Apigenin suppresses the low oxaliplatin-induced epithelial-mesenchymal transition in oral squamous cell carcinoma cells via LINC00857
Highlight box
Key findings
• Apigenin can effectively suppress the metastasis of oral squamous carcinoma cells (OSCCs) induced by low-dose oxaliplatin (OXA) through inhibiting the level of LINC00857.
What is known and what is new?
• Apigenin is a natural flavonoid compound with proven antitumor activity.
• Apigenin exhibits a synergistic effect with OXA and can enhance the inhibitory effect of OXA on OSCC proliferation. Apigenin suppressed the expression of the long non-coding RNA (lncRNA) LINC00857 in OSCC.
What is the implication, and what should change now?
• The present study has contributed to enhancing the comprehension of the pharmacological mechanism of apigenin, thereby offering novel insights for its potential clinical implementation in the management of OSCC.
Introduction
Chemotherapy is the most common therapeutic mode for the treatment of tumors in clinical setting, which can effectively suppress the tumor proliferation and prolong the survival of patients, among which oxaliplatin (OXA) stands out as one of the most widely utilized options (1,2). However, certain studies have indicated that under specific circumstances, the administration of chemotherapy drugs may potentially induce tumor cell metastasis, particularly when administered at lower dosages (3,4). The current trend in research is increasingly focused on investigating the adverse effects of low-dose chemotherapeutic agents in tumor treatment and exploring effective strategies to manage these side effects.
Currently, there is a growing research interest in natural product small molecules, with many demonstrating remarkable anticancer effects or potential as adjuncts to existing anticancer therapies (5). Flavonoids are a class of small molecules that exhibit a diverse range of anticancer effects, among which apigenin stands out as a typical flavonoid molecule with potent anticancer properties (6,7). Researchers in the 1950s initially investigated apigenin and analyzed its impact on histamine secretion, subsequently discovering its inhibitory effect on cell proliferation and gene mutation, consequently establishing its association with anticancer effects (8,9). The efficacy of apigenin in inhibiting tumor cell proliferation, metastasis, and invasion, as well as inducing apoptosis and autophagy, has been demonstrated through the activation of signaling pathways including phosphatidylinositol 3-kinase (PI3K)/protein kinase B (PKB), transcription 3 (STAT3), and Wingless/integrase-1 (WNT) (10).
The process of converting epithelial cells into mesenchymal cells is referred to as epithelial-mesenchymal transition (EMT) (11,12). The phenotypic changes in tumor cells during epithelial tumor metastasis are primarily induced by environmental stimuli, enabling them to adapt to various encountered microenvironments such as intercellular matrices, components of body fluids, or blood (13-15). For oral squamous carcinoma cells (OSCCs), EMT plays an essential role in their local recurrence and lymph node metastasis, affecting patient survival by regulating EMT associated factors (16).
Recently, multiple studies have indicated that the inhibitory effects of low-dose platinum-based chemotherapeutic agents on tumor cells are limited. Furthermore, low-dose platinum-based chemotherapeutic agents could also induce EMT in tumor cells and promote their metastasis (17,18). In the case of oral tumors, these chemotherapeutic agents exert their action on tumor cells via the bloodstream, and as the distance between the cells and tumor blood vessels increases, the effective concentration of the drug decreases, therefore, it is crucial to address the potential adverse effects of low-dose chemotherapeutic agents on tumor cells from a clinical perspective.
Therefore, this study was proposed to investigate the synergistic inhibitory effect of apigenin and OXA on OSCC proliferation as well as the potential of apigenin in suppressing EMT induced by low-dose OXA. We present this article in accordance with the MDAR reporting checklist (available at https://tcr.amegroups.com/article/view/10.21037/tcr-23-2335/rc).
Methods
Cell culture
OSCC HSC-3 (Chinese Academy of Sciences, Shanghai, China) cultured in Dulbecco’s Modified Eagle Medium (DMEM) (Hyclone, Logan, UT, USA) supplemented with penicillin, streptomycin, and 10% bovine serum (Hyclone) and maintained at a temperature of 37 ℃ in a 5% CO2 atmosphere.
Cell viability assay
The resistance of apigenin to HSC-3 was examined utilizing the 3-(4,5-dimethyl-2-thiazolyl)-2,5-diphenyl-2-H-tetrazolium bromide (MTT) assay. Cells were vaccinated in a 96-well plate at a density of 5,000 cells/well and incubated overnight. Triplicate experiments were performed. After 48 h of drug administration, MTT solution was supplement into each flask. Formamide crystals were dissolved through incubation for 4 h in dimethyl sulfoxide (DMSO) (Sigma-Aldrich, Saint Louis, MO, USA). After discarding the supernatant, the formazan crystals were dissolved by adding 150 µL of DMSO. A microplate reader (Multiskan™ FC, USA) was utilized to analyze the absorbance at 590 nm and the 50% inhibitory concentration (IC50) was determined. CompuSyn software was utilized to calculate the synergistic effect, and the presence of synergism was indicated when the combination index (CI) of the combined treatment group was less than 1 for different doses. OXA and cisplatin (DDP) were purchased from MedChemExpress, USA. Apigenin was purchased from Push Bio-Technology, China.
Colony formation assay
The cells were dissociated using 0.25% trypsin and subsequently into individual cells during the logarithmic growth phase. These individual cells were then resuspended in complete medium in which 10% fetal bovine serum was added and quantified for cell counting purposes. The experimental group was inoculated in a 6-well plate at a density of 400 cells per well, and 4 mL of pre-warmed complete medium which contained 10% fetal bovine serum at room temperature was added and mixed gently. After an incubation period of 14 days in a cell incubator, the supernatant was aspirated and washed with phosphate-buffered saline (PBS) twice. The cells were subsequently fixed by adding paraformaldehyde to each well for a duration of 20 minutes, followed by one wash with PBS after removing the paraformaldehyde. Finally, crystal violet staining was performed on the fixed cells for a duration of 10 minutes. Following several washes with PBS solution, the cells were air-dried and photographed.
Wound-healing assay
A wound healing assay was conducted with HSC-3 cells to evaluate alterations in cell motility and migration. After cells were cultured to confluence in a 48-well plate, a pipette tip was utilized to generate a scratch on the cell monolayer, which was subsequently rinsed with PBS. Images were captured at 0, 24, and 48 hours after drug treatment [PBS, OXA (5 µM), apigenin (40 µM) + OXA (5 µM)] under a microscope.
Transwell assays
The invasiveness of HSC-3 cells was assessed utilizing a transwell assay. Cells were suspended with three different concentrations of medium [control, OXA (5 µM), apigenin (40 µM) + OXA (5 µM)]. Subsequently, the cells were inoculated onto an 8 µm polyethylene terephthalate filter membrane (Corning, NY, USA) that had been pre-coated with matrix gel (BD, NJ, USA), while the lower chamber of the transwell was filled with approximately 500 mL of culture medium. Following a 30-minute fixation with 4% paraformaldehyde and subsequent staining with 0.1% crystal violet (Sigma-Aldrich) for 20 minutes, the invading cells were meticulously observed and manually enumerated under an inverted microscope at a magnification of one hundred-fold.
Three-dimensional culture assay
Cancer cells from different groups were inoculated into a pre-coated Matrigel 48-well plate and cultured in 250 µL of DMEM supplement with 10% fetal bovine serum for 24 hours. The test tubes were imaged using a light microscope to capture visual representations. An assessment of the tube structure was performed to evaluate its angiogenic potential.
Immunofluorescence assays
Cells were processed [control, OXA (5 µM), apigenin (40 µM) + OXA (5 µM)], followed by overnight culture and subsequent processing using methanol and Triton X-100. After 24 h of incubation, cells were washed twice with PBS, followed by fixation with 10% cold formalin (−20 ℃) and then blocked with FBS (5% bull serum albumin and 0.1%Tritonx-100) for 1 h. Cells were then incubated in a solution containing a 1:100 dilution of E-cadherin (ab227639, Abcam, Cambridge, UK) and vimentin (ab8978, Abcam) antibody for 1h at room temperature. After three washes lasting 20 minutes each, the cells were incubated with a secondary antibody at a dilution of 1:200 for 30 minutes. The staining process was concluded by incubating the sample with 4,6-diamidino-2-phenylindole for a duration of 10 min and wash twice with PBS, followed by visualization using a laser scanning confocal microscope (Nikon, Tokyo, Japan).
RNA isolation, reverse transcriptase-quantitative polymerase chain reaction (RT-qPCR) and cell transfection
The extraction and reverse transcription of total RNA from cultured cells were performed using the kit (Yeasen, Shanghai, China). The relative RNA levels were quantified with glyceraldehyde-3-phosphate dehydrogenase (GAPDH) serving as an internal reference control. The resulting complementary DNA served as a template for subsequent qPCR analysis, and the data were analyzed using the 2-ΔΔCq method. The primers are as follows, LINC00342: forward 5'-TGCTGAGTAACTGTGATTCCC-3' and reverse 5'-CTTGGCTTCTCCCTCTAGTTG-3', LINC01273: forward 5'-AGTCTCTGTTGCGGTGTTC-3' and reverse 5'-TGTTTTCCAGGTCCATAGGTG-3', LINC00630: forward 5'-TGCAGTGAACATGGGAGTAC-3' and reverse 5'-AATCTGGCAAAGAGGGACTG-3', LINC00857: forward 5'-TCCGTTAAGCACCAGAAGTC-3' and reverse 5'-AGCAATATGGAAGGGAATGGAG-3'.
LINC00857 was overexpressed with pcDNA3.1, blank pcDNA-3.1 vector was used as control. Transfection of all constructed plasmids into cells was conducted and used for subsequent studies after 48 hours of transfection. The efficiency of overexpression was examined with RT-qPCR.
Statistical analysis
SPSS 22.0 software was utilized for statistical analysis. Three replications were set up for each experiment and data were collected and organized and expressed as mean ± standard deviation (SD). The t-test, one-way analysis of variance (ANOVA), or two-way ANOVA were employed to evaluate and compare group differences, with a significance level set at P≤0.05.
Results
Apigenin enhanced the anticancer effects of platinum-based chemotherapeutic agents on OSCC
Figure 1A illustrates the molecular structure of apigenin. In this study, apigenin was combined with DDP and OXA to act on OSCC HSC-3 cell line, respectively. The results demonstrated that the addition of apigenin further decreased the IC50 value of platinum-based chemotherapeutic agents on HSC-3 cells in a dose-dependent manner, as illustrated in Figure 1B,1C. Moreover, the inhibitory effects of apigenin, DDP/OXA, and DDP/OXA combined with apigenin on HSC-3 cell proliferation were counted, and the results showed that apigenin exerted inhibitory effects on HSC-3 cells, while the combination with platinum-based chemotherapeutic agents enhanced these inhibitory effects on tumor cell proliferation even more effectively. The effect of the drug combination was statistically analyzed using CompuSyn software, and the results revealed that the CI of the combination of apigenin and DDP/OXA was less than 1 at the medium and low drug concentrations, and that the efficacy of the two drugs was synergistic, as shown in Figure 1D,1E.
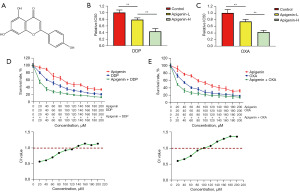
Low-dose OXA promoted metastasis of HSC-3 cells
The effects of OXA on HSC-3 cells at different concentrations were investigated using the clone formation assay, and the results demonstrated that the high dosage of OXA (40 µM) exhibited a significant inhibitory effect on tumor cell proliferation, while the inhibitory effect of low-dose OXA (5 µM) was limited, as shown in Figure 2A. The further results of cellular morphology showed that, compared with the HSC-3 cells in the control group, the pseudopods of the tumor cells in low-dose OXA group (5 µM) were elongated and the cells were spindle shaped, as shown in Figure 2B, suggesting that the metastatic potential of the tumor cells might be enhanced by the low concentration of OXA. Furthermore, cell wound healing assay results revealed that the low concentration of OXA promoted the migratory ability in HSC-3 cells than the control group, as illustrated in Figure 2C,2D.
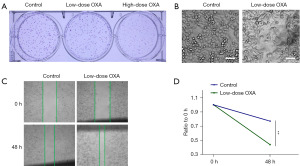
The metastasis promotion induced by low concentrations of OXA in HSC-3 was inhibited by apigenin
The effects of apigenin in combination with low concentrations of OXA on HSC-3 cells were investigated through cell wound healing, transwell, tumor cell angiogenesis, and EMT marker immunofluorescence assays. The results demonstrated that apigenin significantly suppressed the migration-promoting effect of low concentration of OXA on HSC-3 cells (Figure 3A,3B), meanwhile, it could also significantly inhibit the invasion of tumor cells (Figure 3C,3D). Angiogenesis assay revealed that low concentrations of OXA promoted the vasculogenic capacity of tumor cells in comparison to the control group, while this trend was inhibited by apigenin, as shown in Figure 3E,3F. EMT is an essential phenomenon in the metastatic process of tumor cells, and E-cadherin and Vimentin are epithelial phenotype marker molecules and mesenchymal phenotype marker molecules, respectively. Immunofluorescence experiments showed that low concentrations of OXA upregulated Vimentin protein expression while downregulating E-cadherin protein expression in HSC-3 cells than the control group, indicating the promotion of EMT by low concentrations of OXA. In contrast, the promotion of EMT was significantly inhibited by the combination of apigenin and low concentrations of OXA, and the results are shown in Figure 3G-3I.
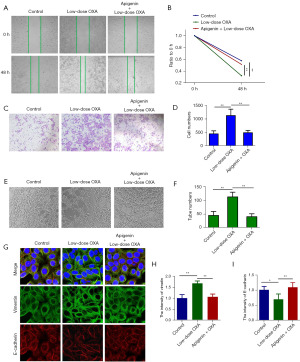
Apigenin inhibited the expression of LINC00857 in OSCC cells
To further elucidate the action mechanism of apigenin in OSCC cells, the expression profiles of long non-coding RNA (lncRNA) in OSCC cells before and after apigenin treatment were analyzed in this study, the data were from previous study (19). There were 1,655 up-regulated lncRNAs and 963 down-regulated lncRNAs identified, as indicated in Figure 4A-4C. Among the apigenin-induced changes in lncRNA expression, four significantly altered lncRNAs were selected, which were LINC00857-001, LINC00630-001, LINC01273-001 and lINC00324-001 (Figure 4D). These alterations in lncRNA expression were validated by qRT-PCR. Notably, LINC00857-001 exhibited a significant decrease upon apigenin treatment (Figure 4E), thus warranting its selection for further investigation and subsequent designation as LINC00857.
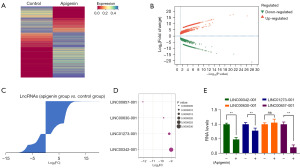
Apigenin inhibited the tumor metastasis promoting effect of low-dose OXA via LINC00857
In this study, LINC00857 was overexpressed by transfecting pcDNA3.1-LINC00857. The results revealed a significant upregulation of LINC00857 expression in cells overexpressing this transcript than the control group, as illustrated in Figure 5A. The previous results demonstrated a significant inhibition of tumor cell invasion induced by low-dose OXA upon treatment with apigenin, whereas the inhibitory effect of apigenin on tumor cell invasion was attenuated in the presence of LINC00857 overexpression, as illustrated in Figure 5B,5C. Additionally, the previous findings demonstrated a substantial suppression of tumor cell angiogenesis induced by low-dose OXA through apigenin, but the inhibitory effect of apigenin on tumor cell angiogenesis was attenuated when LINC00857 was overexpressed, as depicted in Figure 5D,5E.
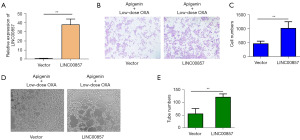
Discussion
The diagnosis and treatment of OSCC have witnessed remarkable advancements in recent years, but its prognosis remains unfavorable. The management of OSCC primarily involves surgical intervention, complemented by radiotherapy or chemotherapy, whereas these treatments have not significantly improved patient survival rates over the past two decades, with the 5-year survival rate remaining consistently low at approximately 60% (20). A contributing factor to this dismal prognosis is delayed diagnosis, and implementing early screening and timely therapeutic interventions can effectively impede the progression of OSCC, leading to an 80% improvement in patient survival (21). A significant increase in metastasis of residual cancer cells has been reported following treatment with OXA. The previous study has demonstrated that platinum-based anticancer drugs at low doses can induce EMT in cancer cells. Chemotherapy-induced EMT is an essential determinant of chemoresistance and tumor metastasis. The results of this study were consistent with the previous researches that conventional doses of OXA significantly inhibited the proliferation of OSCC, but low doses of OXA did not exhibit significant inhibitory effect on OSCC proliferation. More importantly, low doses of OXA significantly promoted the migration, invasion, and angiogenic ability of OSCC, as well as the EMT of tumor cells. At present, platinum-based chemotherapeutic agents remain the first-line drugs in clinical anticancer treatment, and their delivery to tumor cells through the blood flow for efficacy. Solid tumors exhibit a lack of vascularization, rendering it impossible for chemotherapeutic agents to maintain optimal blood concentrations within tumor tissues, while lower concentrations of chemotherapeutic agents not only fail to inhibit the tumor cells, but also stimulate the tumor cells to make the corresponding response, as well as the metastasis of the tumor cells (22). This unfavorable scenario poses challenges in the clinical management of tumors.
Novel therapeutic strategies which safely enhance the sensitivity of chemotherapy offer a promising pathway for the effective treatment of OSCC. Currently, numerous natural small molecules have demonstrated outstanding anticancer activity, with flavonoid molecules being particularly significant. Apigenin, a well-known flavonoid molecule, has been extensively studied and shown to possess potent anticancer properties (23). The findings of this study demonstrated that apigenin exhibited a synergistic effect with OXA and can enhance the inhibitory effect of OXA on OSCC proliferation. Further results demonstrated that apigenin effectively inhibited the migration, invasion, and angiogenic capacity of OSCC induced by low-dose OXA, as well as inhibiting the EMT process of tumor cells. Apigenin is a natural small molecule originated from plants, characterized by its simple structure, easy availability, and minimal toxic side effects, which is suitable for a wide range of clinical applications (24). The combined administration of apigenin and OXA in the treatment of OSCC can not only enhance the anti-tumor effect of OXA, but also alleviate the side effects of OXA at some extent, which is of very important application value.
Further results revealed that apigenin effectively suppressed the expression of the lncRNA LINC00857 in OSCC. LncRNAs have been identified as key regulators of various physiological and pathological processes, including but not limited to growth, apoptosis, differentiation, motility and invasion, signal transduction, DNA damage response, immune modulation. These processes are closely associated with tumorigenesis and make lncRNAs a crucial target for tumor diagnosis, treatment and prognosis (25-28). LINC00857 was initially discovered in lung cancer tissues in 2016, and subsequent investigations have demonstrated its pro-cancer effect and ability to promote EMT in tumor cells (29,30). LINC00857 exerts regulatory control over tumor cells through various mechanisms. Its mechanism of action involves the absorption of miR-1179 and subsequent upregulation of SPAG5 expression, resulting in the inhibition of apoptosis and promotion of proliferation, in vivo growth, and glycolysis in lung cancer cells (31). LINC00857 exerted pro-cancer effects by promoting the EMT process in hepatocellular carcinoma cells through up-regulating the expression of the mesenchymal marker N-cadherin and down-regulating the epithelial marker E-cadherin (30). In addition, LINC00857 promoted ovarian cancer progression through competitive binding with miR-486-5p to regulate Yes1-associated transcriptional regulator (32). Although apigenin has been reported to exert anti-tumor effects through various mechanisms including regulation of lncRNAs, its inhibitory effect on LINC00857 has not been previously elucidated (19). This study further enriched the pharmacological mechanism of natural small molecule apigenin.
The study was still subject to several limitations. First, this study primarily focused on the efficacy of apigenin in combination with OXA, particularly at low concentration of OXA, and its preliminary pharmacological mechanism, without delving into its detailed pharmacological mechanism. Additionally, this study was predominantly conducted in vitro and lacked further validation from in vivo and clinical studies. Moreover, tumor stem cells are closely related to tumor metastasis (33). It has been shown that apigenin is able to exert its inhibitory effect on tumor stem cells by suppressing the Wnt/β-catenin signaling pathway, down-regulating nuclear factor-κB protein expression, and inducing cell cycle arrest through up-regulation of p21 and cyclin-dependent kinases (34,35). The effect and mechanism of apigenin on OSCC tumor stem cells also requires investigation. We intend to refine these findings through future investigations.
Conclusions
In conclusion, this study focused on investigating the combination of apigenin and a low concentration of OXA on OSCC, and revealed that apigenin could inhibit the pro-tumor metastatic effect induced by low concentration of OXA through downregulating the expression of LINC00857. The present study has contributed to enhancing the comprehension of the pharmacological mechanism of apigenin, thereby offering novel insights for its potential clinical implementation in the management of OSCC.
Acknowledgments
Funding: None.
Footnote
Reporting Checklist: The authors have completed the MDAR reporting checklist. Available at https://tcr.amegroups.com/article/view/10.21037/tcr-23-2335/rc
Data Sharing Statement: Available at https://tcr.amegroups.com/article/view/10.21037/tcr-23-2335/dss
Peer Review File: Available at https://tcr.amegroups.com/article/view/10.21037/tcr-23-2335/prf
Conflicts of Interest: All authors have completed the ICMJE uniform disclosure form (available at https://tcr.amegroups.com/article/view/10.21037/tcr-23-2335/coif). The authors have no conflicts of interest to declare.
Ethical Statement: The authors are accountable for all aspects of the work in ensuring that questions related to the accuracy or integrity of any part of the work are appropriately investigated and resolved.
Open Access Statement: This is an Open Access article distributed in accordance with the Creative Commons Attribution-NonCommercial-NoDerivs 4.0 International License (CC BY-NC-ND 4.0), which permits the non-commercial replication and distribution of the article with the strict proviso that no changes or edits are made and the original work is properly cited (including links to both the formal publication through the relevant DOI and the license). See: https://creativecommons.org/licenses/by-nc-nd/4.0/.
References
- Zhang C, Xu C, Gao X, et al. Platinum-based drugs for cancer therapy and anti-tumor strategies. Theranostics 2022;12:2115-32. [Crossref] [PubMed]
- Chen X, Zhou Z, Zhang Z, et al. Puerarin inhibits EMT induced by oxaliplatin via targeting carbonic anhydrase XII. Front Pharmacol 2022;13:969422. [Crossref] [PubMed]
- Karagiannis GS, Pastoriza JM, Wang Y, et al. Neoadjuvant chemotherapy induces breast cancer metastasis through a TMEM-mediated mechanism. Sci Transl Med 2017;9:eaan0026. [Crossref] [PubMed]
- D'Alterio C, Scala S, Sozzi G, et al. Paradoxical effects of chemotherapy on tumor relapse and metastasis promotion. Semin Cancer Biol 2020;60:351-61. [Crossref] [PubMed]
- Wang J, Li D, Zhao B, et al. Small Molecule Compounds of Natural Origin Target Cellular Receptors to Inhibit Cancer Development and Progression. Int J Mol Sci 2022;23:2672. [Crossref] [PubMed]
- Constantinescu T, Lungu CN. Anticancer Activity of Natural and Synthetic Chalcones. Int J Mol Sci 2021;22:11306. [Crossref] [PubMed]
- Imran M, Aslam Gondal T, Atif M, et al. Apigenin as an anticancer agent. Phytother Res 2020;34:1812-28. [Crossref] [PubMed]
- Spicak V, Subrt F. Effect of apigenin on histamine liberation. Cesk Fysiol 1958;7:263-4. [PubMed]
- Birt DF, Walker B, Tibbels MG, et al. Anti-mutagenesis and anti-promotion by apigenin, robinetin and indole-3-carbinol. Carcinogenesis 1986;7:959-63. [Crossref] [PubMed]
- Madunić J, Madunić IV, Gajski G, et al. Apigenin: A dietary flavonoid with diverse anticancer properties. Cancer Lett 2018;413:11-22. [Crossref] [PubMed]
- Huang Y, Hong W, Wei X. The molecular mechanisms and therapeutic strategies of EMT in tumor progression and metastasis. J Hematol Oncol 2022;15:129. [Crossref] [PubMed]
- Jiang J, Li J, Zhou X, et al. Exosomes Regulate the Epithelial-Mesenchymal Transition in Cancer. Front Oncol 2022;12:864980. [Crossref] [PubMed]
- Sommariva M, Gagliano N. E-Cadherin in Pancreatic Ductal Adenocarcinoma: A Multifaceted Actor during EMT. Cells 2020;9:1040. [Crossref] [PubMed]
- Garofalo S, Porzia A, Mainiero F, et al. Environmental stimuli shape microglial plasticity in glioma. Elife 2017;6:e33415. [Crossref] [PubMed]
- Qin Y, Li JY, Kuang JN, et al. Sensitive time-resolved fluoroimmunoassay for the quantitative detection of okadaic acid. Front Mar Sci 2022;9:961751. [Crossref]
- Jayanthi P, Varun BR, Selvaraj J. Epithelial-mesenchymal transition in oral squamous cell carcinoma: An insight into molecular mechanisms and clinical implications. J Oral Maxillofac Pathol 2020;24:189. [Crossref] [PubMed]
- Zhang R, Li S, Lan J, et al. CNTN-1 Upregulation Induced by Low-Dose Cisplatin Promotes Malignant Progression of Lung Adenocarcinoma Cells via Activation of Epithelial-Mesenchymal Transition. Front Genet 2022;13:891665. [Crossref] [PubMed]
- Mottaghi S, Abbaszadeh H. The anticarcinogenic and anticancer effects of the dietary flavonoid, morin: Current status, challenges, and future perspectives. Phytother Res 2021;35:6843-61. [Crossref] [PubMed]
- Shi C, Ma C, Ren C, et al. LINC00629, a KLF10-responsive lncRNA, promotes the anticancer effects of apigenin by decreasing Mcl1 stability in oral squamous cell carcinoma. Aging (Albany NY) 2022;14:9149-66. [Crossref] [PubMed]
- Ling Z, Cheng B, Tao X. Epithelial-to-mesenchymal transition in oral squamous cell carcinoma: Challenges and opportunities. Int J Cancer 2021;148:1548-61. [Crossref] [PubMed]
- Wang Y, Zhang X, Wang S, et al. Identification of Metabolism-Associated Biomarkers for Early and Precise Diagnosis of Oral Squamous Cell Carcinoma. Biomolecules 2022;12:400. [Crossref] [PubMed]
- Pan Z, Zhang H, Dokudovskaya S. The Role of mTORC1 Pathway and Autophagy in Resistance to Platinum-Based Chemotherapeutics. Int J Mol Sci 2023;24:10651. [Crossref] [PubMed]
- Jiang ZB, Wang WJ, Xu C, et al. Luteolin and its derivative apigenin suppress the inducible PD-L1 expression to improve anti-tumor immunity in KRAS-mutant lung cancer. Cancer Lett 2021;515:36-48. [Crossref] [PubMed]
- Singh D, Gupta M, Sarwat M, et al. Apigenin in cancer prevention and therapy: A systematic review and meta-analysis of animal models. Crit Rev Oncol Hematol 2022;176:103751. [Crossref] [PubMed]
- Deng PC, Chen WB, Cai HH, et al. LncRNA SNHG14 potentiates pancreatic cancer progression via modulation of annexin A2 expression by acting as a competing endogenous RNA for miR-613. J Cell Mol Med 2019;23:7222-32. [Crossref] [PubMed]
- Zhang R, Hao S, Yang L, et al. LINC00339 promotes cell proliferation and metastasis in pancreatic cancer via miR-497-5p/IGF1R axis. J BUON 2019;24:729-38. [PubMed]
- Liu B, Wu S, Ma J, et al. lncRNA GAS5 Reverses EMT and Tumor Stem Cell-Mediated Gemcitabine Resistance and Metastasis by Targeting miR-221/SOCS3 in Pancreatic Cancer. Mol Ther Nucleic Acids 2018;13:472-82. [Crossref] [PubMed]
- Zhang X, Hong R, Chen W, et al. The role of long noncoding RNA in major human disease. Bioorg Chem 2019;92:103214. [Crossref] [PubMed]
- Wang L, He Y, Liu W, et al. Non-coding RNA LINC00857 is predictive of poor patient survival and promotes tumor progression via cell cycle regulation in lung cancer. Oncotarget 2016;7:11487-99. [Crossref] [PubMed]
- Xia C, Zhang XY, Liu W, et al. LINC00857 contributes to hepatocellular carcinoma malignancy via enhancing epithelial-mesenchymal transition. J Cell Biochem 2019;120:7970-7. [Crossref] [PubMed]
- Wang L, Cao L, Wen C, et al. LncRNA LINC00857 regulates lung adenocarcinoma progression, apoptosis and glycolysis by targeting miR-1179/SPAG5 axis. Hum Cell 2020;33:195-204. [Crossref] [PubMed]
- Lin X, Feng D, Li P, et al. LncRNA LINC00857 regulates the progression and glycolysis in ovarian cancer by modulating the Hippo signaling pathway. Cancer Med 2020;9:8122-32. [Crossref] [PubMed]
- Sacco A, Battaglia AM, Santamaria G, et al. SOX2 promotes a cancer stem cell-like phenotype and local spreading in oral squamous cell carcinoma. PLoS One 2023;18:e0293475. [Crossref] [PubMed]
- Ghanbari-Movahed M, Shafiee S, Burcher JT, et al. Anticancer Potential of Apigenin and Isovitexin with Focus on Oncogenic Metabolism in Cancer Stem Cells. Metabolites 2023;13:404. [Crossref] [PubMed]
- Yan X, Qi M, Li P, et al. Apigenin in cancer therapy: anti-cancer effects and mechanisms of action. Cell Biosci 2017;7:50. [Crossref] [PubMed]