The value of a panel of circulating microRNAs in screening prostate cancer
Highlight box
Key findings
• Our experiments constructed a four-miRNA panel with the ability to become a screening marker for prostate cancer.
What is known and what is new?
• miRNAs have important value in diagnosing cancer.
• Our study demonstrated the value of miRNAs in prostate cancer screening.
What is the implication, and what should change now?
• The results of this study help provide screening markers for prostate cancer, making the screening more efficient and cost-effective.
Introduction
Prostate cancer (PCa) is the second most common cancer in men, with an estimated 40,000 confirmed cases worldwide each year (1). The burden of PCa has risen rapidly in Asia recently, and the incidence and prevalence of PCa have risen in almost every country (2). Early stage PCa usually has no symptoms associated, and the clinical presentation and outcome vary greatly (3). Early diagnosis and monitoring can be effective in managing this disease (4). The following methods are currently used to diagnose PCa: digital rectal examination, determination of prostate specific antigen (PSA), biopsy, transrectal ultrasound and magnetic resonance imaging (MRI) (5,6). The only test that seems to be available as a screening tool is the serum PSA test. However, PSA lacks specificity as a PCa marker and it is also increased in benign prostatic hyperplasia (BPH) and prostatitis (7). Studies have shown that PSA screening has no positive or substantive effect on the overall survival of patients (8). In addition, MRI has important value in the diagnosis and staging of PCa (9), but due to its high cost, it is not suitable for cancer screening (10). Ultrasound-guided biopsy is generally the standard method for PCa biopsies, which is invasive and not suitable for large-scale screening (11). Hence, finding a biomarker for PCa screening is essential for men’s global health.
MicroRNA (miRNAs) are small, single-stranded, non-coding RNA molecules containing 21 to 23 nucleotides and it is possible to collect miRNAs from urine samples for a potential for a potential minimally invasive diagnostic test (12). miRNAs play a significant role in various pathophysiological procedures such as cell development, proliferation, differentiation, apoptosis, senescence, and cell recognition (13). The biology of diseases such as cancer is also linked to abnormally increased or decreased miRNA expression (14). miRNAs have frequently been identified as specific, sensitive, and stable, suggesting that they may be potential specific biomarkers for PCa (15). At the same time, miRNA in serum can be stably present and can be obtained by non-invasive methods (16), as well as requiring only simple techniques such as quantitative reverse transcription-polymerase chain reaction (qRT-PCR) to detect its expression level (17). Much research has demonstrated the potential of miRNAs to be a screening biomarker for PCa (18,19).
In our study, we detected some miRNAs related to PCa including miR-10a-5p, miR-372, miR-29a-3p, miR-183-5p, miR-1231, miR-199b-3p, miR-489-3p, miR-191, miR-129-5p, miR-486-5p, miR-10a-5p, miR-429. Throughout the training and validation phases, the expression levels of different miRNAs in the serum of PCa patients and healthy controls (HCs) were measured by qRT-PCR to screen for miRNAs with diagnostic value. We selected miRNAs with high diagnostic value to make up a diagnostic panel. Furthermore, we used these miRNAs for target gene prediction and bioinformatics analysis. We present this article in accordance with the STARD reporting checklist (available at https://tcr.amegroups.com/article/view/10.21037/tcr-23-1313/rc).
Methods
Participants and ethics statement
From January 2018 to September 2020, we recruited 224 participants at Peking University Shenzhen Hospital, including 112 patients with PCa and 112 HCs. The study was conducted in accordance with the Declaration of Helsinki (as revised in 2013). The study was approved by the Ethics Committee of Peking University Shenzhen Hospital (No. 2019-043) and informed consent was taken from all the patients. All PCa patients were pathologically diagnosed with PCa after surgical removal of cancerous tissue. Apart from this, none of the patients had history of cancer nor did they receive any treatment before the serum sample was taken. HCs were males of the same age who had no prostate disease and no family history of PCa. These patients were all Asians from hospitals in the region. Table 1 sets out the populations and clinical outcomes of the 224 participants. The difference in age distribution between PCa patients and HCs was not statistically significant, with a P value >0.05.
Table 1
Classifications | Training stage (n=56) | Validation stage (n=168) | |||
---|---|---|---|---|---|
Cases (%) | Controls (%) | Cases (%) | Controls (%) | ||
Total number | 28 | 28 | 84 | 84 | |
Age (years) | 68.0±7.5 | 68.2±8.0 | 68.6±8.1 | 68.3±8.3 | |
Tumor stage | |||||
≤T2 | 17 (60.7) | 51 (60.7) | |||
>T2 | 11 (39.3) | 33 (39.3) | |||
Lymph node metastasis | |||||
Positive | 3 (10.7) | 8 (9.5) | |||
Negative | 25 (89.3) | 76 (90.5) | |||
Bone metastasis | |||||
Positive | 2 (7.1) | 7 (8.3) | |||
Negative | 26 (92.9) | 77 (91.7) | |||
ISUP grade group | |||||
1 | 7 (25.0) | 21 (25.0) | |||
2 | 8 (28.6) | 22 (26.2) | |||
3 | 2 (7.2) | 8 (9.5) | |||
4 | 4 (14.2) | 12 (14.3) | |||
5 | 7 (25.0) | 21 (25.0) | |||
PSA, ng/mL | |||||
Mean value | 28.85 | 30.03 | |||
Standard deviation | 47.31 | 45.39 |
Data are presented as average value ± standard deviation and n (%). ISUP, International Society of Urological Pathology; PSA, prostate specific antigen.
Study design
Firstly, we selected 12 miRNAs from literature published in PubMed that are believed to be associated with PCa and are generally expressed aberrant in prostate tissue. The retrieval strategy was as follow: “Prostate cancer” AND “MicroRNAs”[Mesh]. Then, the 12 miRNAs showing highest potential, for which there was no literature on their expression in serum, were selected for further investigation (Table 2). A total of 12 miRNAs were aberrantly expressed in cancer tissues, including: miR-10a-5p, miR-372, miR-29a-3p, miR-183-5p, miR-1231, miR-199b-3p, miR-489-3p, miR-191, miR-129-5p, miR-486-5p, miR-10a-5p, miR-429. During the training phase, we measured the expression levels of these 12 miRNAs in the serum of 28 PCa patients and 28 HCs. The miRNAs aberrantly expressed in the sera of PCa patients were put into the validation set for further testing. In the validation phase, we increased the sample size, examined the expression levels of these miRNAs in the serum, calculated their P values, drew the receiver operating characteristic curve (ROC), and calculated the area under the curve (AUC). Ultimately, a miRNA panel with high specificity and sensitivity was constructed by stepwise logistic regression.
Table 2
Candidate miRNA | Corresponding reference title |
---|---|
hsa-miR-10a-5p | miR-10a-5p and miR-29b-3p as Extracellular Vesicle-Associated Prostate Cancer Detection Markers |
Brain-derived neurotrophic factor (BDNF) and its signaling in cancer | |
hsa-miR-29a-3p | The LINC00852/miR-29a-3p/JARID2 axis regulates the proliferation and invasion of prostate cancer cell |
Circular RNA circFOXO3 promotes prostate cancer progression through sponging miR-29a-3p | |
lncRNA-MIAT regulates cell biological behaviors in gastric cancer through a mechanism involving the miR-29a-3p/HDAC4 axis | |
hsa-miR-129-5p | MiR-129-5p/DLX1 signaling axis mediates functions of prostate cancer during malignant progression |
miR-129-5p inhibits prostate cancer proliferation via targeting ETV1 | |
Norcantharidin induces autophagy-related prostate cancer cell death through Beclin-1 upregulation by miR-129-5p suppression | |
hsa-miR-181a-5p | miR-181a-5p is downregulated and inhibits proliferation and the cell cycle in prostate cancer |
Migration and invasion inhibitory protein (MIIP) inhibits epithelial-mesenchymal transition (EMT) and cell invasion in prostate cancer through miR-181a/b-5p-KLF17 axis | |
hsa-miR-183-5p | MiR-183-5p promotes migration and invasion of prostate cancer by targeting TET1 |
Hsa-miR-183-5p Modulates Cell Adhesion by Repression of ITGB1 Expression in Prostate Cancer | |
hsa-miR-191 | Upregulation of miR-191 promotes cell growth and invasion via targeting TIMP3 in prostate cancer |
Activin A regulates microRNAs and gene expression in LNCaP cells | |
hsa-miR-199b-3p | MicroRNA-199b-3p suppresses malignant proliferation by targeting Phospholipase Cε and correlated with poor prognosis in prostate cancer |
hsa-miR-372 | microRNA-372 Suppresses Migration and Invasion by Targeting p65 in Human Prostate Cancer Cells |
hsa-miR-429 | miR-429 as biomarker for diagnosis, treatment and prognosis of cancers and its potential action mechanisms: A systematic literature review |
Urinary microRNAs can predict response to abiraterone acetate in castration resistant prostate cancer: A pilot study | |
hsa-miR-486-5p | miR-486-5p suppresses prostate cancer metastasis by targeting Snail and regulating epithelial-mesenchymal transition |
The miR-486-5p plays a causative role in prostate cancer through negative regulation of multiple tumor suppressor pathways | |
hsa-miR-489-3p | miR-489-3p Inhibits Prostate Cancer Progression by Targeting Distal-Less Homeobox 1 (DLX1) |
has-miR-1231 | miR-1231 Is Downregulated in Prostate Cancer with Prognostic and Functional Implications |
Obtaining serum specimens from patients and HCs
The study collected serum samples from 224 men between January 2018 and September 2020, including 112 patients with pathologically confirmed PCa after surgical resection and 112 HCs. 10 mL serum sample was taken from each partaker and centrifuged at 3,000 g for 10 min at 4 ℃ for 2h. Serum samples were stored at −80 ℃ and frozen for RNA extraction. The clinical characteristics of the participants are shown in Table 1.
RNA extraction and RT-qPCR
We added 2 µL cel-miR-54-5p (10 nm/L, RiboBio, China) to each sample, which was used to standardize the variability in the extraction process as an internal reference (20). Subsequently, total RNA from samples were extracted by TRIzol LS isolation kit (Thermo Fisher Scientific, Waltham, MA, USA) and RNA concentration and purity were gauged by NanoDrop 2000c spectrophotometer (Thermo Scientific, USA). qRT-PCR was served to determine the expression level of these miRNAs. The miRNAs were amplified by the reverse transcription-specific primers of the Bulge-Loop miRNA qRT-PCR primer set (RiboBio, Guangzhou, China) in reverse transcription. Then, by the qPCR with the Taqman probe, the expression level of miRNAs was detected on the LightCycler480 Real-Time PCR system (Roche Diagnostics, Mannheim, Germany). The qRT-PCR was performed with 40 cycles following at 95 ℃ for 20 s, 95 ℃ for 10 s, 60 ℃ for 20 s, and 70 ℃ for 10 s.
Statistical analyses
We used 2−ΔΔCq method to calculate the miRNAs expression levels (21). Categorical variables between groups were reported in the form of numbers and percentages. Continuous variables were presented as the average value ± standard deviation. Kruskal-Wally’s rank test was used to compare several distinct phases. Different expression levels of candidate miRNAs in PCa and HC samples were anatomized by using Students’ T-test or Mann-Whitney test. The diagnostic miRNA panel was designed with the aid of multiple logistic regression analysis. The ROC curve and AUC value were deemed as the diagnostic value for miRNAs. P value <0.05 was set as statistically significant. SPSS software (SPSS 26.0 Inc, Chicago, IL, USA) GraphPad Prism 8 (GraphPad Prism 9.4.1.681) and Medcalc (Version 19) were used for the statistical processing.
Bioinformatics analysis
miRWalk3.0 database was used to identify target genes for candidate miRNAs. Genes predicted by over three candidate miRNAs were chosen as target genes, then placed into the David database (22,23) for gene ontology (GO) functional annotation and pathway analysis, and visualized by using ImageGP (24). Through analysis of the GEPIA database (25), we examined target genes of which the expression was significantly different in PCa patients compared with HCs, which may be related to tumorigenesis and progression of PCa.
Results
Testing candidate miRNAs at the training phase
During the training phase, the corresponding miRNAs expression levels were measured in the serum of 28 PCa patients and 28 HCs using qRT-PCR. The expression levels of these 12 miRNAs in PCa patients and HCs are shown in Figure 1. At a standard of P value <0.05, we found a total of six miRNAs including miR-429, miR-10a-5p, miR-183-5p, miR-181a-5p, miR-1231, miR-129-5p that remained abnormally expressed in serum in the cancer group and HCs. We further tested the expression levels of these miRNAs during the validation phase.
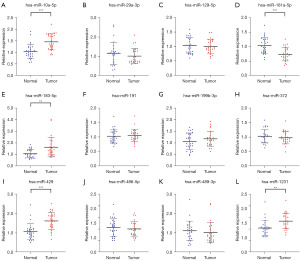
Further validation of candidate miRNAs
In the validation phase, we further examined the expression levels of these six miRNAs in serum samples from 84 PCa patients and 84 HCs. The expression levels and ROC curves of these miRNAs are shown in Figure 2. The results are as follows: the expression level of miR-10a-5p, miR-183-5p and miR-429 were meaningfully upregulated (P value <0.001) and the remaining three miRNAs expression levels were all downregulated. The results of AUC values are as follows: 0.671 [95% confidence interval (CI): 0.594–0.742; Figure 2B] for miR-10a-5p, 0.636 (95% CI: 0.557–0.709; Figure 2D) for miR-129-5p, 0.570 (95% CI: 0.491–0.547; Figure 2F) for miR-181a-5p, 0.646 (95% CI: 0.569–0.720; Figure 2H) for miR-183-5p, 0.714 (95% CI: 0.639–0.782; Figure 2J) for miR-429 and 0.624 (95% CI: 0.566–0.717; Figure 2L) for miR-1231. Next, in Table 3, Youden index was used to compute the best cut- off value and specify the best specificity and sensitivity of these five miRNAs for PCa diagnostics.
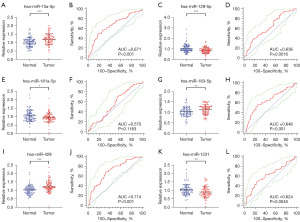
Table 3
miRNAs | AUC | 95% CI | P value | Associated criterion | Sensitivity (%) | Specificity (%) |
---|---|---|---|---|---|---|
miR-1231 | 0.624 | 0.566–0.717 | 0.005 | ≤0.827 | 51.19 | 78.05 |
miR-181a-5p | 0.570 | 0.491–0.547 | 0.116 | ≤1.027 | 70.24 | 47.56 |
miR-10a-5p | 0.671 | 0.594–0.742 | <0.001 | >1.212 | 47.62 | 82.93 |
miR-183-5p | 0.646 | 0.569–0.720 | <0.001 | >1.228 | 42.86 | 85.37 |
miR-429 | 0.714 | 0.639–0.782 | <0.001 | >1.116 | 67.86 | 68.29 |
miR-129-5p | 0.636 | 0.557–0.709 | 0.002 | ≤0.797 | 40.48 | 81.71 |
Four-miRNA panel | 0.878 | 0.843–0.941 | <0.001 | >0.435 | 83.33 | 79.27 |
AUC, area under curve; CI, confidence interval.
Building a four-miRNA panel for PCa screening
We found that constructing a panel with a group of miRNAs had higher diagnostic value than a single miRNA. Therefore, we constructed a four-miRNA diagnostic panel including miR-1231, miR-10a-5p, miR-429 and miR-129-5p through a stepwise logistic regression model. The last logistic regression model is based on the following formula: [logit p=−2.032 + (−2.6×miR-1231) + (2.821×miR-10a-5p) + (−5.576×miR-429) + (−5.104×miR-129-5p)]. The roc of this four-miRNA panel is shown in Figure 3, with an AUC value of 0.878 (95% CI: 0.843–0.941; sensitivity =83.33%, specificity =79.27%; Table 3).
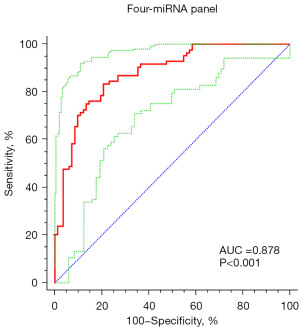
Bioinformatics analysis of four miRNAs
We performed target gene prediction for these four miRNAs including miR-1231, miR-10a-5p, miR-429 and miR-129-5p in mirWalk 3.0. As shown in Figure 4A, a total of 210 target genes were predicted by three and more miRNAs. To further investigate the potential functions of these target genes, an analysis of GO annotation and KEGG enrichment was carried out in the DAVID database (22,23). GO functional annotation consisted of biological process (BP), cellular component (CC), and molecular function (MF). We also put the target genes that were simultaneously predicted by the four miRNAs into the GEPIA (26) database. At the criterion of P value <0.01 and |log2FC| cutoff >1, NRG1 was discovered to be differentially expressed in PCa and normal tissues as shown in Figure 4B. Figure 5 shows the first five elements of the GO annotation including vesicle-mediated transport (GO:0016192), positive regulation of bone morphogenetic protein (BMP) signaling pathway (GO:0030513), mesoderm formation (GO:0001707), retinal cone cell development (GO:0046549),and ceramide catabolic process (GO:0046514) in the BP category; anchoring junction (GO:0070161), external side of plasma membrane (GO:0009897), early endosome (GO:0005769), cytoplasmic vesicle membrane (GO:0030659), mRNA cap binding complex (GO:0005845) in the CC category; RNA binding (GO:0003723), myosin phosphatase activity (GO:0017018), receptor tyrosine kinase binding (GO:0030971), chemorepellent activity (GO:0045499), ceramidase activity (GO:0017040) in the MF category. The enriched KEGG pathway contained pathways in PI3K-Akt signaling pathway and EGFR tyrosine kinase inhibitor resistance.

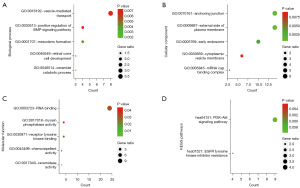
Discussion
PCa is a considerable public health anxiety around the world and remains one of the causes of cancer-related morbidity and mortality (27). Early diagnosis and treatment can effectively improve the prognosis of the disease (4). However, the commonly used biomarker PSA has not yet been shown to be a routine screening tool (28) because it is susceptible to interference from some benign diseases. Research (29) has shown that miRNAs can target a large number of genes and play a key role in basic cellular processes such as cell differentiation, cell cycle progression, proliferation, apoptosis, epithelial mesenchymal transition (EMT), angiogenesis, tumor cell metabolism, and metastasis through a special mechanism (30). Many studies (31,32) have demonstrated that urinary miRNAs are a potential biomarker for the diagnosis of PCa. In PCa, miRNAs affect the occurrence and development of cancer by stimulating cell cycle, avoiding cell apoptosis and EMT, and regulating androgen-receptor mediated signaling (33). miRNAs in tissues and body fluids can be applied to be a diagnostic and prognostic marker for cancer due to its often-dysregulated expression and stable presence (34,35). In our study, a four-miRNA panel with the highest diagnostic value was selected through three stages of screening, training, and validation. This panel has the potential to become a PCa screening marker [AUC =0.878 (95% CI: 0.843–0.941); sensitivity =83.33%, specificity =79.27%]. Due to the low cost of detecting miRNAs in serum, it is easy to apply in prostate screening. This significantly improves the prognosis by helping to detect PCa at an early stage. Although our trial did not further investigate the relationship between these miRNAs and PCa staging, research has demonstrated a marked decrease in miRNAs expression levels in metastatic PCa tissue (36). It seems to indicate that miRNAs also contribute to the staging of PCa and provides more targeted treatment for patients.
In our panel, miR-10a-5p has been shown to have diagnostic potential for PCa in extracellular vesicles (37,38). MiR-10a-5p can also influence PCa development by regulating the brain-derived neurotrophic factor (BDNF)/TRKB pathway (39). It has also been shown that high levels of mir in tissues are associated with the prognosis of PCa (40). These studies have demonstrated the important role of miR-10a-5p in PCa.
In a previous study, mir-429 has been reported to be implicated in epithelial-mesenchymal transition, progression, development, invasion, metastases, apoptosis, and drug resistance in a variety of cancers (41). In PCa, miRNAs can inhibit cell proliferation by targeting p27Kip1 (42). In breast cancer, miRNAs are seen as potential diagnostic and prognostic markers (43), and our study demonstrated its value in PCa. Currently, there is a study on miR-1231. Expression of miR-1231 is downregulated in both PCa tissues and cell lines, and usually acts as a tumor suppressor (44). The pathways through which tumors are affected are currently worthy of continued study.
Another miRNA in the diagnostic panel is miR-129-5p. MiR-129-5p acts as an oncogenic factor that can impede cancer cell growth by targeting etv1 and inhibiting DLX1 expression (45,46). MiR-129-5p has been shown to promote resistance to doxorubicin in PC-3-DR cells by inhibiting CAMK2N1 expression (47). This gives a new direction for the treatment of PCa.
To further explore the function and role of this group of miRNAs, we performed target gene prediction in mirWalk 3.0. The results suggested that these aberrantly regulated miRNAs targets were associated with many important cancer-related biological processes and pathways such as PI3K−Akt signaling pathway and EGFR tyrosine kinase inhibitor resistance. In the biological process terms, vesicle-mediated transport and positive regulation of BMP signaling pathway are encompassed in the development of many cancers (48-50). An examination by Chen et al. demonstrated that PI3K-Akt regulates metastasis and invasion of PCa cells (51). Sinomenine inhibits PCa cell proliferation, migration, invasion and promotes apoptosis of cancer cells by regulating miR-23a (52). CUDC-907 inhibits PI3K-Akt and histone deacetylases to suppress PCa tumor growth in mice (53). This brings a new strategy for the treatment of PC. Many protein molecules can inhibit the growth and metastasis of PCa by affecting EGFR such as FBXW2 and DUSP22 (54,55).
Under the criterion of P value <0.01 and |log2FC| cutoff >1 based on the expression level, NRG1 was chosen as the target gene among four miRNAs predicted at the same time by the GEPIA database. NRG1 has been less studied in PCa and may be an independent risk factor and associated with the development of PCa (56,57). In triple negative breast cancer (TNBC), NRG1 regulates Fra-1 expression and coordinates cancer cell metastasis via the novel ERK1/2-Fbxw7-c-Myc pathway. This makes NGR1 a possible target for the treatment of TNBC (58). In the same way, NRG1 has emerged as a promising target for targeted therapy in non-small cell lung cancer (59).
It appears that our constructed miRNAs panel has a high diagnostic value from a statistical point of view. This provides a more cost-effective and efficient method for screening PCa. Our experiment still has some shortcomings. For instance, our samples are limited in size and come from only one region. We may have overlooked other valuable miRNAs in the serum. These factors may have brought some influence on the experimental results.
Conclusions
We have constructed a four-miRNA panel with the ability to become a screening marker for PCa. This facilitates early detection and timely treatment of PCa and may reduce the overdiagnosis and treatment associated with PSA screening. And targets genes NRG1 may be encompassed in the development and progression of PCa and holds promise as a new therapeutic target.
Acknowledgments
Funding: This study was supported by
Footnote
Reporting Checklist: The authors have completed the STARD reporting checklist. Available at https://tcr.amegroups.com/article/view/10.21037/tcr-23-1313/rc
Data Sharing Statement: Available at https://tcr.amegroups.com/article/view/10.21037/tcr-23-1313/dss
Peer Review File: Available at https://tcr.amegroups.com/article/view/10.21037/tcr-23-1313/prf
Conflicts of Interest: All authors have completed the ICMJE uniform disclosure form (available at https://tcr.amegroups.com/article/view/10.21037/tcr-23-1313/coif). The authors have no conflicts of interest to declare.
Ethical Statement: The authors are accountable for all aspects of the work in ensuring that questions related to the accuracy or integrity of any part of the work are appropriately investigated and resolved. The study was conducted in accordance with the Declaration of Helsinki (as revised in 2013). The study was approved by the Ethics Committee of Peking University Shenzhen Hospital (No. 2019-043) and informed consent was taken from all the patients.
Open Access Statement: This is an Open Access article distributed in accordance with the Creative Commons Attribution-NonCommercial-NoDerivs 4.0 International License (CC BY-NC-ND 4.0), which permits the non-commercial replication and distribution of the article with the strict proviso that no changes or edits are made and the original work is properly cited (including links to both the formal publication through the relevant DOI and the license). See: https://creativecommons.org/licenses/by-nc-nd/4.0/.
References
- EAU Guidelines. Edn. presented at the EAU Annual Congress Milan 2023. ISBN 978-94-92671-19-6.
- Luo LS, Jiang JF, Luan HH, et al. Spatial and temporal patterns of prostate cancer burden and their association with Socio-Demographic Index in Asia, 1990-2019. Prostate 2022;82:193-202. [Crossref] [PubMed]
- Uhr A, Glick L, Gomella LG. An overview of biomarkers in the diagnosis and management of prostate cancer. Can J Urol 2020;27:24-7. [PubMed]
- Sardana G, Diamandis EP. Biomarkers for the diagnosis of new and recurrent prostate cancer. Biomark Med 2012;6:587-96. [Crossref] [PubMed]
- Cersosimo RJ, Carr D. Prostate cancer: current and evolving strategies. Am J Health Syst Pharm 1996;53:381-96; quiz 446-8. [Crossref] [PubMed]
- Paladini A, Cochetti G, Colau A, et al. The Challenges of Patient Selection for Prostate Cancer Focal Therapy: A Retrospective Observational Multicentre Study. Curr Oncol 2022;29:6826-33. [Crossref] [PubMed]
- Tenke P, Horti J, Balint P, et al. Prostate cancer screening. Recent Results Cancer Res 2007;175:65-81. [Crossref] [PubMed]
- Barry MJ, Simmons LH. Prevention of Prostate Cancer Morbidity and Mortality: Primary Prevention and Early Detection. Med Clin North Am 2017;101:787-806. [Crossref] [PubMed]
- Sun Y, Reynolds HM, Parameswaran B, et al. Multiparametric MRI and radiomics in prostate cancer: a review. Australas Phys Eng Sci Med 2019;42:3-25. [Crossref] [PubMed]
- Van Poppel H, Albreht T, Basu P, et al. Serum PSA-based early detection of prostate cancer in Europe and globally: past, present and future. Nat Rev Urol 2022;19:562-72. [Crossref] [PubMed]
- Wolf AM, Wender RC, Etzioni RB, et al. American Cancer Society guideline for the early detection of prostate cancer: update 2010. CA Cancer J Clin 2010;60:70-98. [Crossref] [PubMed]
- Cochetti G, Cari L, Maulà V, et al. Validation in an Independent Cohort of MiR-122, MiR-1271, and MiR-15b as Urinary Biomarkers for the Potential Early Diagnosis of Clear Cell Renal Cell Carcinoma. Cancers (Basel) 2022;14:1112. [Crossref] [PubMed]
- Wang L, Li B, Li L, et al. MicroRNA-497 suppresses proliferation and induces apoptosis in prostate cancer cells. Asian Pac J Cancer Prev 2013;14:3499-502. [Crossref] [PubMed]
- Lee D, Tang W, Dorsey TH, et al. miR-484 is associated with disease recurrence and promotes migration in prostate cancer. Biosci Rep 2020;40:BSR20191028. [Crossref] [PubMed]
- Moya L, Meijer J, Schubert S, et al. Assessment of miR-98-5p, miR-152-3p, miR-326 and miR-4289 Expression as Biomarker for Prostate Cancer Diagnosis. Int J Mol Sci 2019;20:1154. [Crossref] [PubMed]
- Porzycki P, Ciszkowicz E, Semik M, et al. Combination of three miRNA (miR-141, miR-21, and miR-375) as potential diagnostic tool for prostate cancer recognition. Int Urol Nephrol 2018;50:1619-26. [Crossref] [PubMed]
- Fabris L, Ceder Y, Chinnaiyan AM, et al. The Potential of MicroRNAs as Prostate Cancer Biomarkers. Eur Urol 2016;70:312-22. [Crossref] [PubMed]
- Pang C, Song X, Fu C, et al. Diagnostic Value of Peripheral Blood miR-374b-5p in Patients with Prostate Cancer. Clin Lab 2020; [Crossref] [PubMed]
- Tinay I, Tan M, Gui B, et al. Functional roles and potential clinical application of miRNA-345-5p in prostate cancer. Prostate 2018;78:927-37. [Crossref] [PubMed]
- Niu Y, Su M, Wu Y, et al. Circulating Plasma miRNAs as Potential Biomarkers of Non-Small Cell Lung Cancer Obtained by High-Throughput Real-Time PCR Profiling. Cancer Epidemiol Biomarkers Prev 2019;28:327-36. [Crossref] [PubMed]
- Livak KJ, Schmittgen TD. Analysis of relative gene expression data using real-time quantitative PCR and the 2(-Delta Delta C(T)) Method. Methods 2001;25:402-8. [Crossref] [PubMed]
- Sherman BT, Hao M, Qiu J, et al. DAVID: a web server for functional enrichment analysis and functional annotation of gene lists (2021 update). Nucleic Acids Res 2022;50:W216-21. [Crossref] [PubMed]
- Huang da W. Sherman BT, Lempicki RA. Systematic and integrative analysis of large gene lists using DAVID bioinformatics resources. Nat Protoc 2009;4:44-57. [Crossref] [PubMed]
- Li J, Miao B, Wang S, et al. Hiplot: a comprehensive and easy-to-use web service for boosting publication-ready biomedical data visualization. Brief Bioinform 2022;23:bbac261. [Crossref] [PubMed]
- Tang Z, Li C, Kang B, et al. GEPIA: a web server for cancer and normal gene expression profiling and interactive analyses. Nucleic Acids Res 2017;45:W98-W102. [Crossref] [PubMed]
- Li C, Tang Z, Zhang W, et al. GEPIA2021: integrating multiple deconvolution-based analysis into GEPIA. Nucleic Acids Res 2021;49:W242-6. [Crossref] [PubMed]
- Scott E. Prostate cancer. ScientificWorldJournal 2011;11:749-50. [Crossref] [PubMed]
- Lefevre ML. Prostate cancer screening: more harm than good? Am Fam Physician 1998;58:432-8. [PubMed]
- Coradduzza D, Cruciani S, Arru C, et al. Role of miRNA-145, 148, and 185 and Stem Cells in Prostate Cancer. Int J Mol Sci 2022;23:1626. [Crossref] [PubMed]
- Cochetti G, Rossi de Vermandois JA, Maulà V, et al. Role of miRNAs in prostate cancer: Do we really know everything? Urol Oncol 2020;38:623-35. [Crossref] [PubMed]
- Juracek J, Madrzyk M, Stanik M, et al. Urinary microRNAs and Their Significance in Prostate Cancer Diagnosis: A 5-Year Update. Cancers (Basel) 2022;14:3157. [Crossref] [PubMed]
- Aveta A, Cilio S, Contieri R, et al. Urinary MicroRNAs as Biomarkers of Urological Cancers: A Systematic Review. Int J Mol Sci 2023;24:10846. [Crossref] [PubMed]
- Jackson BL, Grabowska A, Ratan HL. MicroRNA in prostate cancer: functional importance and potential as circulating biomarkers. BMC Cancer 2014;14:930. [Crossref] [PubMed]
- Wang Z, Sha HH, Li HJ. Functions and mechanisms of miR-186 in human cancer. Biomed Pharmacother 2019;119:109428. [Crossref] [PubMed]
- Di Minno A, Aveta A, Gelzo M, et al. 8-Hydroxy-2-Deoxyguanosine and 8-Iso-Prostaglandin F2α: Putative Biomarkers to assess Oxidative Stress Damage Following Robot-Assisted Radical Prostatectomy (RARP). J Clin Med 2022;11:6102. [Crossref] [PubMed]
- Nayak B, Khan N, Garg H, et al. Role of miRNA-182 and miRNA-187 as potential biomarkers in prostate cancer and its correlation with the staging of prostate cancer. Int Braz J Urol 2020;46:614-23. [Crossref] [PubMed]
- Worst TS, Previti C, Nitschke K, et al. miR-10a-5p and miR-29b-3p as Extracellular Vesicle-Associated Prostate Cancer Detection Markers. Cancers (Basel) 2019;12:43. [Crossref] [PubMed]
- Ku A, Fredsøe J, Sørensen KD, et al. High-Throughput and Automated Acoustic Trapping of Extracellular Vesicles to Identify microRNAs With Diagnostic Potential for Prostate Cancer. Front Oncol 2021;11:631021. [Crossref] [PubMed]
- Malekan M, Nezamabadi SS, Samami E, et al. BDNF and its signaling in cancer. J Cancer Res Clin Oncol 2023;149:2621-36. [Crossref] [PubMed]
- Che P, Jiang S, Zhang W, et al. A novel prognostic model based on three clinic-related miRNAs for prostate cancer. Front Surg 2022;9:872953. [Crossref] [PubMed]
- Guo CM, Liu SQ, Sun MZ. miR-429 as biomarker for diagnosis, treatment and prognosis of cancers and its potential action mechanisms: A systematic literature review. Neoplasma 2020;67:215-28. [Crossref] [PubMed]
- Ouyang Y, Gao P, Zhu B, et al. Downregulation of microRNA-429 inhibits cell proliferation by targeting p27Kip1 in human prostate cancer cells. Mol Med Rep 2015;11:1435-41. [Crossref] [PubMed]
- Dai W, He J, Zheng L, et al. miR-148b-3p, miR-190b, and miR-429 Regulate Cell Progression and Act as Potential Biomarkers for Breast Cancer. J Breast Cancer 2019;22:219-36. [Crossref] [PubMed]
- Wang Y, Zhang Q, Guo B, et al. miR-1231 Is Downregulated in Prostate Cancer with Prognostic and Functional Implications. Oncol Res Treat 2020;43:78-86. [Crossref] [PubMed]
- Gao G, Xiu D, Yang B, et al. miR-129-5p inhibits prostate cancer proliferation via targeting ETV1. Onco Targets Ther 2019;12:3531-44. [Crossref] [PubMed]
- Li Q, Xu K, Tian J, et al. MiR-129-5p/DLX1 signalling axis mediates functions of prostate cancer during malignant progression. Andrologia 2021;53:e14230. [Crossref] [PubMed]
- Wu C, Miao C, Tang Q, et al. MiR-129-5p promotes docetaxel resistance in prostate cancer by down-regulating CAMK2N1 expression. J Cell Mol Med 2020;24:2098-108. [Crossref] [PubMed]
- Brena D, Huang MB, Bond V. Extracellular vesicle-mediated transport: Reprogramming a tumor microenvironment conducive with breast cancer progression and metastasis. Transl Oncol 2022;15:101286. [Crossref] [PubMed]
- Havrylov S, Redowicz MJ, Buchman VL. Emerging roles of Ruk/CIN85 in vesicle-mediated transport, adhesion, migration and malignancy. Traffic 2010;11:721-31. [Crossref] [PubMed]
- Dituri F, Cossu C, Mancarella S, et al. The Interactivity between TGFβ and BMP Signaling in Organogenesis, Fibrosis, and Cancer. Cells 2019;8:1130. [Crossref] [PubMed]
- Chen H, Zhou L, Wu X, et al. The PI3K/AKT pathway in the pathogenesis of prostate cancer. Front Biosci (Landmark Ed) 2016;21:1084-91. [Crossref] [PubMed]
- Xu F, Li Q, Wang Z, et al. Sinomenine inhibits proliferation, migration, invasion and promotes apoptosis of prostate cancer cells by regulation of miR-23a. Biomed Pharmacother 2019;112:108592. [Crossref] [PubMed]
- Hu C, Xia H, Bai S, et al. CUDC-907, a novel dual PI3K and HDAC inhibitor, in prostate cancer: Antitumour activity and molecular mechanism of action. J Cell Mol Med 2020;24:7239-53. [Crossref] [PubMed]
- Lin HP, Ho HM, Chang CW, et al. DUSP22 suppresses prostate cancer proliferation by targeting the EGFR-AR axis. FASEB J 2019;33:14653-67. [Crossref] [PubMed]
- Zhou T, Chen T, Lai B, et al. FBXW2 inhibits prostate cancer proliferation and metastasis via promoting EGFR ubiquitylation and degradation. Cell Mol Life Sci 2022;79:268. [Crossref] [PubMed]
- Kamdar S, Isserlin R, Van der Kwast T, et al. Exploring targets of TET2-mediated methylation reprogramming as potential discriminators of prostate cancer progression. Clin Epigenetics 2019;11:54. [Crossref] [PubMed]
- Liao J, Ye Y, Xu X. Comprehensive analysis of tumor mutation burden and immune microenvironment in prostate cancer. Clin Transl Oncol 2022;24:1986-97. [Crossref] [PubMed]
- Shu L, Chen A, Li L, et al. NRG1 regulates Fra-1 transcription and metastasis of triple-negative breast cancer cells via the c-Myc ubiquitination as manipulated by ERK1/2-mediated Fbxw7 phosphorylation. Oncogene 2022;41:907-19. [Crossref] [PubMed]
- Yang SR, Schultheis AM, Yu H, et al. Precision medicine in non-small cell lung cancer: Current applications and future directions. Semin Cancer Biol 2022;84:184-98. [Crossref] [PubMed]