Comprehensive analysis of exosome gene LYPD3 and prognosis/immune cell infiltration in lung cancer
Highlight box
Key findings
• We found that Ly6/PLAUR domain containing 3 (LYPD3) is involved in many biological processes of lung cancer (LC), such as regulating immune cell infiltration and affecting prognosis.
What is known and what is new?
• LC is a leading cause of cancer-associated mortality worldwide, with high incidence and mortality rates. LYPD3 is a tumorigenic and highly glycosylated cell surface protein that has been rarely reported in LC.
• This study explored the prognostic role of LYPD3 in LC and the infiltration of immune cells.
What is the implication, and what should change now?
• Our findings highlight the clinical value and prognostic significance of LYPD3 in LC, providing a theoretical and molecular basis for future clinical and basic research.
Introduction
Lung cancer (LC) is a prevalent malignancy with high morbidity and mortality rates (1-3). Its two primary pathological classifications are non-small-cell lung carcinoma (NSCLC) and small-cell lung carcinoma (SCLC) (4). Early symptoms of LC are often non-specific, resulting in delayed diagnosis and treatment (5). As such, early detection is critical for improving disease-free survival (DFS) and overall survival (OS) (6,7). While low-dose computed tomography can reduce mortality by 20% (8), identifying a biomarker for early screening would be more convenient and effective. The discovery of effective biomarkers can aid in diagnosis and prognosis prediction, ultimately reducing LC-induced mortality. Thus, the identification of a biomarker for LC detection is of utmost importance.
C4.4A [Ly6/PLAUR domain containing 3 (LYPD3)] is a cell surface protein implicated in tumorigenesis and metastasis (9-12). LYPD3 has been shown to be highly expressed in colorectal cancer, breast cancer, and renal cell carcinoma, with stronger expression in metastatic tissues (13-15). Jacobsen et al. investigated the association between LYPD3 and tumorigenesis, suggesting a potential role in tumor invasion and metastasis (16). LYPD3 has been demonstrated to influence cancer initiation, progression, and chemoresistance in metastatic cancers by affecting tumor proliferation and apoptosis, which is associated with many important regulatory mechanisms of cancer (17,18). The expression of LYPD3 has been linked to the occurrence of LC and poor prognosis (17,19,20). However, few reports have explored the relationship between LYPD3 and LC, particularly regarding its potential as a biomarker for LC.
In our study, we conducted a comprehensive analysis of various databases to demonstrate the differential expression and prognostic characteristics of LYPD3 in LC. We also investigated its potential biological functions and elucidated its relevance in tumor immune infiltration. Our findings highlight the clinical value and prognostic significance of LYPD3 in LC, providing a theoretical and molecular basis for future clinical and basic research. We present this article in accordance with the REMARK reporting checklist (available at https://tcr.amegroups.com/article/view/10.21037/tcr-23-1557/rc).
Methods
Data gathering and screening
We collected RNA-seq data from 541 LC samples and 59 normal samples from The Cancer Genome Atlas (TCGA) database (https://portal.gdc.cancer.gov/). The Gene Expression Omnibus (GEO) (https://www.ncbi.nlm.nih.gov/geo/) was also used to evaluate the OS of LYPD3 in LC. ExoCarta (http://www.exocarta.org/) was used to screen for exosome molecules in LC. Additionally, the Human Protein Atlas (HPA; https://www.proteinatlas.org/) was utilized to compare the LYPD3 protein expression in normal and tumor tissues in LC by using immunohistochemistry (IHC) data. This study was conducted in accordance with the Declaration of Helsinki (as revised in 2013).
Pan-cancer expression of LYPD3 analysis
The Tumor Immune Estimation Resource (TIMER) database mainly covers 10,897 samples from the TCGA database. We used this database to analyze the pan-cancer expression of LYPD3. The Wilcox test was used to determine whether the difference was significant.
Prognostic analysis
Gene Expression Profiling Interactive Analysis 2 (GEPIA2) database was used to analyze the messenger RNA (mRNA) expression of target genes in tumor and normal tissues interactively (21). This study aimed to determine the relationship between LYPD3 expression and prognosis of LC. The Kaplan-Meier (KM) plotter database is a web-based database that can predict the prognostic characteristics of many cancers (22). We used this database to explore the association between LYPD3 expression and LC survival. The results were displayed using survival curves and log-rank P and hazard ratio (HR).
Functional enrichment analysis
To explore LYPD3-related functional and pathway enrichment, we performed Gene Ontology (GO) and Kyoto Encyclopedia of Genes and Genomes (KEGG) analyses using the R package “Clusterprofiler”. We also performed gene set enrichment analysis (GSEA) using GSEA software (23). The thresholds are set to P value <0.05 and |fold change (FC)| >1.5.
Immune cells infiltration analysis
The relative scores of LYPD3 in 28 subtypes of human immune cells were studied quantitatively by single-sample GSEA (ssGSEA) based on the R package “GSVA”. The fraction of 22 kinds of infiltrating immune cells of LC were also evaluated by using R software package “CIBERSORT”. P value <0.05 means statistically significant.
Therapeutic analysis
The immunophenoscores (IPS) of LC were downloaded from The Cancer Immunome Atlas (TCIA) database (24). Then, the IPS values of high LYPD3 group and low LYPD3 group in different immunotherapy methods were compared to predict immunotherapy sensitivity. The half maximal inhibitory concentration (IC50) values for the most commonly used chemotherapy drugs were calculated using the R package “pRRophetic”. Drug sensitivity was compared between the high LYPD3 group and the low LYPD3 group. The statistically significant differences were tested using the Wilcox test.
Statistical analysis
R software (version 3.6.1) was used for all statistical analyses. Survival curves were plotted by the KM plotter with the log-rank test. Wilcoxon test was used to compare the two groups. Chi-square test was used to analyze the correlation between immune checkpoint inhibitor (ICI) score group and tumor mutational burden (TMB). Spearman analysis was used to calculate the correlation coefficient. P value <0.05 was considered significant.
Results
Screening for exosome molecules LYPD3 that affect the prognosis of LC
To investigate the impact of exosome molecules on LC, we utilized the ExoCarta database to compare 1,769 mRNAs and 5,401 proteins, screening 904 exosome genes (Figure 1A). Subsequent cluster analysis of these 904 genes (Figure 1B,1C) revealed high expression of 116 mRNA and low expression of 38 mRNA [log2FC >1, false discovery rate (FDR) <0.05]. We then selected genes with |log2FC| ≥3 among the differentially expressed genes for prognostic analysis using GEPIA. Our results showed that high expression of LYPD3 was significantly associated with poorer OS (HR =1.9; P value =7.1e−5), indicating that LYPD3 may affect OS in LC, as opposed to other genes (Figure 1D). Further analysis using the GEO database and KM plotter confirmed that high expression of LYPD3 was associated with worse prognosis in LC (HR =1.4; P=2.7e−8) (Figure 1E).
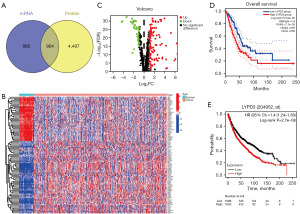
The expression of LYPD3 in LC
We evaluated the expression levels of LYPD3 in multiple human malignancies using samples from the TCGA database. Our analysis revealed that, compared to adjacent normal tissues, LYPD3 was upregulated in most cancers, including BRCA, CHOL, COAD, ESCA, PRAD, LIHC, LUAD, LUSC, READ, SKCMSTAD, and THCA, and downregulated in a few cancers such as HNSC, HNSC-HPVKICH, KIRC, and KIRP (Figure 2A; TCGA study abbreviations: https://gdc.cancer.gov/resources-tcga-users/tcga-code-tables/tcga-study-abbreviations). Immunohistochemical analysis of samples from the HPA database showed high staining of LYPD3 in LC specimens compared to non-LC tissues (Figure 2B). Analysis of 59 normal samples and 541 LC samples from the TCGA database revealed that the expression level of LYPD3 in LC was significantly higher than that in normal tissues (Figure 2C). A paired test on normal tissues and LC tissues from 58 patient samples further confirmed the high expression of LYPD3 in LC (Figure 2D).
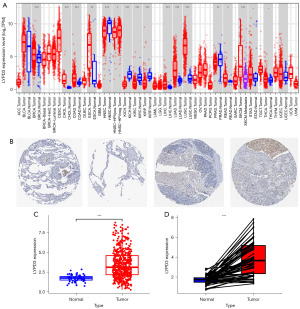
The relationship of LYPD3 with clinical pathological factors
We conducted a statistical analysis of the expression of LYPD3 in clinical pathological factors. Our results showed that upregulation of LYPD3 was associated with clinical stage, T classification, and N classification, but not significantly associated with gender, age, or M classification (Figure 3A). We also investigated the correlation between LYPD3 and the prognosis of LC patient subgroups classified by clinical pathological factors. Univariate Cox analysis revealed that LYPD3 (HR =1.223; P<0.001), clinical stage (HR =1.637; P<0.001), and T classification (HR =1.549; P<0.001) were significantly associated with LC prognosis (Figure 3B). Multivariate Cox analysis confirmed that, after adjusting for other clinical pathological factors, LYPD3 is an independent prognostic factor (Figure 3C).
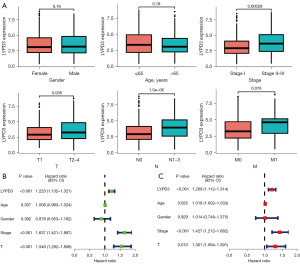
Analysis of co-expressed genes and potential biological functions of LYPD3 in LC
We further screened out 531 positively correlated genes as high LYPD3 expression group, and 1,094 negatively correlated genes as low LYPD3 expression group, based on the median value of LYPD3 mRNA expression (log2FC >1 and FDR <0.05) (Figure 4A). GO analysis revealed that genes co-expressed with LYPD3 were mainly associated with epidermis development, skin development, and organic anion transport (in BP), transporter complex, apical plasma membrane, and transmembrane transporter complex [in cellular component (CC)], channel activity, passive transmembrane transporter activity, and metal ion transmembrane transporter activity [in molecular function (MF)] (Figure 4B,4C). KEGG analysis showed that these genes were primarily related to neuroactive ligand-receptor interaction, MAPK signaling pathway, protein digestion and absorption, and adrenergic signaling in cardiomyocytes (Figure 4D). GSEA analysis of the relationship between LYPD3 co-expressed genes and KEGG pathways revealed that co-expressed genes were mainly related to cell cycle, DNA replication, proteasome, regulation of actin cytoskeleton, small cell LC, spliceosome, and steroid hormone biosynthesis (Figure 4E).
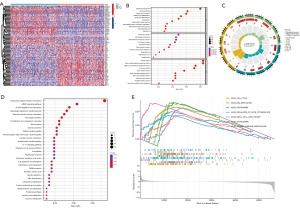
LYPD3 is associated with the degree of immune infiltration in LC
Previous bioinformatics analysis has confirmed a close relationship between LYPD3 and the immune response in LC. To further verify this relationship, we used TIMER to analyze the cell infiltration scores of immune cells in both high and low LYPD3 expression groups. Our results showed that, in the high LYPD3 expression group, cell infiltration in antigen-presenting cell (APC) co-inhibition, CC chemokine receptor (CCR), macrophages, major histocompatibility complex (MHC) class 1, natural killer (NK) cells, and parainflammation was significantly reduced, while cell infiltration in inflammatory dendritic cells (iDCs), mast cells, and T helper cells was significantly increased (Figure 5A). We also used the R package CIBERSORT to compare the distribution of 22 immune cells in the two LYPD3 groups and found that plasma cells, T cell CD4 memory resting, dendritic cells resting, and mast cells resting were more abundant in the low LYPD3 expression group, while T cell CD4 memory activated, NK cells resting, and macrophages M0/M1 were more abundant in the high LYPD3 expression group (Figure 5B). Correlation coefficient analysis of these 22 cells revealed that LYPD3 expression was positively correlated with cell infiltration of macrophages M0/M1, T cell CD4 memory activated, NK cells resting, T cells CD8 and other cells and negatively correlated with cell infiltration of dendritic cells resting, mast cells resting, T cell CD4 memory resting, macrophages M2, NK cells activated and plasma cells (Figure 5C). Further analysis showed that the expression of LYPD3 had a mild but significant correlation with the infiltration of macrophages M0 (R=0.24; P=2.9e−7), T cell CD4 memory activated (R=−0.18; P=0.00018), and dendritic cells resting (R=−0.21; P=1e−5) (Figure 5D-5F).
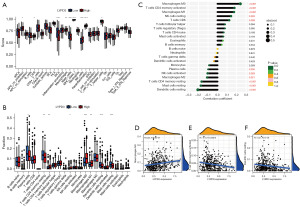
Analysis of immune therapy and drug sensitivity of LYPD3 in LC
Immune therapy, represented by ICIs, has made significant breakthroughs in tumor treatment. Our initial analysis of immune checkpoint-related transcripts revealed that LYPD3 was positively correlated with CD70, TNFSF9, CD276, VTCN1, and TNFSF4 and negatively correlated with CD40LG, TNFSF15, CD200R1, CD160, and BTLA (Figure 6A). We further used the IPS algorithm to evaluate the immune therapy response in the high LYPD3 expression group and low LYPD3 expression group. Our results showed that IPS and IPS-CTLA4 were significantly different between the high LYPD3 expression group and low LYPD3 expression group (both <0.05), while IPS-PD1 (P=0.38) and IPS-CTLA4-PD1 (P=0.25) showed no statistical difference (Figure 6B). Our previous GSEA revealed that DNA damage repair-related pathways were significantly enriched in LYPD3 co-expressed genes. Existing research suggests that abnormalities in DNA damage and repair are directly related to genomic instability. We therefore analyzed the correlation between LYPD3 expression and TMB and found a significant positive correlation between the two (R=0.19; P=1.2e−5) (Figure 6C).

To further investigate the clinical utility of LYPD3 in LC treatment, we evaluated the therapeutic effects of commonly used chemotherapy drugs in the high LYPD3 expression group and low LYPD3 expression group. Our results showed that the median IC50 of bexarotene, cyclopamine, etoposide, and paclitaxel in the high LYPD3 expression group was significantly lower than that in the low LYPD3 expression group (Figure 6D-6G), indicating potential for LYPD3 in immune therapy.
Discussion
LYPD3 is a tumorigenic and highly glycosylated cell surface protein (25), consisting of 278 amino acids distributed in the serine-rich C-terminal region and two N-terminal Lu domains of threonine. Overexpression of LYPD3 has been associated with a malignant phenotype and poor prognosis in colorectal cancer and esophageal squamous cell carcinoma (ESCC), whereas in breast cancer it is associated with a good prognosis (26,27). A few studies have demonstrated that LYPD3 were associated with NSCLC and lung adenocarcinoma carcinogenesis (20,28). There is also strong evidence that LYPD3 could specifically participate in tumor cell invasion through its interaction with extracellular matrix (29). Therefore, the relationship between LYPD3 and the development of LC deserves further study.
Studies have shown that exosomes can affect tumor growth and metastasis, paraneoplastic syndrome, and treatment resistance (30,31). Additionally, exosomes could be used for comprehensive, multi-parameter diagnostic detection and as a therapeutic carrier (32). In our study, we performed exosome screening to obtain 904 associated genes, including LYPD3. Further analysis using OS and KM revealed an association between LYPD3 and LC prognosis. This finding suggests that LYPD3 may have great potential for early diagnosis and treatment of LC.
In this study, we conducted a pan-cancer analysis of LYPD3 expression in LC. Analysis of the HPA database revealed that the LYPD3 protein was significantly more abundant in cancer tissues than in normal counterparts in LC. We also analyzed the clinical-pathological factors of LC and found that LYPD3 was an independent prognostic factor in LC. These results suggest that LYPD3 may serve as a prognostic biomarker for LC.
We conducted gene enrichment analysis of LYPD3 co-expression genes. KEGG analysis revealed that these genes were primarily related to the MAPK signaling pathway, protein digestion and absorption, and adrenergic signaling in cardiomyocytes. GSEA analysis showed that co-expressed genes were mainly related to cell cycle, DNA replication, small cell LC, spliceosome, and steroid hormone biosynthesis, suggesting a close relationship between LYPD3 and the immune response in LC.
Immune cell infiltration has been shown to be an important factor affecting immunotherapy resistance and tumor progression (33). We analyzed the correlation between LYPD3 expression level and immune cell infiltration. As shown in Figure 5, the expression level of LYPD3 was related to the fraction of various immune cells. The expression of LYPD3 had a significant correlation with the infiltration of macrophages M0 (R=0.24; P=2.9e−7), T cell CD4 memory activated (R=−0.18; P=0.00016), and dendritic cells resting (R=−0.21; P=1e−5), indicating that LYPD3 can reflect the infiltration of immune cells in LC.
ICI therapy has been successful in treating various tumors by overcoming tumor cell-mediated loss of immunity, restoring anticancer immunity, and clearing tumor cells (34). In this study, immune checkpoint analysis revealed an association between LYPD3 and multiple checkpoint molecules. Studies have shown that patients with high TMB may have a stronger immune response and be more sensitive to ICIs (35,36). We found a significant positive correlation between LYPD3 expression and TMB (37). Additionally, we found that the median IC50 of bexarotene, cyclopamine, etoposide, and paclitaxel in the LYPD3 high expression group was significantly lower than that in the LYPD3 low expression group. These results suggest that low expression of LYPD3 may be associated with a better prognosis, indicating significant therapeutic potential for LYPD3.
In summary, this study fully demonstrates the value of LYPD3 in the progression of LC and its potential as a biological target and prognostic predictor. The expression of LYPD3 is not only closely related to the prognosis of LC patients but also highly correlated with immune infiltration. Furthermore, LYPD3 may have great application prospects in the immunotherapy of LC.
Conclusions
In conclusion, this study describes the expression characteristics, related pathways, relationship with tumor-infiltrating immune cells, and therapeutic value of LYPD3 in LC from a bioinformatics perspective. LYPD3 has broad potential in predicting the immune microenvironment and immunotherapy response.
Acknowledgments
Funding: This work was supported by
Footnote
Reporting Checklist: The authors have completed the REMARK reporting checklist. Available at https://tcr.amegroups.com/article/view/10.21037/tcr-23-1557/rc
Peer Review File: Available at https://tcr.amegroups.com/article/view/10.21037/tcr-23-1557/prf
Conflicts of Interest: All authors have completed the ICMJE uniform disclosure form (available at https://tcr.amegroups.com/article/view/10.21037/tcr-23-1557/coif). The authors have no conflicts of interest to declare.
Ethical Statement: The authors are accountable for all aspects of the work in ensuring that questions related to the accuracy or integrity of any part of the work are appropriately investigated and resolved. This study was conducted in accordance with the Declaration of Helsinki (as revised in 2013).
Open Access Statement: This is an Open Access article distributed in accordance with the Creative Commons Attribution-NonCommercial-NoDerivs 4.0 International License (CC BY-NC-ND 4.0), which permits the non-commercial replication and distribution of the article with the strict proviso that no changes or edits are made and the original work is properly cited (including links to both the formal publication through the relevant DOI and the license). See: https://creativecommons.org/licenses/by-nc-nd/4.0/.
References
- Sung H, Ferlay J, Siegel RL, et al. Global Cancer Statistics 2020: GLOBOCAN Estimates of Incidence and Mortality Worldwide for 36 Cancers in 185 Countries. CA Cancer J Clin 2021;71:209-49. [Crossref] [PubMed]
- Han D, Li J, Wang H, et al. Circular RNA circMTO1 acts as the sponge of microRNA-9 to suppress hepatocellular carcinoma progression. Hepatology 2017;66:1151-64. [Crossref] [PubMed]
- Sorber L, Zwaenepoel K, Deschoolmeester V, et al. Circulating cell-free nucleic acids and platelets as a liquid biopsy in the provision of personalized therapy for lung cancer patients. Lung Cancer 2017;107:100-7. [Crossref] [PubMed]
- Duma N, Santana-Davila R, Molina JR. Non-Small Cell Lung Cancer: Epidemiology, Screening, Diagnosis, and Treatment. Mayo Clin Proc 2019;94:1623-40. [Crossref] [PubMed]
- Del Rivero J, Enewold L, Thomas A. Metastatic lung cancer in the age of targeted therapy: improving long-term survival. Transl Lung Cancer Res 2016;5:727-30. [Crossref] [PubMed]
- Walia A, Tuia J, Prasad V. Progression-free survival, disease-free survival and other composite end points in oncology: improved reporting is needed. Nat Rev Clin Oncol 2023;20:885-95. [Crossref] [PubMed]
- Strope SA, Andriole GL. Prostate cancer screening: current status and future perspectives. Nat Rev Urol 2010;7:487-93. [PubMed]
- Clausen MM, Langer SW. Improving the prognosis for lung cancer patients. Acta Oncol 2019;58:1077-8. [Crossref] [PubMed]
- Rösel M, Claas C, Seiter S, et al. Cloning and functional characterization of a new phosphatidyl-inositol anchored molecule of a metastasizing rat pancreatic tumor. Oncogene 1998;17:1989-2002. [Crossref] [PubMed]
- van Putten JPM, Strijbis K. Transmembrane Mucins: Signaling Receptors at the Intersection of Inflammation and Cancer. J Innate Immun 2017;9:281-99. [Crossref] [PubMed]
- Jach D, Cheng Y, Prica F, et al. From development to cancer - an ever-increasing role of AGR2. Am J Cancer Res 2021;11:5249-62. [PubMed]
- Gruet M, Cotton D, Coveney C, et al. β2-Adrenergic Signalling Promotes Cell Migration by Upregulating Expression of the Metastasis-Associated Molecule LYPD3. Biology (Basel) 2020;9:39. [Crossref] [PubMed]
- Willuda J, Linden L, Lerchen HG, et al. Preclinical Antitumor Efficacy of BAY 1129980-a Novel Auristatin-Based Anti-C4.4A (LYPD3) Antibody-Drug Conjugate for the Treatment of Non-Small Cell Lung Cancer. Mol Cancer Ther 2017;16:893-904. [Crossref] [PubMed]
- Kriegbaum MC, Jacobsen B, Füchtbauer A, et al. C4.4A gene ablation is compatible with normal epidermal development and causes modest overt phenotypes. Sci Rep 2016;6:25833. [PubMed]
- Upadhyay G. Emerging Role of Lymphocyte Antigen-6 Family of Genes in Cancer and Immune Cells. Front Immunol 2019;10:819. [Crossref] [PubMed]
- Jacobsen B, Muley T, Meister M, et al. Ly6/uPAR-related protein C4.4A as a marker of solid growth pattern and poor prognosis in lung adenocarcinoma. J Thorac Oncol 2013;8:152-60. [Crossref] [PubMed]
- Lamouille S, Xu J, Derynck R. Molecular mechanisms of epithelial-mesenchymal transition. Nat Rev Mol Cell Biol 2014;15:178-96. [Crossref] [PubMed]
- Fischer KR, Durrans A, Lee S, et al. Epithelial-to-mesenchymal transition is not required for lung metastasis but contributes to chemoresistance. Nature 2015;527:472-6. [Crossref] [PubMed]
- Andus I, Prall F, Linnebacher M, et al. Establishment, characterization, and drug screening of low-passage patient individual non-small cell lung cancer in vitro models including the rare pleomorphic subentity. Front Oncol 2023;13:1089681. [Crossref] [PubMed]
- Hu P, Huang Y, Gao Y, et al. Elevated Expression of LYPD3 Is Associated with Lung Adenocarcinoma Carcinogenesis and Poor Prognosis. DNA Cell Biol 2020;39:522-32. [Crossref] [PubMed]
- Tang Z, Kang B, Li C, et al. GEPIA2: an enhanced web server for large-scale expression profiling and interactive analysis. Nucleic Acids Res 2019;47:W556-60. [PubMed]
- Nagy Á, Lánczky A, Menyhárt O, et al. Validation of miRNA prognostic power in hepatocellular carcinoma using expression data of independent datasets. Sci Rep 2018;8:9227. [Crossref] [PubMed]
- Subramanian A, Tamayo P, Mootha VK, et al. Gene set enrichment analysis: a knowledge-based approach for interpreting genome-wide expression profiles. Proc Natl Acad Sci U S A 2005;102:15545-50. [Crossref] [PubMed]
- Charoentong P, Finotello F, Angelova M, et al. Pan-cancer Immunogenomic Analyses Reveal Genotype-Immunophenotype Relationships and Predictors of Response to Checkpoint Blockade. Cell Rep 2017;18:248-62. [Crossref] [PubMed]
- Hansen LV, Gårdsvoll H, Nielsen BS, et al. Structural analysis and tissue localization of human C4.4A: a protein homologue of the urokinase receptor. Biochem J 2004;380:845-57. [Crossref] [PubMed]
- Ohtsuka M, Yamamoto H, Masuzawa T, et al. C4.4A expression is associated with a poor prognosis of esophageal squamous cell carcinoma. Ann Surg Oncol 2013;20:2699-705. [Crossref] [PubMed]
- Wang G, Guo S, Zhang W, et al. Co-expression network analysis identifies key modules and hub genes implicated in esophageal squamous cell cancer progression. Medicine in Omics 2021;1:100003.
- Zhao F, Tian H, Liu X, et al. Homeobox A1 Facilitates Immune Escape and Alleviates Oxidative Stress in Lung Adenocarcinoma. Oxid Med Cell Longev 2022;2022:4102666. [Crossref] [PubMed]
- Paret C, Hildebrand D, Weitz J, et al. C4.4A as a candidate marker in the diagnosis of colorectal cancer. Br J Cancer 2007;97:1146-56. [Crossref] [PubMed]
- Zhang L, Yu D. Exosomes in cancer development, metastasis, and immunity. Biochim Biophys Acta Rev Cancer 2019;1871:455-68. [Crossref] [PubMed]
- Zhu L, Sun HT, Wang S, et al. Isolation and characterization of exosomes for cancer research. J Hematol Oncol 2020;13:152. [Crossref] [PubMed]
- Kalluri R, LeBleu VS. The biology, function, and biomedical applications of exosomes. Science 2020;367:eaau6977. [Crossref] [PubMed]
- Shimizu K, Iyoda T, Okada M, et al. Immune suppression and reversal of the suppressive tumor microenvironment. Int Immunol 2018;30:445-54. [Crossref] [PubMed]
- Darvin P, Toor SM, Sasidharan Nair V, et al. Immune checkpoint inhibitors: recent progress and potential biomarkers. Exp Mol Med 2018;50:1-11. [Crossref] [PubMed]
- Samstein RM, Lee CH, Shoushtari AN, et al. Tumor mutational load predicts survival after immunotherapy across multiple cancer types. Nat Genet 2019;51:202-6. [Crossref] [PubMed]
- Jardim DL, Goodman A, de Melo Gagliato D, et al. The Challenges of Tumor Mutational Burden as an Immunotherapy Biomarker. Cancer Cell 2021;39:154-73. [Crossref] [PubMed]
- Klempner SJ, Fabrizio D, Bane S, et al. Tumor Mutational Burden as a Predictive Biomarker for Response to Immune Checkpoint Inhibitors: A Review of Current Evidence. Oncologist 2020;25:e147-59. [Crossref] [PubMed]