring that questions related to the accuracy or integrity of any part of the work are appropriately investigated and resolved.
Open Access Statement: This is an Open Access article distributed in accordance with the Creative Commons Attribution-NonCommercial-NoDerivs 4.0 International License (CC BY-NC-ND 4.0), which permits the non-commercial replication and distribution of the article with the strict proviso that no changes or edits are made and the original work is properly cited (including links to both the formal publication through the relevant DOI and the license). See: https://creativecommons.org/licenses/by-nc-nd/4.0/.
References
- Pilard C, Ancion M, Delvenne P, et al. Cancer immunotherapy: it's time to better predict patients' response. Br J Cancer 2021;125:927-38. [Crossref] [PubMed]
- Barsouk A, Aluru JS, Rawla P, et al. Epidemiology, Risk Factors, and Prevention of Head and Neck Squamous Cell Carcinoma. Med Sci (Basel) 2023;11:42. [Crossref] [PubMed]
- Chow LQM. Head and Neck Cancer. N Engl J Med 2020;382:60-72. [Crossref] [PubMed]
- Canning M, Guo G, Yu M, et al. Heterogeneity of the Head and Neck Squamous Cell Carcinoma Immune Landscape and Its Impact on Immunotherapy. Front Cell Dev Biol 2019;7:52. [Crossref] [PubMed]
- Weed DT, Zilio S, McGee C, et al. The Tumor Immune Microenvironment Architecture Correlates with Risk of Recurrence in Head and Neck Squamous Cell Carcinoma. Cancer Res 2023;83:3886-900. [Crossref] [PubMed]
- Gong Y, Bao L, Xu T, et al. The tumor ecosystem in head and neck squamous cell carcinoma and advances in ecotherapy. Mol Cancer 2023;22:68. [Crossref] [PubMed]
- Knops AM, South A, Rodeck U, et al. Cancer-Associated Fibroblast Density, Prognostic Characteristics, and Recurrence in Head and Neck Squamous Cell Carcinoma: A Meta-Analysis. Front Oncol 2020;10:565306. [Crossref] [PubMed]
- Geng X, Chen H, Zhao L, et al. Cancer-Associated Fibroblast (CAF) Heterogeneity and Targeting Therapy of CAFs in Pancreatic Cancer. Front Cell Dev Biol 2021;9:655152. [Crossref] [PubMed]
- Wu SZ, Roden DL, Wang C, et al. Stromal cell diversity associated with immune evasion in human triple-negative breast cancer. EMBO J 2020;39:e104063. [Crossref] [PubMed]
- Mou T, Zhu H, Jiang Y, et al. Heterogeneity of cancer-associated fibroblasts in head and neck squamous cell carcinoma. Transl Oncol 2023;35:101717. [Crossref] [PubMed]
- Mele D, Pessino G, Trisolini G, et al. Impaired intratumoral natural killer cell function in head and neck carcinoma. Front Immunol 2022;13:997806. [Crossref] [PubMed]
- Liotta F, Querci V, Mannelli G, et al. Mesenchymal stem cells are enriched in head neck squamous cell carcinoma, correlates with tumour size and inhibit T-cell proliferation. Br J Cancer 2015;112:745-54. [Crossref] [PubMed]
- Lin NC, Hsu JT, Tsai KY. Survival and clinicopathological characteristics of different histological grades of oral cavity squamous cell carcinoma: A single-center retrospective study. PLoS One 2020;15:e0238103. [Crossref] [PubMed]
- Rodriguez AB, Peske JD, Woods AN, et al. Immune mechanisms orchestrate tertiary lymphoid structures in tumors via cancer-associated fibroblasts. Cell Rep 2021;36:109422. [Crossref] [PubMed]
- Li W, Zhang X, Wu F, et al. Gastric cancer-derived mesenchymal stromal cells trigger M2 macrophage polarization that promotes metastasis and EMT in gastric cancer. Cell Death Dis 2019;10:918. [Crossref] [PubMed]
- Helmink BA, Reddy SM, Gao J, et al. B cells and tertiary lymphoid structures promote immunotherapy response. Nature 2020;577:549-55. [Crossref] [PubMed]
- Laumont CM, Banville AC, Gilardi M, et al. Tumour-infiltrating B cells: immunological mechanisms, clinical impact and therapeutic opportunities. Nat Rev Cancer 2022;22:414-30. [Crossref] [PubMed]
- Almangush A, Bello IO, Elseragy A, et al. Tertiary lymphoid structures associate with improved survival in early oral tongue cancer. BMC Cancer 2022;22:1108. [Crossref] [PubMed]
- Schürch CM, Bhate SS, Barlow GL, et al. Coordinated Cellular Neighborhoods Orchestrate Antitumoral Immunity at the Colorectal Cancer Invasive Front. Cell 2020;182:1341-1359.e19. [Crossref] [PubMed]
- Zhang W, Li I, Reticker-Flynn NE, et al. Identification of cell types in multiplexed in situ images by combining protein expression and spatial information using CELESTA. Nat Methods 2022;19:759-69. [Crossref] [PubMed]
- Huang Z, Shao W, Han Z, et al. Artificial intelligence reveals features associated with breast cancer neoadjuvant chemotherapy responses from multi-stain histopathologic images. NPJ Precis Oncol 2023;7:14. [Crossref] [PubMed]
- Conarty JP, Wieland A. The Tumor-Specific Immune Landscape in HPV+ Head and Neck Cancer. Viruses 2023;15:1296. [Crossref] [PubMed]
- Partlová S, Bouček J, Kloudová K, et al. Distinct patterns of intratumoral immune cell infiltrates in patients with HPV-associated compared to non-virally induced head and neck squamous cell carcinoma. Oncoimmunology 2015;4:e965570. [Crossref] [PubMed]
- Ruffin AT, Li H, Vujanovic L, et al. Improving head and neck cancer therapies by immunomodulation of the tumour microenvironment. Nat Rev Cancer 2023;23:173-88. [Crossref] [PubMed]
- Walter V, Yin X, Wilkerson MD, et al. Molecular subtypes in head and neck cancer exhibit distinct patterns of chromosomal gain and loss of canonical cancer genes. PLoS One 2013;8:e56823. [Crossref] [PubMed]
- Bard JE, Nowak NJ, Buck MJ, et al. Multimodal Dimension Reduction and Subtype Classification of Head and Neck Squamous Cell Tumors. Front Oncol 2022;12:892207. [Crossref] [PubMed]
- Puram SV, Tirosh I, Parikh AS, et al. Single-Cell Transcriptomic Analysis of Primary and Metastatic Tumor Ecosystems in Head and Neck Cancer. Cell 2017;171:1611-1624.e24. [Crossref] [PubMed]