Up-regulation of NCAPG mediated by E2F1 facilitates the progression of osteosarcoma through the Wnt/β-catenin signaling pathway
Highlight box
Key findings
• Non-SMC condensin I complex subunit G (NCAPG) expression is abnormally upregulated in osteosarcoma;
• E2F transcription factor 1 (E2F1) binding in the NCAPG promoter region mediates its elevated expression in osteosarcoma;
• NCAPG promotes osteosarcoma progression via the Wnt/β-catenin signaling axis.
What is known and what is new?
• Previous studies indicated that NCAPG is associated with the prognosis and progression of a series of solid malignant tumors.
• Our study reveals that NCAPG expression is upregulated in osteosarcoma. We also elucidated that E2F1 binds to the promoter region of NCAPG to promote its transcription, and elevated NCAPG promotes osteosarcoma progression through the Wnt/β-catenin pathway.
What is the implication, and what should change now?
• The potential application value is to provide some theoretical support for the further exploration of diagnostic biomarkers for osteosarcoma in the future, as well as for precision-targeted therapies.
Introduction
Osteosarcoma is a malignant tumor that occurs in adolescents and the elderly. Owing to the driving factors of osteosarcoma being complex and diverse, and the pathogenesis being unclear, it brings great challenges to the clinical treatment of patients. At present, the treatment methods for osteosarcoma mainly include surgery combined with chemotherapy and neoadjuvant chemotherapy. In addition, immunotherapy, gene therapy, and stem cell therapy are occasionally used clinically. However, some patients still have the risk of metastasis and recurrence, and the prognosis of patients is poor. Therefore, it is urgent to search for new biomarkers and therapeutic targets for osteosarcoma to improve the efficiency of diagnosis and treatment.
As a regulatory subunit, the protein encoded by the non-SMC condensin I complex subunit G (NCAPG) gene participates in the formation of the condensin complex, which plays a role in condensing and stabilizing chromosomes during mitosis and meiosis. In addition to its significant role in normal cellular physiological activities, increasing evidence has shown that NCAPG is also closely related to tumors. It has been found that NCAPG expression levels are associated with poor prognosis in a series of solid tumors including hepatocellular carcinoma, gastric cancer, ovarian cancer, etc. (1-7). Mechanistically, Wu et al. demonstrated that NCAPG could promote the progression of lung adenocarcinoma via the TGF-β signaling pathway (8). In cardia adenocarcinoma and colorectal cancer, studies indicated that overexpression of NCAPG facilitates epithelial-mesenchymal transition (EMT) through the Wnt/β-catenin signaling pathway (9,10). Interestingly, researchers revealed that NCAPG participates in trastuzumab resistance in human epidermal growth factor receptor 2 (HER2)-positive breast cancer via activating SRC/STAT3 signaling axis (11). In osteosarcoma, many studies have found that the Wnt/β-catenin signaling pathway is closely related to osteosarcoma progression and chemotherapy resistance, among others. Targeting this pathway can significantly inhibit tumor malignant progression while enhancing patient sensitivity to chemotherapy. However, whether NCAPG is involved in osteosarcoma progression by regulating the Wnt/β-catenin signaling axis needs to be further elucidated (12-14).
As a protein-coding gene, the expression of NCAPG is also regulated by a variety of molecules. MicroRNA (miRNA) is a class of non-coding single-stranded RNA molecules of about 22 nucleotides in length encoded by endogenous genes and involved in post-transcriptional gene expression regulation. Studies have clarified that a variety of miRNAs, including miR-99a-3p, miR-378-3p, miR-23b-3p, miR-214-3p, etc., are involved in regulating the expression of NCAPG in a series of malignant neoplasms (15-18). E2F transcription factor 1 (E2F1), a transcription factor closely related to cell cycle and tumor regulation, has been reported to be involved in the progression of cervical cancer, breast cancer, gastric cancer, and other tumors via regulating the expression of downstream target genes (19-21).
However, the regulatory relationship between NCAPG and its regulating transcription factors, especially E2F1, has not been reported yet.
Here, we utilized public databases and online website tools to explore the effect of NCAPG on the malignant biological behavior of osteosarcoma cells and its underlying molecular mechanism combined with in vitro experiments. Further, we have initially revealed the regulating mechanism that leads to the abnormal expression of NCAPG in osteosarcoma, hoping to provide a certain theoretical basis for the clinical diagnosis and precisely targeted therapy for osteosarcoma patients. We present this article in accordance with the MDAR reporting checklist (available at https://tcr.amegroups.com/article/view/10.21037/tcr-23-2175/rc).
Methods
Cell culture and lentivirus transfection
Human osteosarcoma cell lines involved in this paper, including the hFOB 1.19, U2OS, and MG-63, were maintained in DMEM (A culture medium containing various amino acids and glucose) supplemented with 10% fetal bovine serum-containing 100 U/mL penicillin and streptomycin. HEK293 cells were used as tool cells and maintained in the same medium as osteosarcoma cell lines. The human osteoblast hFOB1.19 cells, as control normal cells, were maintained in DMEM/F-12 supplemented with 10% fetal bovine serum. All cell lines were cultured in a 37 ℃ incubator with a concentration of 5% CO2. Lentivirus small hairpin RNA (shRNA) targeting NCAPG was obtained from Shanghai Gene Pharma Company. The detailed steps of transfection were performed according to the operating manuscript. The target sequence of NCAPG shRNA was as follows: sense: 5'-GCUGAAACAUUGCAGAAAUTT-3' and antisense: 5'-AUUUCUGCAAUGUUUCAGCTT-3'. Cell lines were obtained from National Collection of Authenticated Cell Cultures (hFOB 1.19, Organism: human, Gender: female, Morphology: epithelial cell and adherent, Serial: GNHu14, Identifier: CSTR:19375.09.3101HUMGNHu14; U2OS, Organism: human, Gender: female, Morphology: epithelial cell and adherent, Serial: TCHu 88, Identifier: CSTR:19375.09.3101HUMTCHu88; MG-63, Organism: human, Gender: male, Morphology: Fibroblast-like and adherent, Serial: TCHu124, Identifier: CSTR:19375.09.3101HUMTCHu124).
RNA isolation, reverse transcription, and reverse transcription quantitative polymerase chain reaction (RT-qPCR)
Firstly, 1 mL TRIZOL was added to lyse the cells, then transferred the cells to a 1.5 mL EP tube. Chloroform of 200 µL was added to the tube, and it was then let stand at 4 ℃ for 15 minutes. The tube was centrifuged at 12,000 rpm for 15 minutes. The supernatant was transferred to another new 1.5 mL EP tube following adding an equal volume of isopropanol. The tube was placed at 4 ℃ for 10 minutes. After centrifuging at 14,000 rpm for 10 minutes, the EP tube was taken out, and the supernatant was discarded. Next, 1 mL of 75% absolute ethanol was added to the tube, and the tube was centrifuged at 7,500 rpm for 5 min. Finally, the supernatant was discarded, and 20 µL of enzyme-free water was added to the tube to dissolve the RNA. After measuring the RNA concentration, reverse transcription was performed with RevertAid First Strand cDNA Synthesis Kit (K1622, Thermo-fisher, USA) according to the instruction manual. Then the cDNA obtained through reverse transcription was utilized for the RT-qPCR experiment. GAPDH was used as an internal control. Finally, calculate the relative quantitative value of each group through the 2−ΔΔCt method. The primers related to our research were listed as follows: GAPDH: forward 5'-CTGGGCTACACTGAGCACC-3', reverse 5'-AAGTGGTCGTTGAGGGCAATG-3'; NCAPG forward 5'-ATCCAGAAGTTAGACGGGCAG-3', reverse 5'-GTGCGCCCTACAATTTTTGGC-3'; E2F1: forward 5'-ACGTGACGTGTCAGGACCT-3', reverse 5'-GATCGGGCCTTGTTTGCTCTT-3'.
Western blot
Osteosarcoma cells and normal osteoblast were lysed using cell lysis RIPA buffer and protease inhibitor at a ratio of 100:1. Subsequently, western blot was performed as described previously (22). Antibodies related to our research included anti-NCAPG (ab226805, Abcam, Cambridge, UK), anti-GAPDH (ab8245, Abcam, Cambridge, UK), anti-E2F1 (ab245308, Abcam), anti-Snail (ab216347, Abcam), anti-E-cadherin (#3195T, CST, Boston, USA), anti-Vimentin (#5741T, CST), anti-Wnt (#2721T, CST), anti-GSK3β (ab32391, Abcam), anti-p-GSK3β (ab68476, Abcam), anti-β-catenin (ab246504, Abcam), anti-p-β-catenin (ab75777, Abcam).
MTT
Cells were seeded in a 96-well plate at a density of 5×103 per well, with 6 replicates in each group. The cells were cultured in the incubator for 1 day, 2 days, and 3 days respectively. Then, cells were fixed with 4% paraformaldehyde and stained with crystal violet dye. Finally, the optical density (OD) value of the cells in each well was measured in a microplate reader.
Colony formation assay
1×103 cells were planted in a 6-well plate. After placing the cells in an incubator for 2 weeks, take out the six-well plate, and fix the cells with 4% paraformaldehyde for 30 minutes at 4 ℃. Subsequently, cells were stained with crystal violet at room temperature for 30 minutes. Capture images and analyze the statistical results with Image J software.
Transwell and wound healing assay
Transwell assay and wound healing assay were performed as described previously (8). Differently, in the transwell experiment, the number of osteosarcoma cells planted in the upper chamber was about 8×103. In the wound healing assay, we seeded cells in a 6-well plate at a density of 2×104 per well.
Chromatin immunoprecipitation (CHIP)
Firstly, the crosslinking reaction was annulled by glycine in osteosarcoma cells treated with formaldehyde. Subsequently, sonication was utilized to process protein-DNA complexes into about 200–1,000 bp small fragments. Then, 5 µg of specific IP grade anti-E2F1 antibody was used so that the E2F1 antibody-NCAPG protein-DNA complex could be formed smoothly. Protein A/Protein G Sepharose beads were added to form an immunoprecipitated complex. After washing and reversing the cross-links, the enriched DNA was purified and then subjected to an RT-qPCR experiment.
Luciferase reporter assay
The human NCAPG luciferase reporter plasmid was constructed by inserting the NCAPG promoter sequence containing E2F1 binding sites or corresponding mutant sites into pGL3-Basic. The overexpression plasmid of E2F1 and the NCAPG luciferase reporter plasmid were co-transfected into HEK293 cells. Next, the biological activity of luciferase was detected by adding the luciferase substrate to the cells, and the transcriptional regulation of NCAPG by E2F1 was finally determined.
Online database
Osteosarcoma patients’ clinical information was obtained from the The Cancer Genome Atlas (TCGA) public database (https://www.cancer.gov/about-nci/organization/ccg/research/structural-genomics/tcga). Three online databases, JASPER (https://jaspar.genereg.net/), PROMO (http://alggen.lsi.upc.es/cgi-bin/promo_v3/promo/promoinit.cgi?dirDB=TF_8.3/), and hTFtarget (http://bioinfo.life.hust.edu.cn/hTFtarget#!/), were used to predict transcription factors in the NCAPG promoter region (23-26). GEPIA (http://gepia.cancer-pku.cn/index.html) was used to analyze the relationship between NCAPG and survival in sarcoma patients. The study was conducted in accordance with the Declaration of Helsinki (as revised in 2013).
Statistical analysis
We downloaded the transcriptome-count raw data for sarcomas from the TCGA public database, which includes 2 normal and 263 sarcoma samples. We performed log2 (count+1) processing on the count data and performed normality tests (Anderson-Darling test, Shapiro-Wilk test, and Kolmogorov-Smirnov test) on the log2(count+1) data in GraphPad Prism software. Student t-test was finally used to compare the differential expression of NCAPG, MAZ, and E2F1 between normal and sarcoma patients. For cellular experiments differences in mean values between the two groups were compared using a t-test. The correlation between groups was assessed with the Pearson correlation coefficient. Kaplan-Meier method was utilized for survival analysis and the log-rank test was used to determine statistical significance. P value of less than 0.05 was considered statistically significant. *P<0.05, **P<0.01, ***P<0.001, ****P<0.0001.
Results
NCAPG was up-regulated in sarcoma and was associated with a poor prognosis
Many studies have suggested that NCAPG is abnormally up-regulated in various malignant tumors (4,5,7-10). At the same time, among tumor patients, patients with higher NCAPG expression often indicate a poor prognosis. Considering that there are few studies on NCAPG in osteosarcoma, we analyzed the expression level of NCAPG in the tissues of patients with sarcoma in the TCGA public database by employing bioinformatics method. The results showed that the expression of NCAPG was significantly increased in sarcoma compared with normal tissues (Figure 1A). Further mining of the clinical information of sarcoma patients in the TCGA public database, we found that the expression of NCAPG was positively correlated with poor differentiation of sarcoma tissue (Figure 1B), but was not significantly correlated with tumor recurrence (Figure 1C). NCAPG is expressed at higher levels in patients with metastatic sarcoma compared to patients with non-metastatic sarcoma (Figure 1D). Combining the survival time and survival status of cases with sarcoma, we found that compared with patients with low NCAPG expression, patients with high NCAPG expression had worse disease-free survival and overall survival, which indicated that NCAPG expression was associated with a poor prognosis of patients (Figure 1E,1F).
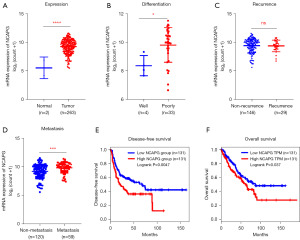
E2F1 may act as a potential transcription factor for NCAPG via bioinformatics analysis
In the early stage, we found that the expression of NCAPG was increased in sarcoma through bioinformatics analysis. It is well known that transcriptional regulation is an important mechanism leading to increased gene expression. To further explore the molecular mechanism that causes the abnormal increase of NCAPG in sarcoma, we first predicted the transcription factors in the promoter region of the NCAPG gene through the public databases JASPAR, PROMO, and hTFtarget, and comprehensively analyzed the transcription factors predicted from the three databases and found that the three transcription factors MYC associated zinc finger protein (MAZ), GATA binding protein 2 (GATA2), and E2F transcription factor 1 (E2F1) were common to the three database predictions (Figure 2A). Subsequently, we performed a correlation analysis between these three transcription factors and NCAPG, respectively, and found that in sarcoma, MAZ was positively correlated with NCAPG expression (r=0.3678) (Figure 2B), GATA2 was negatively correlated with NCAPG expression (r=−0.2880) (Figure 2C). E2F1 was also positively correlated with NCAPG (r=0.7415) (Figure 2D). Next, we analyzed the expression of MAZ and E2F1 in sarcoma and their relationship with tumor metastasis in the TCGA database. The results indicated that MAZ was up-regulated in sarcoma (Figure 2E), while it did not yet show a significant difference between metastatic and non-metastatic sarcoma (Figure 2F). E2F1 was elevated in sarcoma (Figure 2G). Moreover, E2F1 expression was higher in metastatic tumors compared to non-metastatic sarcoma (Figure 2H). The analysis results above indicated that E2F1 may be a potential transcription factor leading to the increased expression of NCAPG in sarcoma.
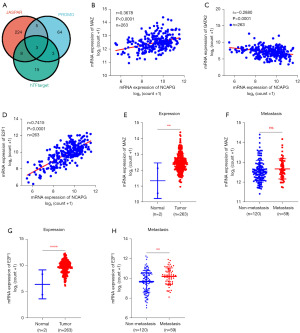
E2F1 regulated NCAPG transcription by binding to the promoter region of the NCAPG gene in osteosarcoma cells
To confirm the transcriptional regulation effect of E2F1 on NCAPG in osteosarcoma cells, we first detected the expression level of E2F1 by RT-qPCR and western blot. The results showed that compared with osteoblast, E2F1 expression was increased in osteosarcoma cells (Figure 3A,3B). We constructed stable NCAPG-silenced cell lines in U2OS and MG-63 cells and examined the influence of E2F1 knockdown on NCAPG expression. RT-qPCR and western blot results indicated that the expression of NCAPG was decreased after the knockdown of E2F1 (Figure 3C-3E). Next, we predicted the recognition motif of E2F1 by JASPAR (Figure 3F) and found that there were two E2F1 binding sites in the NCAPG promoter region (Figure 3G). Subsequently, we found via CHIP experiments that E2F1 mainly bound at site1 but not at site2 in the NCAPG promoter region (Figure 3H,3I). Finally, we performed luciferase reporter experiments by co-transfecting the E2F1 overexpression plasmid and NCAPG wild-type/mutant-type luciferase reporter plasmid in HEK-293 cells, and the results suggested that the luciferase activity of the wild-type group was higher than that of the mutant group, which further confirmed that E2F1 activated NCAPG transcription by binding at E2F1 binding site 1 in the NCAPG promoter region (Figure 3J).
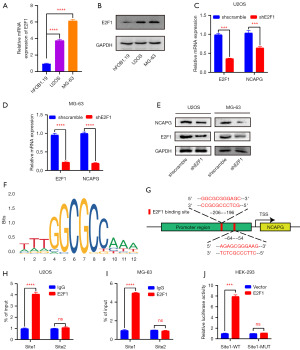
Silencing of NCAPG inhibited the proliferation ability of osteosarcoma cells
To explore the effect of NCAPG on the malignant biological behavior of osteosarcoma cells, we first verified the elevated expression of NCAPG in osteosarcoma cells by RT-qPCR and western blot experiments (Figure 4A-4C). Subsequently, we used shRNA targeting NCAPG to inhibit the expression of NCAPG in U2OS and MG-63 cells (Figure 4D-4F). The results of MTT experiments showed that compared with the control group, the growth of NCAPG knockdown cells was slowed down (Figure 4G,4H). Clonal formation experiments indicated that knockdown of NCAPG reduced the ability of U2OS and MG-63 cells to form clonal colonies (Figure 4I,4J). These results suggested that the proliferation of osteosarcoma cells was attenuated after NCAPG inhibition.
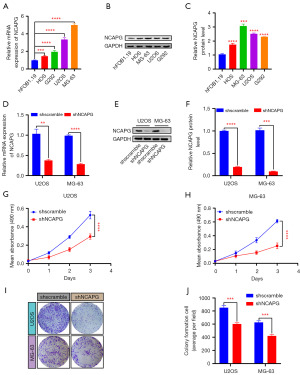
Knockdown of NCAPG suppressed the invasion and migration of osteosarcoma via restraining the Wnt/β-catenin pathway
By performing wound healing experiments on U2OS and MG-63, we found that after 24 hours of scratching, the wound repairability of osteosarcoma cells in the knockdown NCAPG group was significantly inferior to that in the control group (Figure 5A-5D). The results of transwell assays also suggested that inhibiting the expression level of NCAPG could weaken the invasive ability of U2OS and MG-63 (Figure 5E,5F). EMT is a known pathway significantly associated with tumor cell invasion and migration. Therefore, we examined the effect of NCAPG on EMT-related molecular markers, and the results showed that after knocking down NCAPG, the expression of E-cadherin protein was increased, and the levels of Snail, N-cadherin, and Vimentin were decreased (Figure 5G). This suggested that inhibition of NCAPG can repress the EMT process. Wnt/β-catenin is a switch for the EMT process, so we would like to know whether NCAPG has a certain regulatory effect on the Wnt/β-catenin pathway. Western Blot results indicated that Wnt/β-catenin was inhibited by silencing NCAPG (Figure 5H), which preliminarily proved that NCAPG may regulate the invasion and migration of osteosarcoma through the Wnt/β-catenin pathway, and ultimately accelerate the progression of osteosarcoma.
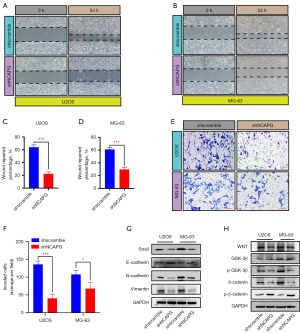
Discussion
Osteosarcoma is the most common primary bone malignancy with a low five-year survival rate. According to statistics, about 20% of patients with osteosarcoma have lung metastases at the time of diagnosis, thus losing an excellent opportunity for treatment. Therefore, early diagnosis is of great significance for the treatment and prognosis of patients with osteosarcoma. Current studies have shown that molecules including Snail, MICA, RASSF1A, HER2, MMP-2, MMP-9, and SATB2 have a certain significance in the diagnosis of osteosarcoma (27-33). However, specific biomarkers for the diagnosis of osteosarcoma are still lacking. In our study, we preliminarily found that NCAPG expression was abnormally elevated in osteosarcoma through bioinformatics analysis and cell experiment validation. At the same time, the results of the public database indicated that there was a certain correlation between the high expression level of NCAPG and the differentiation and tumor recurrence of osteosarcoma. Nonetheless, it should be noted that our study has examined only the expression level in the database and in vitro. Our results were not validated by sufficient tissue samples due to the limited access to tissue samples, which is where we need to work to supplement in the future.
Existing studies have shown that a variety of genes and related signaling pathways including receptor tyrosine-related pathways, PI3K/AKT signaling pathway, MAPK signaling pathway, TP53 mutation, PTEN mutation, etc. are involved in the occurrence and progression of osteosarcoma. Therapeutic modalities targeting the above-mentioned signaling axes and mutated genes have achieved considerable efficacy. However, due to tumor heterogeneity, different patients may respond significantly to the same treatment modality. This prompts us to search for new targeting molecules for osteosarcoma, which can provide certain theoretical guidance for the subsequent development of new clinical drugs.
Previous studies indicated that NCAPG is associated with the prognosis and progression of a series of solid malignant tumors including gastric cancer, breast cancer, oral squamous cell carcinoma, prostate cancer, etc. Loss of NCAPG inhibits tumor cell proliferation, migration, and invasion (1,11,18,21). Mechanistically, the knockdown of NCAPG induces the G0/G1 cell cycle arrest and accordingly inhibits cell division in gastric cancer (1). In non-small cell lung cancer, the function of NCAPG in promoting tumor initiation and progression is closely related to LGALS1 (4). Moreover, in HER2-positive breast cancer, NCAPG could facilitate trastuzumab resistance via mediating the SRC/STAT3 signaling pathway. Inhibition of NCAPG expression in breast cancer cells enables drug-resistant patients to regain trastuzumab-sensitive characteristics (11). In our research, we initially found that NCAPG could regulate tumor progression by regulating the Wnt/β-catenin pathway. Inhibition of NCAPG expression can suppress the proliferation and invasion of osteosarcoma cells to a certain extent. These results suggested that the development of small-molecule drugs targeting MCAPG would have great clinical application prospects. As mentioned earlier, many non-coding miRNAs can bind to NCAPG mRNA molecules, reduce the expression level of NCAPG in tumors, and then achieve the effect of inhibiting tumor progression. In this study, we confirmed for the first time through bioinformatics analysis and cell experiments that E2F1 can regulate the transcription of the NCAPG gene in osteosarcoma, and then play a role in promoting tumor malignant progression. This suggests that E2F1 has certain feasibility as a target for the treatment of osteosarcoma. Nonetheless, our research on the detailed molecular mechanisms by which NCAPG affects osteosarcoma progression is not deep enough, which is also the direction we need to continue to explore in the future.
Conclusions
In summary, our study found that NCAPG was abnormally highly expressed in sarcoma, and its high expression level was significantly associated with the poor prognosis of patients. Further exploration indicated that the transcription factor E2F1 can bind to the promoter region of the NCAPG gene to promote the transcription of NCAPG, which is one of the mechanisms leading to the up-regulation of NCAPG in osteosarcoma. Next, we expounded through phenotypic experiments that silencing of NCAPG restricted the proliferation and invasion of osteosarcoma cells. Preliminary exploration results suggested that the promoting effect of NCAPG on osteosarcoma may be related to the activation of the Wnt/β-catenin signaling pathway (Figure 6).
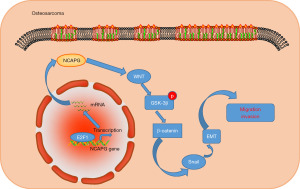
Acknowledgments
Funding: This work was supported by
Footnote
Reporting Checklist: The authors have completed the MDAR reporting checklist. Available at https://tcr.amegroups.com/article/view/10.21037/tcr-23-2175/rc
Data Sharing Statement: Available at https://tcr.amegroups.com/article/view/10.21037/tcr-23-2175/dss
Peer Review File: Available at https://tcr.amegroups.com/article/view/10.21037/tcr-23-2175/prf
Conflicts of Interest: All authors have completed the ICMJE uniform disclosure form (available at https://tcr.amegroups.com/article/view/10.21037/tcr-23-2175/coif). The authors have no conflicts of interest to declare.
Ethical Statement: The authors are accountable for all aspects of the work in ensuring that questions related to the accuracy or integrity of any part of the work are appropriately investigated and resolved. The study was conducted in accordance with the Declaration of Helsinki (as revised in 2013).
Open Access Statement: This is an Open Access article distributed in accordance with the Creative Commons Attribution-NonCommercial-NoDerivs 4.0 International License (CC BY-NC-ND 4.0), which permits the non-commercial replication and distribution of the article with the strict proviso that no changes or edits are made and the original work is properly cited (including links to both the formal publication through the relevant DOI and the license). See: https://creativecommons.org/licenses/by-nc-nd/4.0/.
References
- Sun DP, Lin CC, Hung ST, et al. Aberrant Expression of NCAPG is Associated with Prognosis and Progression of Gastric Cancer. Cancer Manag Res 2020;12:7837-46. [Crossref] [PubMed]
- Gong C, Ai J, Fan Y, et al. NCAPG Promotes The Proliferation Of Hepatocellular Carcinoma Through PI3K/AKT Signaling. Onco Targets Ther 2019;12:8537-52. [Crossref] [PubMed]
- Xu T, Dong M, Wang Z, et al. Elevated mRNA Expression Levels of NCAPG are Associated with Poor Prognosis in Ovarian Cancer. Cancer Manag Res 2020;12:5773-86. [Crossref] [PubMed]
- Sun H, Zhang H, Yan Y, et al. NCAPG promotes the oncogenesis and progression of non-small cell lung cancer cells through upregulating LGALS1 expression. Mol Cancer 2022;21:55. [Crossref] [PubMed]
- Zhang Q, Su R, Shan C, et al. Non-SMC Condensin I Complex, Subunit G (NCAPG) is a Novel Mitotic Gene Required for Hepatocellular Cancer Cell Proliferation and Migration. Oncol Res 2018;26:269-76. [Crossref] [PubMed]
- Song B, Du J, Song DF, et al. Dysregulation of NCAPG, KNL1, miR-148a-3p, miR-193b-3p, and miR-1179 may contribute to the progression of gastric cancer. Biol Res 2018;51:44. [Crossref] [PubMed]
- Guo ZY, Zhu ZT. NCAPG is a prognostic biomarker associated with vascular invasion in hepatocellular carcinoma. Eur Rev Med Pharmacol Sci 2021;25:7238-51. [PubMed]
- Wu Y, Lin Y, Pan J, et al. NCAPG promotes the progression of lung adenocarcinoma via the TGF-β signaling pathway. Cancer Cell Int 2021;21:443. [Crossref] [PubMed]
- Zhang X, Zhu M, Wang H, et al. Overexpression of NCAPG inhibits cardia adenocarcinoma apoptosis and promotes epithelial-mesenchymal transition through the Wnt/β-catenin signaling pathway. Gene 2021;766:145163. [Crossref] [PubMed]
- Shi Y, Ge C, Fang D, et al. NCAPG facilitates colorectal cancer cell proliferation, migration, invasion and epithelial-mesenchymal transition by activating the Wnt/β-catenin signaling pathway. Cancer Cell Int 2022;22:119. [Crossref] [PubMed]
- Jiang L, Ren L, Chen H, et al. NCAPG confers trastuzumab resistance via activating SRC/STAT3 signaling pathway in HER2-positive breast cancer. Cell Death Dis 2020;11:547. [Crossref] [PubMed]
- Hosseini F, Alemi F, Malakoti F, et al. Targeting Wnt/β-catenin signaling by microRNAs as a therapeutic approach in chemoresistant osteosarcoma. Biochem Pharmacol 2021;193:114758. [Crossref] [PubMed]
- Li C, Shi X, Zhou G, et al. The canonical Wnt-beta-catenin pathway in development and chemotherapy of osteosarcoma. Front Biosci (Landmark Ed) 2013;18:1384-91. [Crossref] [PubMed]
- Reimann E, Kõks S, Ho XD, et al. Whole exome sequencing of a single osteosarcoma case--integrative analysis with whole transcriptome RNA-seq data. Hum Genomics 2014;8:20. [PubMed]
- Arai T, Okato A, Yamada Y, et al. Regulation of NCAPG by miR-99a-3p (passenger strand) inhibits cancer cell aggressiveness and is involved in CRPC. Cancer Med 2018;7:1988-2002. [Crossref] [PubMed]
- Yuan Y, Jiang X, Tang L, et al. FOXM1/lncRNA TYMSOS/miR-214-3p-Mediated High Expression of NCAPG Correlates With Poor Prognosis and Cell Proliferation in Non-Small Cell Lung Carcinoma. Front Mol Biosci 2022;8:785767. [Crossref] [PubMed]
- Li P, Wen J, Ren X, et al. MicroRNA-23b-3p targets non-SMC condensing I complex subunit G to promote proliferation and inhibit apoptosis of colorectal cancer cells via regulation of the PI3K/AKT signaling pathway. Oncol Lett 2021;22:812. [Crossref] [PubMed]
- Li J, Sun S, Li J, et al. NCAPG, mediated by miR-378a-3p, regulates cell proliferation, cell cycle progression, and apoptosis of oral squamous cell carcinoma through the GSK-3β/β-catenin signaling. Neoplasma 2021;68:1201-11. [Crossref] [PubMed]
- Lv BB, Ma RR, Chen X, et al. E2F1-activated SPIN1 promotes tumor growth via a MDM2-p21-E2F1 feedback loop in gastric cancer. Mol Oncol 2020;14:2629-45. [Crossref] [PubMed]
- Hollern DP, Swiatnicki MR, Rennhack JP, et al. E2F1 Drives Breast Cancer Metastasis by Regulating the Target Gene FGF13 and Altering Cell Migration. Sci Rep 2019;9:10718. [Crossref] [PubMed]
- Sun H, Ma H, Zhang H, et al. Up-regulation of MELK by E2F1 promotes the proliferation in cervical cancer cells. Int J Biol Sci 2021;17:3875-88. [Crossref] [PubMed]
- Chen T, Yang Z, Liu C, et al. Circ_0078767 suppresses non-small-cell lung cancer by protecting RASSF1A expression via sponging miR-330-3p. Cell Prolif 2019;52:e12548. [Crossref] [PubMed]
- Zhang Q, Liu W, Zhang HM, et al. hTFtarget: A Comprehensive Database for Regulations of Human Transcription Factors and Their Targets. Genomics Proteomics Bioinformatics 2020;18:120-8. [Crossref] [PubMed]
- Farré D, Roset R, Huerta M, et al. Identification of patterns in biological sequences at the ALGGEN server: PROMO and MALGEN. Nucleic Acids Res 2003;31:3651-3. [Crossref] [PubMed]
- Messeguer X, Escudero R, Farré D, et al. PROMO: detection of known transcription regulatory elements using species-tailored searches. Bioinformatics 2002;18:333-4. [Crossref] [PubMed]
- Castro-Mondragon JA, Riudavets-Puig R, Rauluseviciute I, et al. JASPAR 2022: the 9th release of the open-access database of transcription factor binding profiles. Nucleic Acids Res 2022;50:D165-73. [Crossref] [PubMed]
- Wang J, Shi Q, Yuan TX, et al. Matrix metalloproteinase 9 (MMP-9) in osteosarcoma: review and meta-analysis. Clin Chim Acta 2014;433:225-31. [Crossref] [PubMed]
- Zhang M, Zhang X. Association of MMP-2 expression and prognosis in osteosarcoma patients. Int J Clin Exp Pathol 2015;8:14965-70. [PubMed]
- Gill J, Geller D, Gorlick R. HER-2 involvement in osteosarcoma. Adv Exp Med Biol 2014;804:161-77. [Crossref] [PubMed]
- Wang WG, Chen SJ, He JS, et al. The tumor suppressive role of RASSF1A in osteosarcoma through the Wnt signaling pathway. Tumour Biol 2016;37:8869-77. [Crossref] [PubMed]
- Xiao P, Xue L, Che LH, et al. Expression and roles of MICA in human osteosarcoma. Histopathology 2008;52:640-2. [Crossref] [PubMed]
- Yang H, Zhang Y, Zhou Z, et al. Snail-1 regulates VDR signaling and inhibits 1,25(OH)-D3 action in osteosarcoma. Eur J Pharmacol 2011;670:341-6. [Crossref] [PubMed]
- Owosho AA, Ladeji AM, Adesina OM, et al. SATB2 and MDM2 Immunoexpression and Diagnostic Role in Primary Osteosarcomas of the Jaw. Dent J (Basel) 2021;10:4. [Crossref] [PubMed]