The activation of HAMP promotes colorectal cancer cell proliferation through zinc-mediated upregulation of SMAD4 expression
Highlight box
Key findings
• Analysis of The Cancer Genome Atlas-colorectal cancer (CRC) RNA data identified an overlap between prognostically relevant genes and 829 zinc metabolism-related genes in CRC, suggesting that zinc metabolism may be prognostically relevant in CRC. The protein interaction network and functional enrichment of metal ion homeostasis, zinc transport, zinc homeostasis, NRF2 pathway, and Ferroptosis supported this association. The study reveals a potential role for zinc metabolism in CRC prognosis.
What is known and what is new?
• Prognostic genes and zinc metabolism are individually studied in CRC.
• This study uniquely identified key genes, particularly HAMP, linking zinc metabolism and prognosis in CRC, providing novel insights into their functional roles.
What is the implication, and what should change now?
• Implications: the study underscores the clinical relevance of HAMP in CRC prognosis and highlights potential therapeutic avenues targeting zinc metabolism.
• Actions needed: further studies are warranted to elucidate the detailed mechanisms and validate these findings in clinical settings, paving the way for the development of targeted therapies in CRC.
Introduction
Colorectal cancer (CRC) is one of the most common cancers of the gastrointestinal tract and is the most common malignant tumor in the digestive system, and tumor metastasis is the main cause of death in patients with CRC in clinical practice (1). Presently, the standard therapy for CRC is surgery combined with adjuvant chemotherapy or radiotherapy (2). An important aspect of improving patient outcomes is the early diagnosis of primary or recurrent CRC (3). In spite of significant advances made in the early diagnosis and treatment, problems like poor survival, high recurrence rates, and suboptimal prognosis still pose a major threat to CRC patients (4,5). Recent study had demonstrated that Pembrolizumab exhibits durable clinical benefits, including long-lasting anti-tumor activity and prolonged survival, with a manageable safety profile in previously treated advanced or metastatic microsatellite instability-high (MSI-H)/mismatch repair-deficient (dMMR) CRC (6). Study had shown that exosomes promote macrophage phenotypic changes and tumor metastasis in the CRC tumor microenvironment. Specifically, miR-372-5p in CRC cell exosomes induces M2 macrophages to secrete the chemokine CXCL12, which activates the WNT/β-catenin pathway and promotes epithelial-to-mesenchymal transition (EMT), stemness and metastatic ability of CRC cells (7). New ideas for the clinical management of CRC patients in these studies, but also lay the foundation for future research in related fields. As a result, it is urgent to explore new diagnostic, prognostic indicators, and treatment targets, as well as to research the molecular causes of CRC. For example, in CRC, DDX3 is recognized as a potential oncogene. A study had shown that deletion of DDX3 in advanced cancers promotes cancer progression by regulating the MAPK pathway, which in turn promotes cancer progression, especially by affecting E-cadherin and β-catenin signaling (8). On the other hand, the findings of TRPV3, a tumor suppressor and prognostic marker, suggest that the MAPK signaling pathway plays a key role in TRPV3 silencing-mediated CRC progression (9). This suggested that the exploration of new diagnostic and prognostic indicators, as well as in-depth study of the molecular etiology of CRC, is crucial for future drug development and improving patient prognosis.
For many aspects of life, zinc is a trace element that is necessary (10,11). It is essential for the transcription of genes since it is a structural element of the zinc finger motif found in several transcription factors and a catalytic co-factor for numerous significant enzymes, such as RNA polymerases (12-14). According to the significance of zinc for cellular metabolism, zinc shortage has various negative effects (15,16). For instance, even a mild zinc shortage in animals can result in anemia, appetite loss, immune system inadequacies, developmental issues, and abnormalities (17). Nevertheless, excessive zinc can also be detrimental to cellular functions (18). Therefore, it is essential to closely regulate the cytoplasmic concentration of labile zinc that is weakly bound.
Zinc is a crucial mineral for immunological and antioxidant responses, apoptosis, and mental health, as well as for the activation or stability of a vast number of enzymes and transcription factors (19). Recently, the relation between zinc metabolism and human diseases has attracted much attention. The maintenance of the balance of zinc metabolism in the body depends mainly on the regulation of the excretory action of the small intestine. That is, when the body consumes too much zinc, the secretion of zinc from the liver and pancreas to the small intestine increases, resulting in an increase in the excretion of endogenous zinc. Zinc has been shown to have anticancer properties through immune system regulation (20), transcription factors, DNA and RNA synthesis and repair (19), stability of cell structure and membranes, control of enzyme activation or suppression of cell signaling. Khoshdel et al. found that zinc levels in the blood of CRC patients were reduced compared to controls through a clinical study (21).
Since zinc possesses multiple functions, although the mechanism of its involvement is not entirely known, it may play a significant part in the promotion of CRC and protection against it. Thus, our study integrated bioinformatics analysis involving zinc metabolism-related genes and crucial prognostic genes in CRC from The Cancer Genome Atlas (TCGA), identified HAMP as a significant gene of interest. We subsequently conducted in vitro cellular assays to further study the mechanism of HAMP in CRC. We present this article in accordance with the TRIPOD reporting checklist (available at https://tcr.amegroups.com/article/view/10.21037/tcr-23-1292/rc).
Methods
Data acquisition
The RNA sequencing (RNAseq) data of CRC and its clinical data were from the TCGA (https://portal.gdc.com/). R software was used for gene-wide batch survival analysis of CRC to screen for significant prognostic genes. Genes related to zinc metabolism were acquired from the Gene Set Enrichment Analysis (GSEA; https://www.gsea-msigdb.org/gsea/index.jsp) website. Venn diagrams of zinc metabolism-related genes and genes with prognostic significance (P<0.05) in CRC were drawn using Venn Diagram Online to screen the overlapping genes.
Protein-protein interaction (PPI) network and functional enrichment analysis of overlapping genes
The Search Tool for the Retrieval of Interacting Genes (STRING; http://string-db.orgnewstring_cgi) database was applied to predict and construct PPI network between overlapping genes (22). Then, functional enrichment analysis on genes with interconnectivity in the PPI network was conducted by the Database for Annotation, Visualization and Integrated Discovery (DAVID) database to provide a functional overview of the overlapping genes. Gene ontology (GO) involved biological process (BP), cellular component (CC), and molecular function (MF), providing biological function explanations for genes. Kyoto Encyclopedia of Gene and Genome (KEGG) by WikiPathway analyzed and visualized data in a curated, machine-readable format to elucidate how genes with interconnections in the PPI network function through specific pathways.
Construction of the prognostic nomogram
The expression of the five connected genes in the PPI network in the CRC samples and normal control samples of the TCGA database was tested, and the results of P<0.05 were considered statistically significant. Univariate Cox proportional hazards regression analysis was used to compare the prognostic values of the above significantly expressed genes and clinical features of CRC like age, gender, and pN-stage. Moreover, multivariate Cox proportional hazards regression analysis was used to assess which prognostic gene or clinical characteristics could be the promising prognostic indicators (P<0.05). In order to create a more customized prediction model, a prognostic nomogram incorporating independent prognostic factors was constructed to screen the key genes in CRC. The predictive power of the nomogram was assessed by the calibration curve.
Expression and clinical feature analysis on HAMP
To conduct the analysis, data from TCGA database were subsequently processed and analyzed for HAMP gene expression levels. Subsequently, we conducted a comparative analysis of HAMP expression across various clinical samples. The subgroups we selected for our study encompassed individuals of different cancer stages, ranging from stage 1 to stage 4. Furthermore, we stratified our dataset based on the patient’s gender, age, nodal metastasis status, and TP53 mutation status, in order to identify any potential correlations or trends between these parameters and the HAMP expression level. The statistical analysis was carried out using a suitable statistical software. For all the analysis, a P value of less than 0.05 was set as statistically significant.
Cell culture and treatment
Both CRC cell lines (RKO, HCT-116, SW480, SW620) and immortalized normal intestinal epithelial cell lines (FHC) were purchased from the Chinese Academy of Sciences’ Culture Collection Center (Shanghai, China) and cultured in Dulbecco’s Modified Eagle Medium (DMEM) supplemented with 10% fetal bovine serum (FBS), 100 U/mL penicillin, and 100 µg/mL streptomycin at 37 ℃ in a humidified atmosphere of 5% CO2. For specific experiments, the CRC cells were treated with a 15 µM concentration of zinc sulfate (a zinc supplement), or a 3 µM dose of TPEN (a potent zinc chelator). The treated cells were cultured for 24 hours prior to subsequent experiments.
Cell transfection
Specific small hairpin RNAs (shRNAs) targeting HAMP and a vector for SMAD4 overexpression were introduced into the CRC cells via transfection. Transfections were conducted using Lipofectamine 2000, a commonly used reagent for transfection, strictly. After the transfection was done, cells were incubated for 48 hours to ensure sufficient expression knockdown or overexpression before they were used for further analysis. The efficiency of HAMP knockdown and SMAD4 overexpression was tested by quantitative real-time polymerase chain reaction (qRT-PCR) and western blotting (WB).
qRT-PCR
Following the manufacturer’s recommendations, total RNA was isolated from cells using TRIzol reagent. The SuperScript III First-Strand Synthesis System for RT-PCR was used in accordance with the manufacturer’s instructions to create complementary DNA (cDNA). On a StepOnePlus Real-Time PCR System, qRT-PCR was carried out using a SYBR Green PCR Master Mix. Primers specific for HAMP, SMAD4, and glyceraldehyde-3-phosphate dehydrogenase (GAPDH) (used as an internal control) were utilized: HAMP forward, 5'-CTGCAACCCCAGGACAGAG-3', reverse, 5'-GGAATAAATAAGCAAGGGAGGGG-3'; SMAD4 forward, 5'-ACACCAACAAGTAACGATGCC-3', reverse, 5'-GCAAAGGTTTCACTTTCCCCA-3'; GAPDH forward, 5'-TGAGCAAGAGAGGCCCTATCC-3'; reverse, 5'-CCCTCCTGTTATTATGGGGGTCT-3'. Relative gene expression was computed using the 2−ΔΔCt method. Furthermore, the changes in relative HAMP and SMAD4 expression levels in CRC cell lines pre- and post-treatment with zinc sulfate or TPEN were quantified using qRT-PCR.
WB assay
Protease inhibitors were added to reversible interactions of proteins with the radioimmunoprecipitation assay (RIPA) buffer, which was used to lyse the cells. The bicinchoninic acid (BCA) protein assay kit was used to calculate the protein concentration. Sodium dodecyl sulfate-polyacrylamide gel electrophoresis (SDS-PAGE) was used to separate equal quantities of protein, which were then transferred to polyvinylidene fluoride (PVDF) membranes and blocked with 5% non-fat dry milk. Membranes were then probed with primary antibodies specific for HAMP, SMAD4, and GAPDH and the appropriate secondary antibodies coupled to horseradish peroxidase. The increased chemiluminescence method was used to visualize bands. WB was used to validate the results of qRT-PCR at the protein level. Changes in HAMP and SMAD4 expression under different conditions were monitored, as well as the efficiency of HAMP knockdown and SMAD4 overexpression. After SMAD4 overexpression and zinc sulfate treatment, changes in HAMP protein levels were also evaluated by WB.
Transwell assay
To estimate the effect of HAMP knockdown on CRC cell migration and invasion, a Transwell assay was carried out. Cells were seeded into the upper chamber of Transwell inserts (Corning Inc., Corning, NY, USA; 8 µM pore size) with or without Matrigel coating for migration or invasion assays, respectively. 10% FBS was added to the medium in the lower chamber to function as a chemoattractant. Non-migrating or non-invading cells in the top chamber were removed using a cotton swab after incubation for 24 to 48 hours. Invading or migrating cells were counted under a microscope in five random fields after being fixed in methanol, labeled with 0.1% 4',6-diamidino-2-phenylindole (DAPI), and moved to the bottom side of the membrane.
Cell counting kit-8 (CCK-8) assay
The CCK-8 test was used to evaluate CRC cell growth. Cells were seeded in 96-well plates and allowed to attach overnight. At designated time points, each well received 10 L of CCK-8 solution, which was then incubated for 1–2 hours at 37 ℃. The absorbance at 450 nm was observed by a microplate reader, with the cell proliferation rate being directly proportional to the absorbance.
Luciferase reporter assay
To monitor HAMP promoter activity, a luciferase reporter construct carrying the wild-type (WT) HAMP promoter was generated, along with constructs with the deletion of mutant type (MUT)1 and MUT2 variants. These constructs were transfected into CRC cells by Lipofectamine 2000. Following transfection, cells were treated with a 15 µM concentration of zinc sulfate, and incubated for 24 hours. Using a dual-luciferase reporter assay setup, luciferase activity was then evaluated after cell harvesting, with Renilla luciferase serving as a normalization control.
Statistical analysis
GraphPad Prism 8.0 was used to conduct the statistical analysis. The information was displayed as the mean and standard deviation (SD) of at least three separate studies. The two-tailed Student’s t-test was used to compare two groups, while Tukey’s post-hoc test and one-way analysis of variance (ANOVA) were employed to compare multiple groups. The relative gene and protein expression levels in qRT-PCR and WB experiments were analyzed using the 2−ΔΔCt method and densitometry, respectively. For the Transwell and CCK-8 assays, cell counts and absorbance readings were statistically compared to evaluate differences in cell migration, invasion, and proliferation. For the luciferase reporter assay, changes in luciferase activity reflecting alterations in HAMP promoter activity were statistically compared. The correlations between HAMP and SMAD4 expression levels and clinical characteristics of CRC patients were analyzed using the chi-squared test. Statistical significance was defined as a P value <0.05.
Ethical considerations
The present study has obtained consent from the Ethics Committee of Minhang Hospital, Fudan University. The study was conducted in accordance with the Declaration of Helsinki (as revised in 2013).
Results
Identification of zinc metabolism-related genes associated with prognosis in CRC
A comprehensive survival analysis of RNA data from TCGA-CRC was performed using genome-wide batches, revealing 598 genes of prognostic significance. Figure 1A shows the top 20 prognostic genes. A total of 829 genes related to zinc metabolism were obtained from the GSEA website. Intersection of this panel with 598 genes of prognostic significance using an online Venn diagram tool identified 31 overlapping genes (Figure 1B). Following this, we constructed a PPI network consisting of 31 overlapping genes using the STRING database. The resulting network involved 31 nodes and eight edges. Notably, five genes were interrelated in this network: SLC39A14, SLC39A8, SLC30A9, SLC39A13, and HAMP (Figure 1C). These findings suggest a potential functional association between these five zinc metabolism-related genes and prognosis in CRC, and thus warrant further investigation.
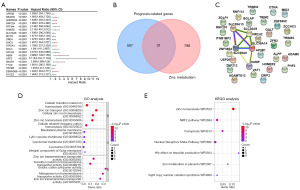
Functional enrichment analysis of key zinc metabolism-related genes in CRC
To elucidate the potential biological functions of the five identified genes (SLC39A14, SLC39A8, SLC30A9, SLC39A13, and HAMP) in CRC, we utilized the DAVID database to conduct enrichment analyses. In the GO enrichment analysis, the BP, CC, and MF categories highlighted several enriched terms. These included cellular transition metal ion homeostasis, zinc ion transport, basolateral plasma membrane, lytic vacuole membrane, zinc ion transmembrane transporter activity, indicating these genes’ roles in metal ion homeostasis and transportation, particularly for zinc ions, in CRC (Figure 1D). Furthermore, KEGG pathway enrichment analysis demonstrated that these genes were significantly enriched in several WikiPathways, including zinc homeostasis, NRF2 pathway, ferroptosis, nuclear receptors meta-pathway, HFE effect on hepcidin production, iron metabolism in placenta, and 3q29 copy number variation syndrome (Figure 1E). This suggested their involvement in various vital cellular processes and pathways.
Expression analysis of key zinc metabolism-related genes in CRC
Subsequently, we examined the expression of the five identified key genes (SLC39A14, SLC39A8, SLC30A9, SLC39A13, and HAMP) in CRC samples and normal samples from the TCGA database. As illustrated in Figure 2A, HAMP and SLC39A13 were significantly overexpressed in CRC samples, while the other three genes did not show a significant difference in expression. Among these, previous research has established HAMP as an immune-related prognostic biomarker in CRC. However, the precise regulatory mechanism of this gene in CRC remains unclear. Based on these findings and the significant clinical relevance of HAMP in CRC, we selected HAMP as the hub gene for further exploration in our study. This choice aims to elucidate its role and regulatory mechanism in CRC, thus providing potential insights for prognosis prediction and therapeutic strategy development in CRC.
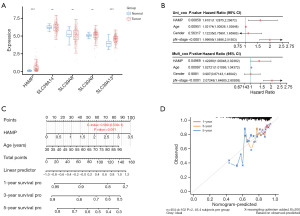
The prognostic value of HAMP and its association with clinical parameters in CRC
Following the application of univariate and multivariate Cox proportional hazards regression models on HAMP and various clinical variables, it was found that HAMP and age emerged as independent prognostic factors for CRC (Figure 2B). Consequently, a nomogram integrating HAMP and age was developed to predict the 1-, 3-, and 5-year overall survival (OS) of CRC patients (Figure 2C). The calibration plot for patient survival prediction displayed a favorable match between the expected and actual outcomes of the gene prognostic nomogram (Figure 2D), suggesting that HAMP may be used independently to predict a patient’s OS in the case of CRC. Subsequently, the chi-squared test revealed a significant overexpression of HAMP in CRC, indicating that its expression levels were influenced by individual cancer stages. However, HAMP expression was found to be unrelated to the patient’s gender, age, nodal metastasis status, and TP53 mutation status, implying its potential as a stage-specific biomarker in CRC (Figure 3A-3F).
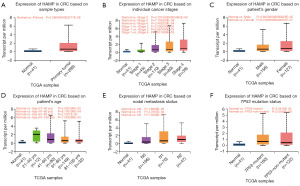
HAMP knockdown inhibited cell migration and invasion in CRC
The relative levels of HAMP in CRC cell lines and normal intestinal epithelial cells were examined using qRT-PCR and WB. Our results revealed a higher level of HAMP in CRC cell lines, particularly in RKO and HCT-116 cells (Figure 4A). Following this, knockdown experiments were performed on these two cell lines targeting HAMP. The knockdown efficiency was estimated by qRT-PCR and WB, which indicated a better efficiency for small interfering (si)-HAMP#1 (Figure 4B). Subsequently, Transwell assays demonstrated that after HAMP knockdown, there was a significant decrease in the number of migrated and invaded RKO and HCT-116 cells (Figure 4C-4F). And flow cytometry assay showed that the apoptosis rate of RKO and HCT-116 cells was significantly increased after knockdown of HAMP (Figure 4G). These findings indicated HAMP might play a critical role in promoting cell invasion in CRC, highlighting its potential as a therapeutic target in CRC management.
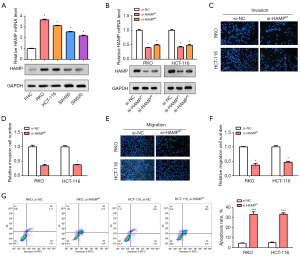
Zinc activated HAMP transcription to promote the proliferation of CRC cells
Zinc supplements (ZnSO4) and zinc chelator (TPEN) (23,24), are known for their regulatory roles in zinc homeostasis within cells, thereby impacting various cellular processes like proliferation and gene expression. In this study, we used these compounds to investigate their effects on CRC cells. qRT-PCR and WB revealed the increased levels of HAMP messenger RNA (mRNA) in CRC cells following treatment with 15 µM zinc supplement ZnSO4 (Figure 5A,5B). In contrast, when CRC cells were treated with 3 µM TPEN, the expression level of HAMP was reduced by qRT-PCR and WB (Figure 5C,5D). These results suggested that zinc ions can positively regulate HAMP expression, and TPEN-induced zinc deprivation may downregulate HAMP. Subsequently, using the CCK-8 assay, we observed a significant increase in cell proliferation of HCT-116 and RKO cells treated with ZnSO4. However, this ZnSO4-induced cell proliferation was attenuated by HAMP knockdown, implying that HAMP might mediate the proliferation-promoting effect of Zn ions (Figure 5E,5F). Conversely, TPEN treatment resulted in inhibition of CRC cell proliferation, and this inhibition was further enhanced in HAMP-knockdown cells (Figure 5G,5H). This suggests that zinc deficiency may inhibit cell proliferation by regulating HAMP expression. Finally, a luciferase reporter assay revealed increased activity of the HAMP promoter in CRC cell lines treated with 15 µM ZnSO4, suggesting that zinc ions may enhance HAMP expression at the transcriptional level (Figure 5I). This provided further insight into the regulatory mechanism of HAMP expression in CRC cells and highlighted the importance of zinc homeostasis in CRC biology.
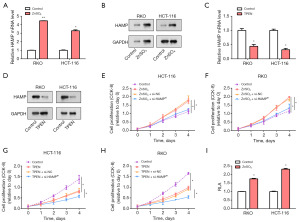
ZnSO4 increased SMAD4 expression to stimulate HAMP promoter activity and enhance CRC cell proliferation
The role of SMAD4 in CRC had been widely studied, given its importance in the regulation of various biological functions. We found that, following treatment with 15 µM ZnSO4, qRT-PCR detected an upregulation in the relative levels of SMAD4 in RKO and HCT-116 cells (Figure 6A). Further, WB results indicated an increase in the nuclear levels of SMAD4, while no significant change was observed in cytoplasmic SMAD4 levels, suggesting a zinc-mediated nuclear translocation of SMAD4 (Figure 6B). Subsequently, SMAD4 overexpression was carried out in CRC cells, leading to a significant increase in SMAD4 levels, confirming the effectiveness of our transfection protocol (Figure 6C,6D). To explore the effect of ZnSO4 on SMAD4 expression, we performed WB assays, which demonstrated that the protein levels of SMAD4 were enhanced following ZnSO4 treatment (Figure 6E). In the next set of experiments, a luciferase reporter construct bearing the WT HAMP promoter, as well as constructs with deletion of MUT1 and MUT2 variants were employed (Figure 6F). The luciferase reporter assay evaluated the transcriptional activity of the HAMP promoter post SMAD4 overexpression and ZnSO4 treatment. We observed an increase in promoter activity in WT, MUT2, and MUT1+2 constructs, while no significant change was observed in the MUT1 construct (Figure 6G,6H). This finding suggested a specific sequence in the MUT1 region is crucial for the zinc-mediated SMAD4 effect on HAMP transcription. Finally, the results of the CCK-8 assay found that CRC cell proliferation was significantly enhanced after combined treatment with SMAD4 overexpression, ZnSO4, and si-HAMP (Figure 6I,6J). This underscored a possible synergistic role of zinc, SMAD4, and HAMP in promoting the proliferation of CRC cells.
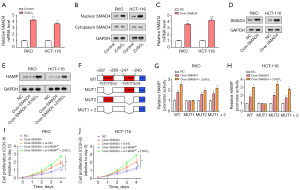
Discussion
CRC is a frequently-seen gastrointestinal tumor with the main clinical features of high mortality and morbidity (25,26). Currently, there are still issues with the diagnosis of CRC like low time efficiency and poor specificity (27). Study reporting focusing on immune populations have found that tumor mutation load status in human CRC contributes to different patterns of patient immune profiles (28). Therefore, finding new and effective clinical biomarkers for the CRC patients is crucial. With the continued exploration of metabolic heterogeneity in cancers, zinc metabolism genes have attracted more and more attention (29,30). It is increasingly important to identify metabolically relevant gene-biased and dependent mechanisms in tumor regulation. Therefore, in this study, we used bioinformatics approaches to study the clinical roles of zinc metabolism-related genes in CRC.
In our investigation, we identified five key zinc metabolism-related genes: SLC39A14, SLC39A8, SLC30A9, SLC39A13, and HAMP, which exhibited potential functional association with CRC prognosis. These genes appeared to have a notable interaction in the constructed PPI network, suggesting a complex mechanism underpinning the impact of zinc metabolism on CRC prognosis. To better understand these genes’ roles in CRC, functional enrichment analyses were conducted. GO enrichment analysis results highlighted their involvement in crucial cellular processes, such as metal ion homeostasis and zinc ion transport. This suggests that the alteration in zinc metabolism could influence CRC progression, possibly through disruption of homeostasis and transportation of zinc ions. Furthermore, KEGG pathway enrichment analysis implicated these genes in vital cellular pathways, including zinc homeostasis, NRF2 pathway, ferroptosis, nuclear receptors meta-pathway, among others. The findings of Knoell et al. suggest that zinc homeostasis imbalance increases susceptibility to permanent lung injury (31). Specific zinc transporter proteins and zinc-binding proteins work together to maintain the dynamic balance of zinc metabolism in the intestine and to maintain zinc homeostasis in the body by regulating zinc absorption and excretion (32). But research into whether zinc is a potential adjuvant for CRC treatment is still unknown. Study had shown that NRF2 signaling pathway contributes to chemo/radioresistance in certain cancers. O’Cathail et al. indicated NRF2 appears to regulate key metabolic pathways in rectal cancer that could be used for therapeutic intervention (33). As ligand-activated transcription factors, nuclear receptors are engaged in physiological processes like growth, homeostasis, differentiation, development and metabolism (34,35). A number of human disorders like cancers are influenced by dysregulation of nuclear receptor signaling (36). The findings of Triki et al. suggest that nuclear receptors may be related to the regulation of CRC cell proliferation (37). This reinforces the notion that zinc metabolism might impact CRC prognosis through multifarious cellular processes and pathways. The intricate interplay between zinc metabolism and these processes could present novel therapeutic targets for CRC. However, additional research is required to unravel the specific mechanisms.
In the context of CRC, we specifically studied the expression of five zinc metabolism-related genes. Among these, HAMP and SLC39A13 were significantly overexpressed in CRC samples. HAMP overexpression promoted CRC development and was associated with tumor mutational burden (TMB), DNA MMR genes, and MSI. Finally, they found that in CRC patients, low HAMP expression also represented better immunotherapy outcomes, reflecting the critical role of HAMP in directing immunotherapy (38). And as HAMP is already acknowledged as an immune-related prognostic biomarker in CRC (39), its precise regulatory mechanism within CRC remains largely unexplored. Given its clinical significance, we focused on HAMP as the hub gene for further investigation, aiming to illuminate its role and regulation within CRC and offer potential insights into prognosis prediction and therapeutic strategy development for CRC. Our analysis revealed HAMP and age as independent prognostic factors for CRC, allowing us to create a nomogram for predicting patient survival. The subsequent calibration plot showed a strong alignment between predicted and actual outcomes, further endorsing HAMP as an independent prognostic factor for CRC. Intriguingly, while HAMP overexpression was linked to cancer stages, there was no correlation found with gender, age, nodal metastasis status, or TP53 mutation status, suggesting the potential of HAMP as a stage-specific biomarker for CRC. Our findings indicated the need for continued research to exploit the potential of HAMP in CRC prognosis and treatment.
Subsequent in vitro studies indicated that HAMP overexpressed in CRC cell lines might play an important role in promoting cell invasion, establishing its potential as a therapeutic target. Knockdown experiments further confirmed that HAMP inhibition significantly reduced cell invasion, mainly in RKO and HCT-116 cells, adding credibility to its critical role in CRC. To extend the study, we carefully examined the effect of ZnSO4 on HAMP expression and CRC cell proliferation. Both qRT-PCR and WB confirmed that ZnSO4 increased the expression of HAMP, while TPEN decreased it. Notably, ZnSO4-enhanced cell proliferation was significantly inhibited by HAMP knockdown, suggesting that HAMP mediates the proliferation-promoting effect of Zn ion. Correspondingly, TPEN inhibited cell proliferation, an effect amplified by HAMP knockdown, suggesting a possible link between zinc deficiency, HAMP expression, and CRC cell proliferation. Next, we explored the effect of ZnSO4 on SMAD4 (40) expression based on the previous research (41,42), and interestingly, ZnSO4 stimulated the nuclear translocation of SMAD4, thereby increasing its protein level. Further, luciferase reporter assays revealed a positive effect of ZnSO4 on HAMP transcriptional activity mediated by SMAD4. This evidence reveals a novel mechanism by which the ZnSO4-SMAD4-HAMP axis enhances CRC cell proliferation. Combined treatment of SMAD4 overexpression, ZnSO4, and si-HAMP significantly promoted CRC cell proliferation, suggesting a synergistic role of Zn, SMAD4, and HAMP in CRC proliferation. These insights highlighted potential pathways for CRC management strategies, focusing primarily on the ZnSO4-SMAD4-HAMP regulatory network.
Conclusions
In conclusion, our study had highlighted the integral role of HAMP in CRC pathogenesis, and further demonstrated the intricate influence of zinc homeostasis and SMAD4 overexpression on HAMP transcription and CRC cell proliferation. That was the activation of HAMP promotes CRC cell proliferation through zinc-mediated upregulation of SMAD4 expression. The precise molecular mechanisms, particularly the synergistic interaction of ZnSO4, SMAD4, and HAMP, underscore the complex gene regulatory networks operative in CRC. Moreover, this study suggested the potential clinical utility of HAMP as a prognostic biomarker and its implications in the development of new therapeutic strategies for CRC. However, further research is warranted to unravel these regulatory mechanisms and validate the therapeutic potential of targeting HAMP in CRC management.
Acknowledgments
Funding: The study was supported by
Footnote
Reporting Checklist: The authors have completed the TRIPOD reporting checklist. Available at https://tcr.amegroups.com/article/view/10.21037/tcr-23-1292/rc
Peer Review File: Available at https://tcr.amegroups.com/article/view/10.21037/tcr-23-1292/prf
Conflicts of Interest: All authors have completed the ICMJE uniform disclosure form (available at https://tcr.amegroups.com/article/view/10.21037/tcr-23-1292/coif). The authors have no conflicts of interest to declare.
Ethical Statement: The authors are accountable for all aspects of the work in ensuring that questions related to the accuracy or integrity of any part of the work are appropriately investigated and resolved. The present study has obtained consent from the Ethics Committee of Minhang Hospital, Fudan University. The study was conducted in accordance with the Declaration of Helsinki (as revised in 2013).
Open Access Statement: This is an Open Access article distributed in accordance with the Creative Commons Attribution-NonCommercial-NoDerivs 4.0 International License (CC BY-NC-ND 4.0), which permits the non-commercial replication and distribution of the article with the strict proviso that no changes or edits are made and the original work is properly cited (including links to both the formal publication through the relevant DOI and the license). See: https://creativecommons.org/licenses/by-nc-nd/4.0/.
References
- Poursheikhani A, Abbaszadegan MR, Nokhandani N, et al. Integration analysis of long non-coding RNA (lncRNA) role in tumorigenesis of colon adenocarcinoma. BMC Med Genomics 2020;13:108. [Crossref] [PubMed]
- Li ZP, Liu XY, Kao XM, et al. Clinicopathological characteristics and prognosis of colorectal mucinous adenocarcinoma and nonmucinous adenocarcinoma: a surveillance, epidemiology, and end results (SEER) population-based study. Ann Transl Med 2020;8:205. [Crossref] [PubMed]
- Tabernero J, Yoshino T, Cohn AL, et al. Ramucirumab versus placebo in combination with second-line FOLFIRI in patients with metastatic colorectal carcinoma that progressed during or after first-line therapy with bevacizumab, oxaliplatin, and a fluoropyrimidine (RAISE): a randomised, double-blind, multicentre, phase 3 study. Lancet Oncol 2015;16:499-508. [Crossref] [PubMed]
- Ramphal W, Boeding JRE, Gobardhan PD, et al. Oncologic outcome and recurrence rate following anastomotic leakage after curative resection for colorectal cancer. Surg Oncol 2018;27:730-6. [Crossref] [PubMed]
- Wu ZF, Lee MS, Wong CS, et al. Propofol-based Total Intravenous Anesthesia Is Associated with Better Survival Than Desflurane Anesthesia in Colon Cancer Surgery. Anesthesiology 2018;129:932-41. [Crossref] [PubMed]
- Le DT, Diaz LA Jr, Kim TW, et al. Pembrolizumab for previously treated, microsatellite instability-high/mismatch repair-deficient advanced colorectal cancer: final analysis of KEYNOTE-164. Eur J Cancer 2023;186:185-95. [Crossref] [PubMed]
- Shi X, Wei K, Wu Y, et al. Exosome-derived miR-372-5p promotes stemness and metastatic ability of CRC cells by inducing macrophage polarization. Cell Signal 2023;111:110884. [Crossref] [PubMed]
- Shen L, Zhang J, Xu M, et al. DDX3 acts as a tumor suppressor in colorectal cancer as loss of DDX3 in advanced cancer promotes tumor progression by activating the MAPK pathway. Int J Biol Sci 2022;18:3918-33. [Crossref] [PubMed]
- Yu W, Huang J, Yu H, et al. TRPV3 inhibits colorectal cancer cell proliferation and migration by regulating the MAPK signaling pathway. J Gastrointest Oncol 2022;13:2447-57. [Crossref] [PubMed]
- Hassan S, Hassan FU, Rehman MS. Nano-particles of Trace Minerals in Poultry Nutrition: Potential Applications and Future Prospects. Biol Trace Elem Res 2020;195:591-612. [Crossref] [PubMed]
- McClung JP, Scrimgeour AG. Zinc: an essential trace element with potential benefits to soldiers. Mil Med 2005;170:1048-52. [Crossref] [PubMed]
- Krishna SS, Majumdar I, Grishin NV. Structural classification of zinc fingers: survey and summary. Nucleic Acids Res 2003;31:532-50. [Crossref] [PubMed]
- Hongfang G, Guanghui C, Khan R, et al. Review: Molecular structure and functions of zinc binding metallothionein-1 protein in mammalian body system. Pak J Pharm Sci 2020;33:1719-26. [PubMed]
- Kim MJ, Kil M, Jung JH, et al. Roles of Zinc-responsive transcription factor Csr1 in filamentous growth of the pathogenic Yeast Candida albicans. J Microbiol Biotechnol 2008;18:242-7. [PubMed]
- Wang M, Fan L, Wei W, et al. Integrated multi-omics uncovers reliable potential biomarkers and adverse effects of zinc deficiency. Clin Nutr 2021;40:2683-96. [Crossref] [PubMed]
- Hernández-Camacho JD, Vicente-García C, Parsons DS, et al. Zinc at the crossroads of exercise and proteostasis. Redox Biol 2020;35:101529. [Crossref] [PubMed]
- Gondal AH, Zafar A, Zainab D, et al. A detailed review study of zinc involvement in animal, plant and human nutrition. Indian Journal of Pure & Applied Biosciences 2021;9:262-71. [Crossref]
- Plum LM, Rink L, Haase H. The essential toxin: impact of zinc on human health. Int J Environ Res Public Health 2010;7:1342-65. [Crossref] [PubMed]
- Skrajnowska D, Bobrowska-Korczak B. Role of Zinc in Immune System and Anti-Cancer Defense Mechanisms. Nutrients 2019;11:2273. [Crossref] [PubMed]
- Gammoh NZ, Rink L. Zinc and the immune system. In: Mahmoudi M, Rezaei N. editors. Nutrition and Immunity. Cham: Springer; 2019:127-58.
- Khoshdel Z, Naghibalhossaini F, Abdollahi K, et al. Serum Copper and Zinc Levels Among Iranian Colorectal Cancer Patients. Biol Trace Elem Res 2016;170:294-9. [Crossref] [PubMed]
- Cook HV, Doncheva NT, Szklarczyk D, et al. Viruses.STRING: A Virus-Host Protein-Protein Interaction Database. Viruses 2018;10:519. [Crossref] [PubMed]
- Fan Y, Zhang X, Yang L, et al. Zinc inhibits high glucose-induced NLRP3 inflammasome activation in human peritoneal mesothelial cells. Mol Med Rep 2017;16:5195-202. [Crossref] [PubMed]
- Gao L, Fan Y, Zhang X, et al. Zinc supplementation inhibits the high glucose‑induced EMT of peritoneal mesothelial cells by activating the Nrf2 antioxidant pathway. Mol Med Rep 2019;20:655-63. [Crossref] [PubMed]
- Goyal H, Mann R, Gandhi Z, et al. Scope of Artificial Intelligence in Screening and Diagnosis of Colorectal Cancer. J Clin Med 2020;9:3313. [Crossref] [PubMed]
- Vassos N, Piso P. Metastatic Colorectal Cancer to the Peritoneum: Current Treatment Options. Curr Treat Options Oncol 2018;19:49. [Crossref] [PubMed]
- Marcuello M, Vymetalkova V, Neves RPL, et al. Circulating biomarkers for early detection and clinical management of colorectal cancer. Mol Aspects Med 2019;69:107-22. [Crossref] [PubMed]
- Mei Y, Xiao W, Hu H, et al. Single-cell analyses reveal suppressive tumor microenvironment of human colorectal cancer. Clin Transl Med 2021;11:e422. [Crossref] [PubMed]
- Penkert J, Ripperger T, Schieck M, et al. On metabolic reprogramming and tumor biology: A comprehensive survey of metabolism in breast cancer. Oncotarget 2016;7:67626-49. [Crossref] [PubMed]
- Han L, Lam EW, Sun Y. Extracellular vesicles in the tumor microenvironment: old stories, but new tales. Mol Cancer 2019;18:59. [Crossref] [PubMed]
- Knoell DL, Smith D, Bao S, et al. Imbalance in zinc homeostasis enhances lung Tissue Loss following cigarette smoke exposure. J Trace Elem Med Biol 2020;60:126483. [Crossref] [PubMed]
- Wan Y, Zhang B. The Impact of Zinc and Zinc Homeostasis on the Intestinal Mucosal Barrier and Intestinal Diseases. Biomolecules 2022;12:900. [Crossref] [PubMed]
- O'Cathail SM, Wu CH, Thomas R, et al. NRF2 Mediates Therapeutic Resistance to Chemoradiation in Colorectal Cancer through a Metabolic Switch. Antioxidants (Basel) 2021;10:1380. [Crossref] [PubMed]
- Zhao Y, Bruemmer D. NR4A orphan nuclear receptors: transcriptional regulators of gene expression in metabolism and vascular biology. Arterioscler Thromb Vasc Biol 2010;30:1535-41. [Crossref] [PubMed]
- Fayard E, Auwerx J, Schoonjans K. LRH-1: an orphan nuclear receptor involved in development, metabolism and steroidogenesis. Trends Cell Biol 2004;14:250-60. [Crossref] [PubMed]
- Tanaka N, Aoyama T, Kimura S, et al. Targeting nuclear receptors for the treatment of fatty liver disease. Pharmacol Ther 2017;179:142-57. [Crossref] [PubMed]
- Triki M, Lapierre M, Cavailles V, et al. Expression and role of nuclear receptor coregulators in colorectal cancer. World J Gastroenterol 2017;23:4480-90. [Crossref] [PubMed]
- Liu C, Lu Z, Yan J, et al. Construction of a prognostic signature associated with liver metastases for prognosis and immune response prediction in colorectal cancer. Front Oncol 2023;13:1234045. [Crossref] [PubMed]
- Wei FZ, Mei SW, Wang ZJ, et al. HAMP as a Prognostic Biomarker for Colorectal Cancer Based on Tumor Microenvironment Analysis. Front Oncol 2022;12:884474. [Crossref] [PubMed]
- Miyaki M, Kuroki T. Role of Smad4 (DPC4) inactivation in human cancer. Biochem Biophys Res Commun 2003;306:799-804. [Crossref] [PubMed]
- Tang Y, Li Y, Yu H, et al. Quercetin prevents ethanol-induced iron overload by regulating hepcidin through the BMP6/SMAD4 signaling pathway. J Nutr Biochem 2014;25:675-82. [Crossref] [PubMed]
- Grider A, Lewis RD, Laing EM, et al. Zinc affects miR-548n, SMAD4, SMAD5 expression in HepG2 hepatocyte and HEp-2 lung cell lines. Biometals 2015;28:959-66. [Crossref] [PubMed]