The role of CD4+ CAR T cells in cancer immunotherapy
Chimeric antigen receptor (CAR) T cell immunotherapy has achieved remarkable clinical success in the treatment of selected blood cancers (1). Additionally, highly impressive efficacy has recently been reported in patients with the paediatric solid tumour, neuroblastoma (2). Nonetheless, common adult cancer types such as lung cancer largely remain stubbornly resistant to CAR T cell immunotherapy (3,4). To address this, it is widely acknowledged that there is a need for more effective CAR T cell trafficking and infiltration of solid tumours, selection of more tumour specific targets, improved CAR T cell persistence, enhanced performance within the immunosuppressive tumour microenvironment (TME) and prevention of life-threatening CAR T cell-associated toxicities (3).
Ideal targets for CAR T cell immunotherapy are highly expressed on tumour cells with little or absent expression on healthy cells. In solid tumours, such prime targets are very difficult to identify as a result of which more than 100 candidates have been evaluated pre-clinically and 30 advanced to clinical studies (5,6). Risk of toxicity and enhanced delivery to the TME may be further reduced by chemokine receptor armouring, thereby directing CAR T cells more efficiently to tumours that produce cognate chemokines, whilst avoiding low antigen expressing healthy cells (7,8). Alternatively, where appropriate, CAR T cells can be injected intratumourally, although penetration within target lesions remains sub-optimal (9).
The TME also contributes importantly to poor CAR T cell function, due to physical constraints, antigen overload, deployment of multiple immunosuppressive attributes, nutrient deprivation and accumulation of harmful metabolites (10,11). As a result, T cell exhaustion may commonly ensue at that site (12). The key pathways that mediate exhaustion include the induced upregulation of inhibitory immune checkpoints such as programmed cell death protein 1 (PD-1), lymphocyte activation gene 3 (LAG-3) and T cell immunoglobulin and mucin domain 3 (Tim-3), upregulation of suppressive transcription factors such as thymocyte selection-associated high mobility group (HMG) box (TOX) and nuclear receptor family 4 (NR4) and onset of metabolic dysfunction. A detailed review of tumour resistance mechanisms and approaches to overcome this by CAR T cell engineering has recently been published (13). In brief, commonly used approaches entail the use of more durable receptor designs, switch systems and manufacturing processes and/or engineering strategies that foster enhanced CAR T cell fitness within the tumour battleground (14-18). Moreover, efficacy and safety may be further potentiated through the use of products derived from early memory/stem memory T cells, owing to their superior persistence and reduced propensity to cause cytokine release syndrome (CRS) (19,20).
Most commonly, CAR T cell batches are manufactured from unfractionated patient-derived T cells. As a result, individual products are highly heterogeneous in nature, often with marked donor-dependent variation in cellular composition and immunophenotype. These attributes can significantly shape CAR T cell fitness and ultimate anti-tumour efficacy (21,22). Donor to donor variability, selection of different CAR T cell architectures and signalling moieties, use of diverse manufacturing protocols and the incorporation of distinct armouring technologies across different studies all potentially obscure the identification of meaningful predictive markers of anti-tumour activity, persistence, and adverse events.
One fundamental question in this regard is the importance of T cell subset composition in the generation of effective CAR T cell drugs. The direct anti-tumour cytotoxic function of CD8+ T cells is widely appreciated. By contrast, CD4+ T cells are traditionally considered to operate primarily as supportive “helper cells”, acting via direct (e.g., cytokine-mediated) and indirect (e.g., maintenance of cross-presenting dendritic cells) mechanisms (23). However, this view of how CD4+ T cells contribute to anti-tumour immunity has begun to evolve on the foot of some recent experimental studies and clinical observations, including the paper featured in this commentary by Boulch et al. (24).
Recent clinical studies have highlighted some unexpected mechanisms by which CD4+ CAR T cells contribute to tumour control. The clinical importance of cytotoxic CD4+ CAR T cells was highlighted in long-term adult survivors with chronic lymphocytic leukaemia following CD19 CAR T cell immunotherapy. In these subjects, the initial phase of anti-tumour activity was mediated by CD8+ or γδ T cells, whereas long-term disease control was almost exclusively mediated by oligoclonal cytotoxic CD4+ T cells (25). Novel helper mechanisms by which CD4+ CAR T cells support their CD8+ counterparts have also been uncovered during the CAR T cell manufacturing process and include interactions mediated between CD40L-CD40 and CD70-CD27 (26). In the absence of such support, CD8+ CAR T cells exhibit a hypofunctional phenotype.
A number of recent pre-clinical investigations have also provided new insights into the importance of CD4+ CAR T cells in anti-tumour efficacy. In a pleural mesothelioma model (27), regional co-delivery of CD4+ and CD8+ CAR T cells targeted against mesothelin resulted in the enhanced accumulation of the latter at the site of disease, leading to increased overall efficacy. When the authors compared the use of different T cell subset compositions, anti-tumour efficacy was ranked in the order (I) CD4+ CAR plus CD8+ CAR; (II) CD4+ CAR alone and (III) CD8+ CAR alone (27). In an orthotopic glioblastoma model, CD8+ CAR T cells exhibit a marked susceptibility to become rapidly exhausted, in contrast to CD4+ CAR T cells (28). In that study, both products were manufactured from enriched CD62L+ CD45RO+ central memory T cells. Although CD4+ CAR T cells achieved greater efficacy, they could not rescue CD8+ CAR T cells from exhaustion meaning that the order of anti-tumour potency was (I) CD4+ CAR alone; (II) CD4+ CAR plus CD8+ CAR equal to (III) CD8+ CAR alone. A notable finding highlighted in both reports was the fact that CD4+ CAR T cells demonstrated intrinsic tumour cell killing activity, in addition to provision of CD8+ cytotoxic T cell support. In keeping with this, CD4+ CAR T cells have been shown to undertake direct, perforin-mediated cytotoxicity, sometimes even acting as “serial” tumour cell killers (29). However, rate of tumour cell killing by CD4+ CAR T cells is significantly slower than by their CD8+ counterparts, a point ascribed to the lower granzyme B content of these cells. Importantly, although CD4+ CAR T cells are less cytolytic than CD8+ CAR T cells, they do produce higher amounts of a range of cytokines, including interleukin (IL)-2 and interferon (IFN)-γ (30) and this is a point that was picked up by Boulch et al. in their study (24). Regarding CD4+ T cell subsets, it should also be noted that particularly impressive results have recently been reported for CD26high CD4+ CAR T cells in a mesothelioma xenograft model, although the role of CD26 in these findings remains unclear (31).
The importance of CD4+ CAR T cells in disease control has also been demonstrated in some haematological cancer models. Studies in leukaemic models have highlighted the importance of CD4+ T cells in achieving myeloid cell activation, elevated cytokine release, enhanced CAR T cell expansion kinetics and ultimately, greater durability of response (24,32). Moreover, in a humanised mouse model of B cell acute lymphoblastic leukaemia (B-ALL), CAR T cells recovered from the bone marrow of mice that had achieved complete responses were enriched for CD4+ CAR T cells (32). Similarly, in vivo generated CD19-specific CD4+ CAR T cells achieved better disease control than CD8+ CAR T cells or a mix of the two subsets in an Nalm-6 B-ALL model (33). In contrast, although Raji lymphoma xenografts respond better to CD8+ (central memory) rather than CD4+ (naïve) CAR T cell counterparts, the combination of both of these subsets yielded best results (30). These studies set the scene for the development of clinical products such as lisocabtagene maraleucel in which a defined subset of CD4+ and CD8+ CAR T cells are infused, an approach that achieves comparable efficacy to other approved products, with some indications that toxicity may be reduced (34).
Dissection of the in vivo cross talk that occurs between CD4+ and CD8+ CAR T cells requires the use of immune competent model systems. Two recent pre-clinical studies by Boulch et al. (24) have proven highly informative in this respect and their findings are summarised graphically in Figure 1. Using a model of CD19+ Burkitt-like lymphoma, they first demonstrated that CD8+ CAR T cells co-localised with tumour to a greater extent than their CD4+ counterparts and this was accompanied by increased tumour cell apoptosis. These findings chime well with the established direct tumour cytolytic activity of CD8+ CAR T cells (35). As before, they also found that CD4+ CAR T cells could induce tumour cell killing. Intriguingly however, this activity was primarily mediated in the absence of cell contact via a process they dubbed “indirect killing”. Additionally, CAR CD4+ T cells proved more effective in recruiting endogenous immune effectors to re-sculpt the TME in a manner that was strongly dependent on IFN-γ and IL-12. Thus, complementarity was demonstrated between both subsets which, respectively, were more adept at immune cell activation (CD4+ CAR T) and direct tumour cell killing (CD8+ CAR T). In a key follow-up study by the same group, mechanisms that underlie CD4+ CAR T cell-dependent cytotoxicity were further interrogated (24). They used intravital imaging of the bone marrow in a CD19 CAR T cell/proB-ALL tumour model and employed a range of knockout mouse models to ascertain the relative importance of CAR T cell-derived IFN-γ and perforin, and tumour or host cell expression of IFN-γ receptor (IFN-γR). By this means, they determined that over two-thirds of CD4+ CAR T cell tumour killing events occurred indirectly through IFN-γ-dependent mechanisms, whereas perforin-dependent direct killing only accounted for the residual minority of these events. Since IFN-γ could disseminate widely throughout the TME, it induced apoptotic cell death of tumour cells at a significant distance from the originating CD4+ CAR T cell, contrasting with the highly localised cytolytic activity of CD8+ CAR T cells. Moreover, both antigen positive and negative variant tumour cells were susceptible to this CD4+ CAR T cell-driven clearance mechanism. However, tumour cell sensitivity to IFN-γ-dependent apoptosis proved critical in this regard, potentially accounting for the variable importance of this mechanism in different tumour types. While causes of IFN-γ resistance were not identified, alterations in IFN-γR expression were excluded (24), highlighting the probability that downstream pathway deficiencies are responsible and warrant further study. In keeping with this, defects in the IFN-γR signalling pathway have also been linked to resistance to CAR T cell mediated destruction of solid tumours (36). However, in apparent conflict with these findings of Boulch et al. is the demonstration by the Maus group that IFN-γ is dispensable for cytotoxic responses and anti-tumour activity against haematological tumours (36,37). A possible reconciling factor to explain these apparent differences is that the haematological models under study by Larson et al. were highly susceptible to CD8+ CAR T cell tumour cell clearance, obviating the need for this CD4/IFN-γ-dependent mechanism (36). Consequently, it would be of great interest to examine the susceptibility of IFN-γ resistant tumour cells to destruction by CD8+ CAR T cells.
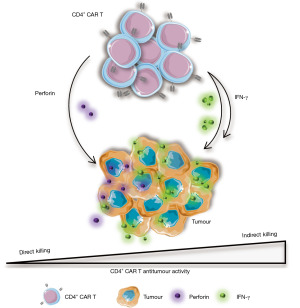
Several potentially important additional tumour protective roles of IFN-γ have emerged in recent years. First, this cytokine has been implicated in intratumoural myeloid cell activation and the facilitation of endogenous T cell memory, both in pre-clinical models and in glioblastoma patients following CAR T cell treatment (38,39). Moreover, IFN-γ is known to also induce target cell death by ferroptosis whereby lipid peroxide accumulation is triggered in an iron-dependent manner (40). The importance of these target-independent effects of IFN-γ stems from the fact that most solid tumour CAR T cell targets exhibit heterogeneous expression, necessitating additional immune effector mechanisms to consolidate clinical response.
Clinical studies have also assigned an increasingly prominent role for CD4+ T cells, both in CAR T cell and other forms of cancer immunotherapy. In the article featured in this commentary, Boulch et al. reported that in patients with diffuse large B cell lymphoma (DLBCL) who received CD19-specific CAR T cell immunotherapy, there was a strong correlation between high CD4:CD8 ratio and IFN-γ release. Importantly, in those patients with high CD4:CD8 ratio and high serum IFN-γ concentration, there was a significant improvement in progression-free survival and overall survival when compared to patients with a high CD4:CD8 ratio and low levels of IFN-γ (24). Given that these patients had a haematological malignancy, these data need to be carefully reconciled with the findings of the Maus group (36,37), as highlighted above. In the context of immune checkpoint blockade, clonally expanded cytotoxic CD4+ T cells that produce IFN-γ have been identified in bladder cancer, where tumour cell killing was major histocompatibility complex (MHC) class II dependent (41). Indeed, a gene signature associated with this cell type correlated with clinical response to anti-programmed death ligand 1 (PD-L1) immunotherapy. Similarly, CD4+ T cell populations have been correlated with positive clinical responses to immune checkpoint blockade in breast and colorectal cancer (42,43). In the colorectal study, a CD4+ T cell population with a strongly cytotoxic gene signature was once again identified. In a phase 1 dose escalation trial undertaken in our centre to evaluate intratumoural pan erythroblastic leukaemia viral oncogene homologue (ErbB)-specific CAR T cells in patients with relapsed refractory head and neck squamous cell carcinoma, we found that the administration of CD4+ T cell rich CAR product was correlated with superior outcome (9). However, although CD4+ CAR T cells drive potent anti-tumour efficacy, evidence also suggests that these cells are the main drivers of associated adverse events, such as CRS (32,44). Intriguingly. IFN-γ has also been implicated in this toxic event (37), highlighting the potentially double edged nature of the CD4+ T cell/IFN-γ axis identified by Boulch and others. Consequently, adjusting the CD4:CD8 ratio in CAR T cell products may help to achieve the appropriate balance of potent anti-tumour activity whilst reducing harmful adverse events to tolerable levels.
Acknowledgments
Funding: None.
Footnote
Provenance and Peer Review: This article was commissioned by the editorial office, Translational Cancer Research. The article has undergone external peer review.
Peer Review File: Available at https://tcr.amegroups.com/article/view/10.21037/tcr-23-2044/prf
Conflicts of Interest: All authors have completed the ICMJE uniform disclosure form (available at https://tcr.amegroups.com/article/view/10.21037/tcr-23-2044/coif). C.B. is an employee of Leucid Bio. J.M. is the scientific founder and chief scientific officer of Leucid Bio and holds founder’s shares in the company. He consults for Leucid Bio, Boult, Poolbeg Pharma, Allen & Overy; and has received honoraria for speaking at Immunotherapy and Cellular Therapy in Hemato-Oncology and King’s CAR-T Preceptorship Course. He also owns patents planned, issued or pending that do not pertain to the role of CD4+ CAR T-cells in cancer immunotherapy. He participates in scientific advisory and data monitoring boards (SAB and SMB), and is a co-PI of a grant funded by Cancer Research UK. M.G. is an associate director at Leucid Bio and holds share options in the company. She also has patents planned, issued or pending that do not pertain to the role of CD4+ CAR T-cells in cancer immunotherapy. The authors have no other conflicts of interest to declare.
Ethical Statement: The authors are accountable for all aspects of the work in ensuring that questions related to the accuracy or integrity of any part of the work are appropriately investigated and resolved.
Open Access Statement: This is an Open Access article distributed in accordance with the Creative Commons Attribution-NonCommercial-NoDerivs 4.0 International License (CC BY-NC-ND 4.0), which permits the non-commercial replication and distribution of the article with the strict proviso that no changes or edits are made and the original work is properly cited (including links to both the formal publication through the relevant DOI and the license). See: https://creativecommons.org/licenses/by-nc-nd/4.0/.
References
- Halim L, Maher J. CAR T-cell immunotherapy of B-cell malignancy: the story so far. Ther Adv Vaccines Immunother 2020;8:2515135520927164. [Crossref] [PubMed]
- Del Bufalo F, De Angelis B, Caruana I, et al. GD2-CART01 for Relapsed or Refractory High-Risk Neuroblastoma. N Engl J Med 2023;388:1284-95. [Crossref] [PubMed]
- Liu Y, He Y. A narrative review of chimeric antigen receptor-T (CAR-T) cell therapy for lung cancer. Ann Transl Med 2021;9:808. [Crossref] [PubMed]
- Maher J. Chimeric Antigen Receptor (CAR) T-Cell Therapy for Patients with Lung Cancer: Current Perspectives. Onco Targets Ther 2023;16:515-32. [Crossref] [PubMed]
- Maher J, Davies DM. CAR Based Immunotherapy of Solid Tumours-A Clinically Based Review of Target Antigens. Biology (Basel) 2023;12:287. [Crossref] [PubMed]
- Maher J, Davies DM. CAR-Based Immunotherapy of Solid Tumours-A Survey of the Emerging Targets. Cancers (Basel) 2023;15:1171. [Crossref] [PubMed]
- Hamieh M, Mansilla-Soto J, Rivière I, et al. Programming CAR T Cell Tumor Recognition: Tuned Antigen Sensing and Logic Gating. Cancer Discov 2023;13:829-43. [Crossref] [PubMed]
- Whilding LM, Halim L, Draper B, et al. CAR T-Cells Targeting the Integrin αvβ6 and Co-Expressing the Chemokine Receptor CXCR2 Demonstrate Enhanced Homing and Efficacy against Several Solid Malignancies. Cancers (Basel) 2019;11:674. [Crossref] [PubMed]
- Papa S, Adami A, Metoudi M, et al. Intratumoral pan-ErbB targeted CAR-T for head and neck squamous cell carcinoma: interim analysis of the T4 immunotherapy study. J Immunother Cancer 2023;11:e007162. [Crossref] [PubMed]
- Kankeu Fonkoua LA, Sirpilla O, Sakemura R, et al. CAR T cell therapy and the tumor microenvironment: Current challenges and opportunities. Mol Ther Oncolytics 2022;25:69-77. [Crossref] [PubMed]
- Hou AJ, Chen LC, Chen YY. Navigating CAR-T cells through the solid-tumour microenvironment. Nat Rev Drug Discov 2021;20:531-50. [Crossref] [PubMed]
- Gemta LF, Siska PJ, Nelson ME, et al. Impaired enolase 1 glycolytic activity restrains effector functions of tumor-infiltrating CD8(+) T cells. Sci Immunol 2019;4:eaap9520. [Crossref] [PubMed]
- Glover M, Avraamides S, Maher J. How Can We Engineer CAR T Cells to Overcome Resistance? Biologics 2021;15:175-98. [PubMed]
- Valkenburg KC, de Groot AE, Pienta KJ. Targeting the tumour stroma to improve cancer therapy. Nat Rev Clin Oncol 2018;15:366-81. [Crossref] [PubMed]
- Rodriguez-Garcia A, Palazon A, Noguera-Ortega E, et al. CAR-T Cells Hit the Tumor Microenvironment: Strategies to Overcome Tumor Escape. Front Immunol 2020;11:1109. [Crossref] [PubMed]
- Huang Y, Kim BYS, Chan CK, et al. Improving immune-vascular crosstalk for cancer immunotherapy. Nat Rev Immunol 2018;18:195-203. [Crossref] [PubMed]
- Wu Y, Huang Z, Harrison R, et al. Engineering CAR T cells for enhanced efficacy and safety. APL Bioeng 2022;6:011502. [Crossref] [PubMed]
- Lu L, Xie M, Yang B, et al. Enhancing the safety of CAR-T cell therapy: Synthetic genetic switch for spatiotemporal control. Sci Adv 2024;10:eadj6251. [Crossref] [PubMed]
- Arcangeli S, Bove C, Mezzanotte C, et al. CAR T cell manufacturing from I/stem memory T lymphocytes enhances antitumor responses while curtailing cytokine release syndrome. J Clin Invest 2022;132:e150807. [Crossref] [PubMed]
- Fraietta JA, Lacey SF, Orlando EJ, et al. Determinants of response and resistance to CD19 chimeric antigen receptor (CAR) T cell therapy of chronic lymphocytic leukemia. Nat Med 2018;24:563-71. [Crossref] [PubMed]
- Sabatino M, Hu J, Sommariva M, et al. Generation of clinical-grade CD19-specific CAR-modified CD8+ memory stem cells for the treatment of human B-cell malignancies. Blood 2016;128:519-28. [Crossref] [PubMed]
- Turtle CJ, Hanafi LA, Berger C, et al. CD19 CAR-T cells of defined CD4+:CD8+ composition in adult B cell ALL patients. J Clin Invest 2016;126:2123-38. [Crossref] [PubMed]
- Tay RE, Richardson EK, Toh HC. Revisiting the role of CD4(+) T cells in cancer immunotherapy-new insights into old paradigms. Cancer Gene Ther 2021;28:5-17. [Crossref] [PubMed]
- Boulch M, Cazaux M, Cuffel A, et al. Tumor-intrinsic sensitivity to the pro-apoptotic effects of IFN-γ is a major determinant of CD4+ CAR T-cell antitumor activity. Nat Cancer 2023;4:968-83. [Crossref] [PubMed]
- Melenhorst JJ, Chen GM, Wang M, et al. Decade-long leukaemia remissions with persistence of CD4(+) CAR T cells. Nature 2022;602:503-9. [Crossref] [PubMed]
- Lee SY, Lee DH, Sun W, et al. CD8(+) chimeric antigen receptor T cells manufactured in absence of CD4(+) cells exhibit hypofunctional phenotype. J Immunother Cancer 2023;11:e007803. [Crossref] [PubMed]
- Adusumilli PS, Cherkassky L, Villena-Vargas J, et al. Regional delivery of mesothelin-targeted CAR T cell therapy generates potent and long-lasting CD4-dependent tumor immunity. Sci Transl Med 2014;6:261ra151. [Crossref] [PubMed]
- Wang D, Aguilar B, Starr R, et al. Glioblastoma-targeted CD4+ CAR T cells mediate superior antitumor activity. JCI Insight 2018;3:e99048. [Crossref] [PubMed]
- Liadi I, Singh H, Romain G, et al. Individual Motile CD4(+) T Cells Can Participate in Efficient Multikilling through Conjugation to Multiple Tumor Cells. Cancer Immunol Res 2015;3:473-82. [Crossref] [PubMed]
- Sommermeyer D, Hudecek M, Kosasih PL, et al. Chimeric antigen receptor-modified T cells derived from defined CD8+ and CD4+ subsets confer superior antitumor reactivity in vivo. Leukemia 2016;30:492-500. [Crossref] [PubMed]
- Nelson MH, Knochelmann HM, Bailey SR, et al. Identification of human CD4(+) T cell populations with distinct antitumor activity. Sci Adv 2020;6:eaba7443. [Crossref] [PubMed]
- Bove C, Arcangeli S, Falcone L, et al. CD4 CAR-T cells targeting CD19 play a key role in exacerbating cytokine release syndrome, while maintaining long-term responses. J Immunother Cancer 2023;11:e005878. [Crossref] [PubMed]
- Agarwal S, Hanauer JDS, Frank AM, et al. In Vivo Generation of CAR T Cells Selectively in Human CD4(+) Lymphocytes. Mol Ther 2020;28:1783-94. [Crossref] [PubMed]
- Abramson JS, Palomba ML, Gordon LI, et al. Lisocabtagene maraleucel for patients with relapsed or refractory large B-cell lymphomas (TRANSCEND NHL 001): a multicentre seamless design study. Lancet 2020;396:839-52. [Crossref] [PubMed]
- Boulch M, Cazaux M, Loe-Mie Y, et al. A cross-talk between CAR T cell subsets and the tumor microenvironment is essential for sustained cytotoxic activity. Sci Immunol 2021;6:eabd4344. [Crossref] [PubMed]
- Larson RC, Kann MC, Bailey SR, et al. CAR T cell killing requires the IFNγR pathway in solid but not liquid tumours. Nature 2022;604:563-70. [Crossref] [PubMed]
- Bailey SR, Vatsa S, Larson RC, et al. Blockade or Deletion of IFNγ Reduces Macrophage Activation without Compromising CAR T-cell Function in Hematologic Malignancies. Blood Cancer Discov 2022;3:136-53. [Crossref] [PubMed]
- Alizadeh D, Wong RA, Gholamin S, et al. IFNγ Is Critical for CAR T Cell-Mediated Myeloid Activation and Induction of Endogenous Immunity. Cancer Discov 2021;11:2248-65. [Crossref] [PubMed]
- Kruse B, Buzzai AC, Shridhar N, et al. CD4(+) T cell-induced inflammatory cell death controls immune-evasive tumours. Nature 2023;618:1033-40. [Crossref] [PubMed]
- Wang W, Green M, Choi JE, et al. CD8(+) T cells regulate tumour ferroptosis during cancer immunotherapy. Nature 2019;569:270-4. [Crossref] [PubMed]
- Oh DY, Kwek SS, Raju SS, et al. Intratumoral CD4(+) T Cells Mediate Anti-tumor Cytotoxicity in Human Bladder Cancer. Cell 2020;181:1612-1625.e13. [Crossref] [PubMed]
- Schmidt M, Weyer-Elberich V, Hengstler JG, et al. Prognostic impact of CD4-positive T cell subsets in early breast cancer: a study based on the FinHer trial patient population. Breast Cancer Res 2018;20:15. [Crossref] [PubMed]
- Zhang L, Yu X, Zheng L, et al. Lineage tracking reveals dynamic relationships of T cells in colorectal cancer. Nature 2018;564:268-72. [Crossref] [PubMed]
- Boulch M, Cazaux M, Cuffel A, et al. A major role for CD4(+) T cells in driving cytokine release syndrome during CAR T cell therapy. Cell Rep Med 2023;4:101161. [Crossref] [PubMed]