Comparison of transbronchial biopsy techniques using needle and forceps biopsies in lung cancer for molecular diagnostics: a prospective, randomized crossover trial
Highlight box
Key findings
• Transbronchial needle aspiration (TBNA) biopsies of peripheral lung lesions provide adequate material for next-generation sequencing (NGS) compared with transbronchial forceps biopsies, despite a smaller median viable cell area.
What is known and what is new?
• Transbronchial needle and forceps biopsy methods have been used to retrieve samples of peripheral lung lesions for diagnostic yield. Whether either method is adequate for NGS in lung cancer has not been reported.
• This prospective, randomized, cross-over trial showed that transbronchial needle biopsies provide sufficient material for NGS.
What is the implication, and what should change now?
• TBNA can be used safely to retrieve samples for NGS.
• A larger case series should be performed to evaluate whether a clinical impact can be achieved with either biopsy method.
Introduction
Molecular pathologic diagnosis has become an essential step in the evaluation stage IV lung cancer due to the availability of targeted therapies for epidermal growth factor receptor (EGFR), anaplastic lymphoma kinase (ALK) and other mutations and the implementation of recommended therapies in national and international guidelines (1,2). Increasingly, molecular testing is becoming available at earlier stages of cancer, as new treatment options are approved at earlier stages of disease (3). With the advent of lung cancer screening programs, lung cancer may be detected at an earlier stage when peripheral pulmonary lesions are found in the absence of a central tumor mass. For these peripheral nodules, bronchoscopy with transbronchial biopsy is a routine diagnostic tool (4).
Two commonly used techniques for transbronchial tissue sampling are needle and forceps biopsies: transbronchial forceps biopsy (TBFB) is a simple, easy-to-learn technique that is particularly useful for lesions that infiltrate or are in close contact with the endobronchial wall. TBFB samples are typically larger, but carry a slightly higher risk of complications compared to needle biopsies, such as bleeding, pneumothorax, and airway obstruction (5). Transbronchial needle aspiration (TBNA) is a similar procedure that provides a simple, safe, and well-tolerated acquisition of small tissue samples from peripheral lung lesions with a smaller risk of complications (6). The advantage of TBNA lies in its deeper penetration into the lung parenchyma, increasing diagnostic yield of samples when the tumor has not infiltrated the bronchial wall (7-9). In the presence of thick-walled or calcified lesions, TBFB may be preferable. In high-risk patients or parabronchial tumor location TBNA can be used and has been shown to provide results when TBFB failed (10).
While both TBFB and TBNA have been used for decades to obtain tissue or cells from peripheral lung lesions, there is no data on whether TBNA provides as many viable tumor cells for molecular testing in direct comparison to TBFB. In this study, we aim to compare these specific transbronchial biopsy methods to determine if TBNA can provide sufficient material for next-generation sequencing (NGS) by using the median viable cell area (MVCA) as a surrogate parameter to assess sample quality in samples from peripheral lung lesions. We present this article in accordance with the CONSORT reporting checklist (available at https://tcr.amegroups.com/article/view/10.21037/tcr-23-2320/rc).
Methods
This was a prospective, single-center study conducted at University Hospital Regensburg, University of Regensburg, Regensburg, Germany, a tertiary hospital and certified lung cancer center of the German Cancer Society. Patients were eligible for this study if they presented with peripheral lung lesions suspicious of lung cancer for which bronchoscopy with transbronchial biopsy was deemed necessary. Peripheral lung lesions were defined as lesions that were not expected to be visible in the segmental bronchi by flexible bronchoscopy. Patient consent for this study was obtained prior to the examination, independent of the consent to the examination. This study was conducted in accordance with the Declaration of Helsinki (as revised in 2013). The study was approved by the institutional ethics board of the University of Regensburg (No.: 21-2463-101).
Patient randomization was performed with an online random number generator in a predetermined list (https://www.random.org/). Patients were randomized 1:1 to either TBFB or TBNA as the first biopsy technique and then crossed over to the other (Figure 1). Biopsies were obtained using an Olympus® EndoJawTM biopsy forceps (Model No. FB-211D, Olympus, Shinjuku, Tokyo, Japan) and a size 19-gauge Boston Scientific® eXcelonTM transbronchial aspiration needle (Model No. M 00564101, Boston Scientific, Marlborough, MA, USA). Samples were collected by both methods and sent to the pathology laboratory for diagnosis. The pathologist was blinded to the biopsy techniques used.
Lesion size was recorded as the largest diameter in axial computed tomography (CT)-imaging. The lesion site was recorded as the bronchial segment from which the tissue samples were obtained. All examinations were performed under fluoroscopic guidance and general anesthesia, as is standard practice at University Hospital Regensburg. When appropriate, endobronchial radial ultrasound and a guide sheath were used to assist in specimen collection, as determined by the physician. The study required three to five passes with each method, and samples were suspended in formaldehyde solution. When appropriate, TBFB was preceded by endobronchial ultrasound-guided TBNA (EBUS-TBNA) of the hilar and mediastinal lymph nodes for additional tissue sampling and nodal staging. However, these tissue samples were not used in the evaluation of the MVCA. All patients received a final fluoroscopy-guided chest X-ray at the end of the examination and a conventional chest X-ray 2 hours later to rule out pneumothorax.
Complications were recorded in categories of bleeding, pneumothorax and other. The most severe bleeding from each technique was documented on a scale of none, mild bleeding (self-limiting or manageable by bronchoscopic suction), moderate bleeding [requiring the additional use of medication (instilled cold saline solution or diluted epinephrine solution)] and severe (any surgical intervention, intensive care monitoring or death), as this has been feasible in other studies (11). Pneumothorax complications were categorized as none, no intervention and intervention indicated.
Pathological diagnosis of specimen was used initially to confirm tumor diagnosis and initiate therapy as needed. Samples slides were fixed and stained with hematoxylin and eosin, then digitized. A slide scanner was used for digitization, and slides were re-evaluated with a digital slide viewer (CaseViewer, 3DHISTECH Ltd, Budapest, Hungary). Using this software, the cells or cell clusters were separately marked to calculate their area and were quantified in µm2. Through summation of all marked areas, the total area of vital cells (TBNA) or tissue (TBFB) in the sample was calculated and recorded in mm2 (Figures 2,3). Sample analysis was performed by the same experienced pathologist to reduce inter-observer variability samples were re-faced prior to cutting the slides. A cell count threshold of 100 malignant cells was used to determine eligibility for molecular analysis. If less than 100 cells were visible, we did not proceed with the genetic analysis. If more cells were visible, NGS was performed. In all cases, 10 slides were prepared for microdissection of tumor cells. More slides were prepared in borderline cases. A custom NGS DNA-sequencing panel was used (nNGM-V22 Custom Qiaseg Panel Kit, QIAGEN, Venlo, The Netherlands), for molecular diagnostics of non-small cell lung cancer (NSCLC). DNA-sequencing was performed on the NextSeq system (Illumina Inc., San Diego, CA, USA). DNA concentration was measured using a Qubit fluorometer (Thermo Fisher Scientific Inc., Waltham, MA, USA).
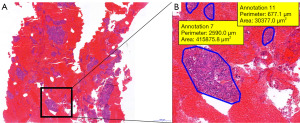
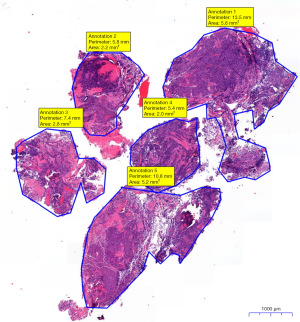
This trial assessed the MVCA of samples retrieved by TBNA for NGS by using MVCA as a surrogate parameter. The primary endpoint of this study was MVCA of TBNA compared to TBFB specimens. Secondary endpoints included complications in the categories of bleeding, pneumothorax, and other complications. This was a feasibility study to compare biopsy methods for MVCA. No previous data was available for MVCA of these biopsy methods. Therefore, no a priori sample size calculation was performed.
Statistical analysis
Statistical analysis was performed with IBM SPSS Version 29 (IBM, Armonk, NY, USA). Nonparametric tests were used to test the level of significance. Continuous variables are presented as median and interquartile range (IQR). Categorical variables are presented as numbers and percentages. A P value of <0.05 was considered statistically significant.
Results
Between August 2021 and April 2022, 17 patients were enrolled in the study, however in two patients it was not possible to reach the peripheral lesions and were considered screening failures (Figure 1). Fifteen patients were in the per-protocol group. Ten patients (66.7%) were male, five patients (33.3%) were female. Baseline characteristics are shown in Table 1. Median age at examination date was 74.0 years (IQR, 66.2–79.1 years). Six of 15 patients (40.0%) were enrolled in cohort 1, in which TBFB was performed first, followed by TBNA. Nine of 15 patients (60.0%) were enrolled in cohort 2. Patient enrollment was stopped when staffing changes would have interfered with data collection.
Table 1
Characteristics | Values |
---|---|
Gender, n (%) | |
Male | 10 (66.7) |
Female | 5 (33.3) |
Age (years), median (IQR) | 74.0 (66.2–79.1) |
Maximum lesion diameter | |
≤2.0 cm, n (%) | 1 (6.7) |
2.1–3.0 cm, n (%) | 3 (20.0) |
>3.0 cm, n (%) | 11 (73.3) |
Overall (cm), median (IQR) | 4.6 (3.0–5.3) |
Lesion localization, n (%) | |
Right upper lobe | 3 (20.0) |
Right middle lobe | 0 (0.0) |
Right lower lobe | 5 (33.3) |
Left upper lobe | 4 (26.7) |
Left lingular lobe | 2 (13.3) |
Left lower lobe | 1 (6.7) |
IQR, interquartile range.
Left upper lobe segment 3 and right lower lobe segment 6 were the most common biopsy sites, with 4/15 (26.7%) and 3/15 (20.0%) biopsies, respectively (Figure 4). Mean number of passes was 4.5 with TBFB and 4.2 with TBNA.
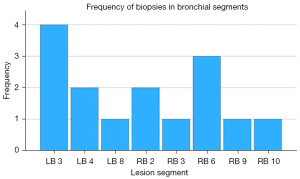
Histopathologic results are shown in Table 2. Malignancy was diagnosed in 9/15 cases (60.0%) by TBFB and TBNA each. When combined, a malignant diagnosis was made in 11 cases (73.3%), of which 7 (63.6%) were confirmed in both specimens. In nine of these 11 cases (81.8%) primary lung cancer was identified with 1 case of small cell lung cancer (SCLC) and eight cases of NSCLC (9.1% and 72.7%, respectively), which were evenly divided with four cases of squamous cell carcinoma and four cases of adenocarcinoma each. In all adenocarcinomas, malignancy was confirmed in both TBFB and TBNA, whereas in squamous cell carcinoma, malignancy was only confirmed in both biopsies in two of four cases. Final lung cancer stages at time of diagnosis are shown in Table S1.
Table 2
Histopathologic diagnosis | No. of patients (%) | No. diagnosis confirmed in TBFB | No. diagnosis confirmed in TBNA |
---|---|---|---|
Malignant | |||
Pulmonary adenocarcinoma | 4 (26.7) | 4 | 4 |
Pulmonary squamous cell carcinoma | 4 (26.7) | 3 | 3 |
Pancreatic cancer | 1 (6.7) | 1 | 0 |
Small cell lung cancer | 1 (6.7) | 1 | 1 |
Urothelial carcinoma | 1 (6.7) | 0 | 1 |
Total | 11 (73.3) | 9 | 9 |
Non-malignant | |||
Fibrotic tissue | 2 (13.3) | 2 | 1 |
Cryptogenic organizing pneumonia | 1 (6.7) | 1 | 1 |
Squamous cell dysplasia | 1 (6.7) | 0 | 1 |
Total | 4 (26.7) | 3 | 3 |
Total | 15 (100.0) | 12 | 12 |
TBFB, transbronchial forceps biopsy; TBNA, transbronchial needle aspiration.
There are two false negative cases: in one case of squamous cell dysplasia, follow-up CT-guided lung biopsy confirmed squamous cell carcinoma. In the two cases of fibrotic tissue, one patient’s positive positron emission tomography (PET)-CT scan led to a clinical diagnosis of recurrence of previously resected bronchial adenocarcinoma, the other patient denied further evaluation and was discharged against medical advice. On a follow-up CT scan for an unrelated issue at 14 months, this patient’s targeted pulmonary lesion remained stable in size.
Median lesion size was 4.6 cm (IQR, 3.0–5.3 cm) and 11/15 (73.3%) of lesions were larger than 3.0 cm. Lesion diameters of less than or equal to 2.0 cm provided no sample in TBNA and a small MVCA of 1.99 mm2 in TBNA, while lesion diameters between 2.1 and 3.0 cm provided the largest MVCA in TBFB [16.30 mm2 (IQR, 1.24–17.97 mm2)] and TBNA [9.54 mm2 (IQR, 6.01–13.72 mm2), Table 3]. Lesions larger than 3.0 cm provided a significant larger MVCA in TBFB than TBNA [MVCA 9.80 mm2 (IQR, 5.69–10.14 mm2) and 1.44 mm2 (IQR, 0.14–4.71 mm2), P=0.001, respectively].
Table 3
Lesion size | Biopsy method | P value | ||||
---|---|---|---|---|---|---|
TBFB | TBNA | |||||
No. of patients | Viable cell area, mm2 | No. of patients | Viable cell area, mm2 | |||
Maximum lesion diameter | ||||||
≤2.0 cm | 1 | 1.99 (1.99–1.99) | 1 | 0.00 (0.00–0.00) | >0.99 | |
2.1–3.0 cm | 3 | 16.30 (1.24–17.97) | 3 | 9.54 (6.01–13.72) | 0.70 | |
>3.0 cm | 11 | 9.80 (5.69–10.14) | 11 | 1.44 (0.14–4.71) | 0.001 | |
Total | 15 | 9.80 (2.70–10.39) | 15 | 2.70 (0.14–8.21) | 0.008 |
Data are presented as median (interquartile range). TBFB, transbronchial forceps biopsy; TBNA, transbronchial needle aspiration.
Overall, MVCA was significantly larger in samples obtained by TBFB than in TBNA samples [TBFB-MVCA 9.80 mm2 (IQR, 2.70–10.39 mm2) vs. TBNA-MVCA 2.70 mm2 (IQR, 0.14–8.21 mm2), P=0.008, Table 3, Figure S1]. No viable cells were found in two TBNA samples, which may account for the large median shift in TBNA sample size. TBNA-MVCA was significantly larger when following TBFB in cohort 1 [7.11 mm2 (IQR, 4.71–9.54 mm2)], than in cohort 2 [1.14 mm2 (IQR, 0.03–2.70 mm2), P=0.036]. In TBFB, the differences between cohorts were not significant.
The primary endpoint of TBNA-MVCA was not met, as there is significant difference in MVCA, yet both TBFB and TBNA provided material for NGS when MVCA was measurable. In the NSCLC samples, median DNA concentration after enrichment was 6.23 ng/µL (IQR, 2.98–18.80 ng/µL). Individual molecular diagnostic test results for NSCLC are shown in Table S2.
After TBNA, we observed mild bleeding in four patients (26.7%) and moderate bleeding in two patients (13.3%). There were fewer cases of bleeding in TBFB, with mild and moderate bleeding observed in two patients (13.3%) each. No severe bleeding was observed. There was no statistical difference in the incidence of bleeding between the two methods (P=0.857, Fisher’s exact test, Table S3).
No pneumothorax was observed during or 2 hours after the examination. In only one patient was a pneumothorax observed on a PET scan the day after the examination, and we were unable to determine which biopsy method might have caused this. Other complications were not reported. No significant difference was found between the two cohorts in the secondary outcomes.
Discussion
In our study, both TBFB and TBNA had a diagnostic yield of 9/15 (60.0%) each. This yield increased to 11/15 (73.3%) when used in combination. When used in combination and disregarding malignancy, both methods provided enough material to be considered sufficient for molecular testing by the investigating pathologist in all cases. These results are in contrast to those reported in literature, where TBNA was reported to have a higher diagnostic yield than TBFB (9). In their meta-analysis, Mondoni et al. showed an overall TBNA yield of 0.53 [95% confidence interval (CI): 0.44–0.61], with a higher accuracy when compared directly to TBFB [0.60 (95% CI: 0.49–0.71) vs. 0.45 (95% CI: 0.37–0.54), respectively] (12). Other publications have reported a diagnostic yield of up to 93.4% using a combination of different biopsy techniques (13).
MVCA was lowest in small diameter lesions of ≤2.0 cm (Table 3), which is consistent with the available literature, where diagnostic yield is greatly improved in lesions larger than 2.0 cm (14-16). MVCA decreased in tumors greater than 3.0 cm of lesions size. This decrease may be due to the fact that these specimens may contain non-diagnostic material from the surrounding tumor environment rather than viable tumor cells, but this study was not designed to account for this effect.
Interestingly, although MVCA of samples provided by TBNA was significantly smaller at 45.4% of that obtained by TBFB, the samples were still sufficient for molecular pathology testing to be performed. Since genetic testing requires a sufficient amount of tumor DNA, even a large sample may be insufficient if it contains too much non-tumor or stromal tissue whose DNA content interferes with genetic testing. Conversely, a small sample containing only tumor cells and non-nucleated cells such as erythrocytes or connective tissue may provide sufficient tissue for molecular pathology testing, resulting in a lower diagnostic threshold. It could be suggested that TBNA causes less tissue damage than TBFB while providing similar diagnostic results and tissue volume for molecular testing. Figure 2 shows an aspirated sample from a TBNA that contains large areas of clustered tumor cells, surrounded by a large volume of non-nucleated erythrocytes, which do not contain DNA and therefore do not interfere with molecular genetic testing. When we compared our samples, we found that while TBFB (Figure 3) provided larger areas of viable tissue, the samples also contained more stromal, connective or non-tumorous lung tissue, causing greater impurities.
TBNA-MVCA was significantly larger in cohort 1, where TBFB was performed first. One reason for this may be that once TBFB has been performed first, TBNA can then be targeted more accurately, thereby increasing the chance of obtaining tissue samples.
The incidence of bleeding in our study was higher than that reported in the literature, where rates of clinically significant bleeding have been reported in 1.9% of patients undergoing transbronchial lung biopsy and in up to 11% of patients with risk factors for coagulopathy (17,18). In our study, TBNA biopsies led to mild bleeding in 26.7%. This result may be disproportionately large due to the small number of patients enrolled, yet our study showed no significant difference in these secondary endpoints.
In literature, pneumothorax has been reported in up to 6% of transbronchial lung biopsies (19). In our study, one pneumothorax was diagnosed on the day after the biopsy, which we considered a rare case of delayed pneumothorax post bronchoscopy (20). Although we suspect that this was a complication of the bronchoscopy, it is impossible to determine which biopsy method, if any, was responsible, therefore it was not included in the statistical analysis.
We provided a structured basis for a randomized controlled trial that allowed us to limit methodological error by randomizing the order of the biopsy methods. Complications were comprehensively recorded and no significant difference in was found between the two methods. We have shown that despite smaller MVCA, TBNA still provides sufficient material for molecular pathology. To our knowledge, this is the first study to compare the area of viable tissue cells, cell clusters or tissue area by transbronchial biopsy or aspiration method for peripheral lung lesions. Kunimasa et al. recently reported an overview of sampling methods for NGS for lung cancer patients, but they compared the transbronchial biopsies with EBUS-TBNA and CT-guided biopsies, and it is not known whether forceps or needle sampling was used (21). Another study by Kage et al. showed that small samples are suitable for targeted NGS and found that diagnostic results are similar to resection, but only forceps biopsies were obtained (22).
There are few limitations to consider. This is a single-center study with only a small number of patients enrolled, in part due to a reduced number of patients diagnosed with suspected malignant lung nodules during the coronavirus disease 2019 (COVID-19) pandemic, during which testing of patients with cancer was greatly reduced (23). In addition, in our institution, most of the primary sampling is performed with EBUS-TBNA of mediastinal or hilar lymph nodes, reducing the number of cases in which initial transbronchial biopsies are performed.
There is no standardized approach to transbronchial biopsies. This can lead to high inter-examiner variability, potentially reducing diagnostic yield when applied in a real-world setting, especially when considering examiner experience. Diagnostic yield of endobronchial-ultrasound guided TBNA has been shown to increase rapidly after only 13 procedures, and European Respiratory Society (ERS)/American Thoracic Society (ATS) guidelines recommend that at least 25 TBNA cytology biopsies be performed under expert supervision to become proficient in specimen collection (24,25). We attempted to reduce confounding by having the procedures performed by an examiner who met these requirements.
Whether the use of general anesthesia has an impact on diagnostic yield is controversial. Although it is local policy at our institution, studies have shown no difference in diagnostic yield when compared to those performed with moderate sedation (26).
Sample quality assessment for NGS was performed by the same reviewing pathologist, which also reduced inter-examiner variability, which has been shown to influence NGS success rates (27). Nevertheless, the required tissue volume for NGS has not been determined and depends on the platform used (28,29). As genotyping is highly dependent on cytological preparation, fixation and staining techniques, there are no clear cut-off values, which may reduce reproducibility of these results (28,30-32). No additional slides were prepared for MVCA analysis, as we felt this may influence real world results. Therefore, whether larger MVCA had an impact on re-facing the samples, was not tested and the study was not designed for this. Whether a larger MVCA correlates to better results should be discussed in future studies.
A limitation of this study is that we did not compare the yield of DNA concentration between the two methods. Instead, the primary focus was to assess sample quality using MVCA for TBFB and TBNA. Future research should focus on DNA sampling from individual biopsy methods.
Immune cell scoring for PD-L1 is usually performed on histologic tissue samples. Smaller TBNA needle sizes may only provide material for cytologic analysis. While there is emerging data on the use of cytologic specimen for immune cell scoring, this requires specialized processing and may be associated with higher inter-observer variability (33-37). ERS/ATS guidelines suggest that needles of size 19 G and larger be used for histologic tissue samples, as was done in this study (24). Therefore, it could be suggested that when smaller gauges are used, or the lesion is accessible, TBNA should be combined with another method of tissue sampling, such as TBFB.
We compared the MVCA of TBFB and TBNA samples and showed that the overall yield is high. This warrants further evaluation to improve the diagnostic outcome of transbronchial biopsies and patient safety.
Conclusions
In our study, the MVCA was significantly larger for TBFB than for TBNA. Despite the smaller MVCA, the diagnostic yield was the same between the two methods, and both methods provided enough material to be considered sufficient for NGS. We argue that TBNA samples may contain less non-tumor tissue, which causes less interference in molecular pathology testing. There was no significant difference in complications between the two methods. Therefore, TBNA seems to be a valuable tool for modern diagnosis and staging of peripheral lung lesions. Larger studies are needed to confirm our preliminary results.
Acknowledgments
Preliminary data of this study was presented at the 63rd Congress of the German Respiratory Society in Düsseldorf, Germany in March 2023.
Funding: None.
Footnote
Reporting Checklist: The authors have completed the CONSORT reporting checklist. Available at https://tcr.amegroups.com/article/view/10.21037/tcr-23-2320/rc
Data Sharing Statement: Available at https://tcr.amegroups.com/article/view/10.21037/tcr-23-2320/dss
Peer Review File: Available at https://tcr.amegroups.com/article/view/10.21037/tcr-23-2320/prf
Conflicts of Interest: All authors have completed the ICMJE uniform disclosure form (available at https://tcr.amegroups.com/article/view/10.21037/tcr-23-2320/coif). The authors have no conflicts of interest to declare.
Ethical Statement: The authors are accountable for all aspects of the work in ensuring that questions related to the accuracy or integrity of any part of the work are appropriately investigated and resolved. The study was conducted in accordance with the Declaration of Helsinki (as revised in 2013). The study was approved by institutional ethics board of the University of Regensburg (No.: 21-2463-101) and informed consent was obtained from all individual participants.
Open Access Statement: This is an Open Access article distributed in accordance with the Creative Commons Attribution-NonCommercial-NoDerivs 4.0 International License (CC BY-NC-ND 4.0), which permits the non-commercial replication and distribution of the article with the strict proviso that no changes or edits are made and the original work is properly cited (including links to both the formal publication through the relevant DOI and the license). See: https://creativecommons.org/licenses/by-nc-nd/4.0/.
References
- Oncology Guideline Program (German Cancer Society, German Cancer Aid, AWMF). S3-Guideline on Prevention, Diagnostics, Therapy and Follow-Up Care of Lung Cancer, extended version 2.1, 2022. AWMF-Register number: 020/007OL. 2022.
- Hendriks LE, Kerr KM, Menis J, et al. Oncogene-addicted metastatic non-small-cell lung cancer: ESMO Clinical Practice Guideline for diagnosis, treatment and follow-up. Ann Oncol 2023;34:339-57. [Crossref] [PubMed]
- Aggarwal C, Bubendorf L, Cooper WA, et al. Molecular testing in stage I-III non-small cell lung cancer: Approaches and challenges. Lung Cancer 2021;162:42-53. [Crossref] [PubMed]
- Fantin A, Manera M, Patruno V, et al. Endoscopic Technologies for Peripheral Pulmonary Lesions: From Diagnosis to Therapy. Life (Basel) 2023;13:254. [Crossref] [PubMed]
- Facciolongo N, Patelli M, Gasparini S, et al. Incidence of complications in bronchoscopy. Multicentre prospective study of 20,986 bronchoscopies. Monaldi Arch Chest Dis 2009;71:8-14. [PubMed]
- Gasparini S, Mei F, Bonifazi M, et al. Bronchoscopic diagnosis of peripheral lung lesions. Curr Opin Pulm Med 2022;28:31-6. [Crossref] [PubMed]
- Shure D, Fedullo PF. Transbronchial needle aspiration of peripheral masses. Am Rev Respir Dis 1983;128:1090-2. [PubMed]
- Yung RC. Tissue diagnosis of suspected lung cancer: selecting between bronchoscopy, transthoracic needle aspiration, and resectional biopsy. Respir Care Clin N Am 2003;9:51-76. [Crossref] [PubMed]
- Franke KJ, Nilius G, Rühle KH. Bronchoscopic diagnosis of peripheral pulmonary foci. Dtsch Med Wochenschr 2006;131:2229-33. [Crossref] [PubMed]
- Lee H, Kim CW. Applicability of TBNA to peripheral lung lesion when transbronchial biopsy fails. European Respiratory Journal 2016;48:PA3726.
- Ernst A, Eberhardt R, Wahidi M, et al. Effect of routine clopidogrel use on bleeding complications after transbronchial biopsy in humans. Chest 2006;129:734-7. [Crossref] [PubMed]
- Mondoni M, Sotgiu G, Bonifazi M, et al. Transbronchial needle aspiration in peripheral pulmonary lesions: a systematic review and meta-analysis. Eur Respir J 2016;48:196-204. [Crossref] [PubMed]
- Kawaraya M, Gemba K, Ueoka H, et al. Evaluation of various cytological examinations by bronchoscopy in the diagnosis of peripheral lung cancer. Br J Cancer 2003;89:1885-8. [Crossref] [PubMed]
- Rivera MP, Mehta AC, Wahidi MM. Establishing the diagnosis of lung cancer: Diagnosis and management of lung cancer, 3rd ed: American College of Chest Physicians evidence-based clinical practice guidelines. Chest 2013;143:e142S-65S.
- Schreiber G, McCrory DC. Performance Characteristics of Different Modalities for Diagnosis of Suspected Lung Cancer*: Summary of Published Evidence. Chest 2003;123:115S-28S. [Crossref] [PubMed]
- Han Y, Kim HJ, Kong KA, et al. Diagnosis of small pulmonary lesions by transbronchial lung biopsy with radial endobronchial ultrasound and virtual bronchoscopic navigation versus CT-guided transthoracic needle biopsy: A systematic review and meta-analysis. PLoS One 2018;13:e0191590. [Crossref] [PubMed]
- Kozak EA, Brath LK, Do . "screening" coagulation tests predict bleeding in patients undergoing fiberoptic bronchoscopy with biopsy? Chest 1994;106:703-5. [Crossref] [PubMed]
- Cordasco EM Jr, Mehta AC, Ahmad M. Bronchoscopically induced bleeding. A summary of nine years' Cleveland clinic experience and review of the literature. Chest 1991;100:1141-7. [Crossref] [PubMed]
- Du Rand IA, Blaikley J, Booton R, et al. British Thoracic Society guideline for diagnostic flexible bronchoscopy in adults: accredited by NICE. Thorax 2013;68:i1-i44. [Crossref] [PubMed]
- Alsaggaf M, Khalofa A, Khosla R. Delayed Pneumothorax Post Transbronchial Biopsy. Chest 2020;158:A1898-9. [Crossref]
- Kunimasa K, Matsumoto S, Nishino K, et al. Comparison of sampling methods for next generation sequencing for patients with lung cancer. Cancer Med 2022;11:2744-54. [Crossref] [PubMed]
- Kage H, Kohsaka S, Shinozaki-Ushiku A, et al. Small lung tumor biopsy samples are feasible for high quality targeted next generation sequencing. Cancer Sci 2019;110:2652-7. [Crossref] [PubMed]
- Van Haren RM, Delman AM, Turner KM, et al. Impact of the COVID-19 Pandemic on Lung Cancer Screening Program and Subsequent Lung Cancer. J Am Coll Surg 2021;232:600-5. [Crossref] [PubMed]
- Bolliger CT, Mathur PN, Beamis JF, et al. ERS/ATS statement on interventional pulmonology. European Respiratory Society/American Thoracic Society. Eur Respir J 2002;19:356-73. [PubMed]
- Wahidi MM, Hulett C, Pastis N, et al. Learning experience of linear endobronchial ultrasound among pulmonary trainees. Chest 2014;145:574-8. [Crossref] [PubMed]
- Fiorelli A, Pecoraro A, Carlucci A, et al. Best Evidence Topic: Does the Depth of Sedation Affect Diagnostic Yield of Endobronchial Ultrasound–guided Transbronchial Needle Aspiration? Clinical Pulmonary Medicine 2020;27:105-12. [Crossref]
- Roy-Chowdhuri S, Goswami RS, Chen H, et al. Factors affecting the success of next-generation sequencing in cytology specimens. Cancer Cytopathol 2015;123:659-68. [Crossref] [PubMed]
- Chen H, Luthra R, Goswami RS, et al. Analysis of Pre-Analytic Factors Affecting the Success of Clinical Next-Generation Sequencing of Solid Organ Malignancies. Cancers (Basel) 2015;7:1699-715. [Crossref] [PubMed]
- Cho M, Ahn S, Hong M, et al. Tissue recommendations for precision cancer therapy using next generation sequencing: a comprehensive single cancer center's experiences. Oncotarget 2017;8:42478-86. [Crossref] [PubMed]
- Dolinar A, Grubelnik G, Srebotnik-Kirbiš I, et al. Optimization of pre-analytical and analytical steps for DNA and RNA analysis of fresh cytology samples. Cancer Med 2022;11:4021-32. [Crossref] [PubMed]
- Roy-Chowdhuri S, Stewart J. Preanalytic Variables in Cytology: Lessons Learned From Next-Generation Sequencing-The MD Anderson Experience. Arch Pathol Lab Med 2016;140:1191-9. [Crossref] [PubMed]
- Bellevicine C, Malapelle U, de Luca C, et al. EGFR analysis: current evidence and future directions. Diagn Cytopathol 2014;42:984-92. [Crossref] [PubMed]
- Lindeman NI, Cagle PT, Aisner DL, et al. Updated Molecular Testing Guideline for the Selection of Lung Cancer Patients for Treatment With Targeted Tyrosine Kinase Inhibitors: Guideline From the College of American Pathologists, the International Association for the Study of Lung Cancer, and the Association for Molecular Pathology. Arch Pathol Lab Med 2018;142:321-46. [Crossref] [PubMed]
- Sorber L, Claes B, Zwaenepoel K, et al. Evaluation of Cytologic Sample Preparations for Compatibility With Nucleic Acid Analysis. Am J Clin Pathol 2022;157:293-304. [Crossref] [PubMed]
- Fox AH, Nishino M, Osarogiagbon RU, et al. Acquiring tissue for advanced lung cancer diagnosis and comprehensive biomarker testing: A National Lung Cancer Roundtable best-practice guide. CA Cancer J Clin 2023;73:358-75. [Crossref] [PubMed]
- Tsao MS, Kerr KM, Kockx M, et al. PD-L1 Immunohistochemistry Comparability Study in Real-Life Clinical Samples: Results of Blueprint Phase 2 Project. J Thorac Oncol 2018;13:1302-11. [Crossref] [PubMed]
- Marletta S, Fusco N, Munari E, et al. Atlas of PD-L1 for Pathologists: Indications, Scores, Diagnostic Platforms and Reporting Systems. J Pers Med 2022;12:1073. [Crossref] [PubMed]