Systematic proteomics analysis revealed different expression of laminin interaction proteins in breast cancer: lower in luminal subtype and higher in claudin-low subtype
Highlight box
Key findings
• Laminins and their integrin receptors showed lower expression levels in luminal breast cancer with no or lower metastatic ability but showed higher expression levels in claudin-low breast cancer with higher metastatic ability, their higher expression could be related to the low claudin expression in breast cancer.
What is known and what is new?
• Breast cancer is a kind of highly heterogeneous cancer, so it is very important to find targets according to its classification.
• We revealed lower and higher expression levels of laminins and their integrin receptors in luminal and claudin-low breast cancer respectively, which could be potential targets during tumor initiation and development.
What is the implication, and what should change now?
• This study facilitates the identification of new, effective biological markers and targets for different breast cancer types.
Introduction
Breast cancer in women is a major concern across the world, and it is associated with high incidence rate and early onset age (1,2). In addition to BRCA1, BRCA2, and TP53 (3-5), many classical biomarkers exist (6,7); nevertheless, identifying new effective biomarkers and targets remains pivotal. With the emergence of proteomics, systematically investigating protein expression (8,9), modification (10-12), and classification (13,14) has become possible in breast cancer. Although more potential biomarkers and targets can be identified using this approach, such a complex method is bound to generate some inaccurate results. Thus, it is important to discriminate between accurate and inaccurate data. Several cancer-related databases are available, providing comprehensive information pertaining to potential targets. For example, Yuan et al. explored the expression pattern and prognostic value of FUNDC1 in pan-cancer across multiple databases, including Oncomine, PrognoScan, Gene Expression Profiling Interactive Analysis (GEPIA), and Kaplan-Meier Plotter (15). Further, Feng et al. identified significant genes with poor prognosis in ovarian cancer via bioinformatical analysis involving databases such as Gene Expression Omnibus, Database for Annotation, Visualization and Integration Discovery and GEPIA (16). Both proteomics and multi-database analysis can thus lead to the identification of potential cancer targets, but most previous studies involved only one of the two approaches; Thus, combining proteomics with multi-database analysis to identify valid cancer targets is accordingly desirable.
Breast cancer is a type of heterogeneous tumor (17,18), and depending on the expression level of the estrogen receptor (ER), progesterone receptor (PR), human epidermal growth factor receptor 2 (HER2), and Ki-67, it can be classified into luminal A (HER2−, ER+ and PR+), luminal B (HER2−, ER+ or HER2+, ER+), HER2− overexpression (HER2+, ER− and PR−), and triple-negative types (HER2−, ER− and PR−) (19,20). According to histological classification, breast cancer can be generally classified into ductal carcinoma and lobular carcinoma (21). Hence, precise investigation for different breast cancer subtype is important.
Herein we assessed protein expression levels in breast cancer using the isobaric tags for relative and absolute quantification (iTRAQ) method and validated our results with muti-database analysis and biological experiments. We revealed laminins and their receptors as repeated targets for breast cancer, which showed lower expression levels in luminal breast cancer with no or low metastatic ability but showed higher expression levels in claudin-low breast cancer. In addition, we revealed negative correlation between laminins, integrins and claudins. Overall, we observed that laminins and their integrin receptors showed dynamic alterations in expression levels during breast initiation and development, which can facilitate the identification of new, effective biological markers and targets for different breast cancer types. We present this article in accordance with the MDAR reporting checklist (available at https://tcr.amegroups.com/article/view/10.21037/tcr-23-2214/rc).
Methods
Sample preparation
Three pairs of invasive ductal breast carcinoma and para-carcinoma tissues (hereafter referred to as “normal tissues”) were obtained from patients at the Second Affiliated Hospital of Qiqihar Medical University (Qiqihar, China) from January 2018 to December of 2018. All patients were diagnosed with breast cancer but had not undergone chemotherapy nor radiotherapy previously. The samples were stored and transported in liquid nitrogen immediately after the operation. The study was conducted in accordance with the Declaration of Helsinki (as revised in 2013). The experimental protocol was approved by the Qiqihar Medical Ethics Committee (approval No. [2021]25), and informed consent was obtained from all patients.
Cell culture
MCF-10A (SCSP-575), MCF-7 (SCSP-531), ZR-751 (TCHu126), BT-549 (TCHu93), and MDA-MB-231 (TCHu227) cells were purchased from the Cell Bank of Chinese Academy of Science. All cell lines were authenticated by short tandem repeat analysis, and also tested for morphology and mycoplasma contamination. MCF-10A cells were cultured using the MEGM kit (Lonza/Clonetics) in the presence of 100 ng/mL cholera toxin (Sigma, Darmstadt, Germany). MCF7 cells were cultured in MEM (HyClone, Logan, Utah, USA) supplemented with 10% fetal bovine serum (FBS, HyClone). ZR-751 and BT-549 cells were cultured in RPMI 1640 (HyClone) with 10% and 20% FBS, respectively, and in the presence of 2.5 g/L glucose (Sigma-Aldrich; Merck KGaA, Darmstadt, Germany) and 0.11 g/L sodium pyruvate. BT-549 cells also required 0.023 IU/mL insulin (Sigma-Aldrich; Merck KGaA). All cells were cultured at 37 ℃ and in a humidified atmosphere of 5% CO2. MDA-MB-231 cells were cultured in L15 (HyClone) supplemented with 10% FBS at 37 ℃ in a humidified atmosphere without CO2. All the cells were cloned less than 30 generations.
Quantitative protein analysis
The protocols pertaining to protein extraction and digestion, tandem mass tag labeling, peptide separation, MS/MS analysis, database search, and proteomics data validation were as previously reported (22).
GEPIA database analysis
The proteins that were up- (fold change ≥1.5) and downregulated (fold change ≤1/1.5) in our quantification results and in GEPIA database (http://gepia.cancer-pku.cn/) (23) were analyzed using Venn analysis in FunRich v3.1.3 (24). Overlapping proteins with differential expression levels were chosen for further analyses. RGUI v3.4.2 was used to create a heat map of expression levels of these proteins in normal and breast cancer tissues (25). The correlation between laminins, integrins and claudins were also analyzed by GEPIA database.
Analyzing components, protein domains, and pathways
The components, protein domains, and pathways of target proteins were analyzed using FunRich v3.1.3 (24) based on the information retrieved from the UniProt database (https://www.uniprot.org/) (26). Entries with a corrected P value of <0.05 were considered to indicate a statistically significant difference.
Oncomine database analysis
The gene expression levels of laminin interaction proteins in normal and breast cancer tissues were analyzed using the Oncomine-Richardson (27) and Oncomine-Curtis database (28) and those of laminins and their integrin receptors in invasive ductal breast carcinoma and corresponding in situ tissues were analyzed using the Oncomine-Schuetz database (29).
Detection of mRNA expression levels by quantitative real time polymerase chain reaction (qRT-PCR)
Approximately 1×106 cells were collected and treated with 1 mL TRIzol, followed by total RNA isolation. The extracted RNA was then converted into cDNA. mRNA expression was determined using TaKaRa TB Green Premix Ex Taq, as per manufacturer instructions. The reaction mixture comprised 0.25 U DNA polymerase, 150 µg cDNA, 0.2 µM forward and reverse primer each, and 1× PCR buffer; ddH2O was used to adjust the final volume to 10 µL. The cycling conditions were as follows: 95 ℃ for 30 s, 40 cycles of 95 ℃ for 5 s and 60 ℃ for 30 s, and finally 95 ℃ for 30 s. The temperature was then increased from 65 ℃ to 95 ℃ at a rate of 0.5 ℃/s for melting curve analysis. The experiments were replicated three times. Table 1 lists the primers used for qRT-PCR.
Table 1
Primer | Sequence (5'-3') |
---|---|
ITGA1 | |
Forward | CTCTGCTACTGCTTCTTCTG |
Reverse | CTGTTCTCCACTGAGCGTCT |
ITGA6 | |
Forward | TGGGCTATCCTCAAGAGTTCAGTT |
Reverse | GTTATGGGAATGGGACGCAG |
LAMA2 | |
Forward | CTTGAATCCGCTGTCTCCTAT |
Reverse | CACAGATGCCACCAAAAATAGT |
LAMA4 | |
Forward | GCGCTCGGTTCTGCCTCTGT |
Reverse | TCGTCTCAGGCGGGTCTTGC |
LAMB1 | |
Forward | GAGTGCCTGAAGGGGCTTATTTG |
Reverse | TTGTCATCATCGGGGATGGTATTA |
LAMB2 | |
Forward | CTGGGATGATGTAGTCTGTGAG |
Reverse | ACAGGTTCTGAATCCGTGAG |
LAMC1 | |
Forward | CTCTTAATCGCCTGAACACT |
Reverse | TCGTTCTTCATACACTCGC |
Statistical analysis
Enrichment analysis was performed by Bonferroni Method and Benjamini-Hochberg method (BH method). The statistical difference between two groups was performed using student’s t test, the data were replicated through three times. The significance level was set at *P≤0.05, **P≤0.01 and ***P≤0.001. Error bars denoted the standard deviation.
Results
Screening proteins with aberrant expression levels in breast cancer tissues
Overall, 3,792 proteins were identified, of which 3,753 were quantified from human breast cancer and corresponding normal tissues. When the quantification ratio was set at 1.5, 207 proteins were found to be upregulated and 207 proteins were downregulated (22). For further validation, we compared our results with those from the GEPIA database. When the fold-change cut-off was set at ≥1.5 or ≤1/1.5, 44% (92/207) of up- and 58% (120/207) of downregulated proteins were also detected in the database (Figure 1A,1B). The indicated common proteins and their differential expression levels in invasive ductal breast carcinoma and normal tissues are shown in Tables S1,S2 and Figure 1C,1D, they were selected for further analyses.
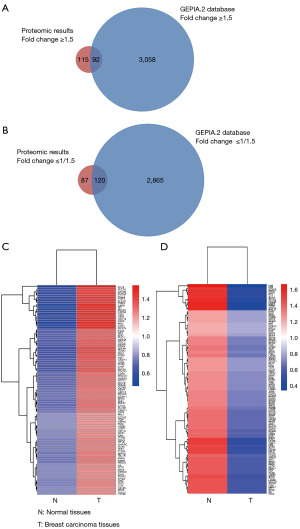
Proteins with aberrant expression levels were enriched in the laminin interaction pathway
Our cellular component analyses revealed that the overlapping proteins were significantly enriched in extracellular components, such as extracellular exosome, extracellular matrix, focal adhesion, extracellular region, and extracellular space (Figure 2A,2B). To investigate the function of these extracellular proteins, we further analyzed them. Protein domain analysis indicated that most of them contained the epidermal growth factor-like domain in leukocyte adhesion molecule (EGF-Lam) domain (Figure 2C), and pathway analysis showed that they were significantly enriched in the laminin interaction pathway (Figure 2D). In addition, all proteins with the EGF-Lam) domain were observed to participate in the laminin interaction pathway (Figure 2E). These findings indicated that proteins involved in laminin interaction may be reliable potential targets.
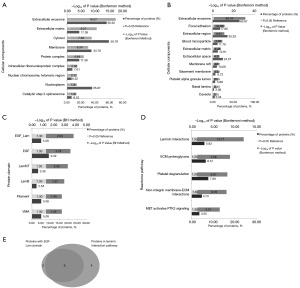
Lower expression levels of laminin interaction-related proteins in breast cancer tissues
Laminin interaction usually involves the combination between heterotrimeric laminin (also referred to laminins) and their receptors on cell membrane and often affects the migration, invasion, and self-renewal of tumor cells (30-34). Heterotrimeric laminin contains one α subunit, one β subunit and one γ subunit, which are encoded by the genes named laminin A, laminin B and laminin C respectively (35). Thus, they are usually named according to their subunit make-up. For example, laminin-211 is just composed of subunit α2, β1 and γ1, which is encoded by gene named laminin subunit α2 (LAMA2), laminin subunit β1 (LAMB1) and laminin subunit γ1 (LAMC1) respectively (36). In our proteomics results, the abnormal expressed proteins in laminin interaction pathway involved laminin subunit β2 (LAMB2), LAMC1, laminin subunit α4 (LAMA4), LAMB1, LAMA2 and their receptors including integrin α7 (ITGA7), integrin α6 (ITGA6), integrin α1 (ITGA1), nidogen-1 (NID1) and basement membrane-specific heparan sulfate proteoglycan core protein (PGBM). In addition, all the above proteins were downregulated in breast cancer tissues when compared with normal tissues (Figure 3A,3B). To validate our results, we analyzed their gene expression levels using the Oncomine database. In Oncomine-Curtis Breast database (27), which includes data more than 2,000 samples, the results showed that genes encoding above 10 proteins were downregulated in ductal breast cancer tissues (Figure 3C). Moreover, Oncomine-Richardson database (28) also suggested that besides ITGA1, all the other nine genes showed significant lower expression level in invasive ductal breast cancer tissues (Figure 3D). Above results revealed lower expression levels of laminin interaction-related proteins in breast cancer tissues.
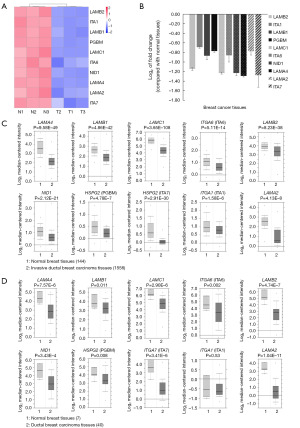
Lower expression levels of laminin interaction-related proteins occurred in luminal but not claudin-low breast cancer cells
To further validate above results acquired from tissue samples, we detected the mRNA expression levels of above laminin interaction-related proteins in different breast cancer cell types. Because laminin subunits and integrin subunits occupied with most of the above ten proteins, thus we chose the proteins above for further validation. Except for LAMA2 and ITGA7, of which the cycle threshold value were more than 35 cycles, we got the data of the other six genes including LAMB2, LAMA4, LAMC1, LAMB1, TIGA1 and ITGA6. The results indicated that when compared with MCF-10A cells, the six genes showed significant lower expression levels in non-metastatic luminal MCF-7 cells; five of them (LAMB2, LAMC1, LAMB1, TIGA1 and ITGA6) showed significant lower expression levels in lower-metastatic luminal ZR-751 cells; only two of them (ITGA1 and ITGA6) showed significant low expression levels in the more invasive claudin-low BT-549 cells (Figure 4A). The results suggested that the lower expression level of laminins and their integrin receptors seems fit to the luminal breast cancer cells with no or lower invasive potential. To validate our hypotheses, we detected the mRNA expression level of above-mentioned genes in MDA-MB-231 cells, which is also claudin-low type and shows strong metastatic ability. As expected, all the six genes showed conversely higher expression levels in MDA-MB-231 cells (Figure 4B). In addition to mRNA expression level, we detected by proteomics techniques the significantly lowered protein expression levels of laminins and their integrin receptors in luminal less metastatic breast cancer cell lines including T47D and ZR-75-1, and their apparently higher expression levels in the more invasive claudin-low MDA-MB-231, MDA-MB-436 and BT-549 cells (Figure 4C,4D). Moreover, due to the Oncomine-Schuetz database (21), the expression level of above proteins in invasive ductal tissues was higher than that of corresponding in situ tissues (Figure 4E). All the above results indicated that the laminins and their integrin receptors may show lower expression during the initiation of breast cancer and achieve higher expression during tumor development.
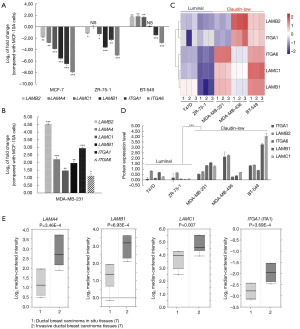
Higher laminins and integrin receptors expression could be correlated with low-claudin expression in breast cancer
Basing on above results, we observed higher expression level of laminins and their integrin receptors in the more invasive breast cancer cells including BT-549, MDA-MB-436 and MDA-MB-231, which belong to triple-negative and claudin-low (claudin-3, claudin-4 and claudin-7 low) breast cancer cells (37-39). To investigate whether laminins and their integrin receptors have something to do with these factors involved in classification, including ER, PR, HER2, claudin-3, claudin-4 and claudin-7, we analyzed the gene expression correlation among them. In our results, neither laminins nor integrins showed the regular relationship with ER, PR and HER2 (data not shown). However, except for the higher P value between genes encoding ITGA6 and claudin-4, all the genes encoding laminin subunits (LAMA2, LAMA4, LAMB1 and LAMC1) and integrin subunits (ITGA1 and ITGA6) showed significant negative correlation with that encoding claudin-3, claudin-4 and claudin-7 (Figure 5A-5F). These results indicate that higher laminins and integrins expression could be correlated with low claudin expression.
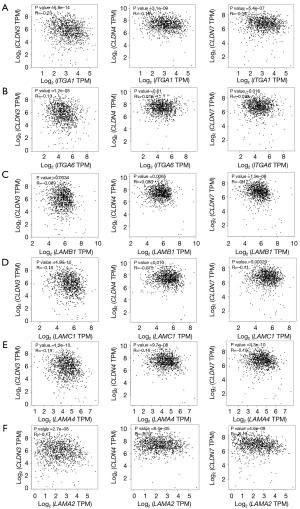
Discussion
At present, proteomics is being widely used for elucidating protein expression, protein modification (22,40) and tumor classification (41,42) for breast cancer. Although proteomics-based methods are well established, their reproducibility is evidently a major challenge for the differences among operators, equipment, and samples. Thus, it is crucial to obtain solid results and to validate them. Here, through our proteomics detection (both for tissue and cell samples), investigation with different databases (GEPIA, Oncomine-Richardson, Oncomine-Curtis and Oncomine-Schuetz), and qRT-PCR detection, we revealed dynamic expression of laminins and their integrin receptors in breast cancer, as well as their correlation with claudins.
As compared with their normal counterpart, breast cancer tissues showed lower expression levels of laminins and their integrin receptors (Figure 3). It has been reported that laminins are necessary for a “soft” extracellular matrix (43) and the loss of the laminin signal can result in a “stiff” extracellular environment and abnormal tissue function, which are hallmarks of solid tumors (44). Thus, the lower expression levels of laminins and their integrin receptors could be one of the reasons for the tumorigenesis of mammary epithelial cells, which is consistent with our results. However, the lower expression level seems to be characteristic for luminal breast cancer cells with no- or lower-invasive ability (such as MCF-7, T47D and ZR-75-1), for we found higher expression level of laminin and their integrins in the more invasive claudin-low breast cancer cells, such as MDA-MB-436, BT-549 and MDA-MB-231 (Figure 4A-4D). In addition, we also found that some laminins and integrins showed higher expression levels in invasive ductal tissues than their corresponding in situ tissues (Figure 4E). That is, the laminins and integrins showed a rebounded expression during cancer progression. As the important components of extracellular matrix, laminins can interact with their receptors and several active intracellular signaling pathway including phosphatidylinositol-3-kinase/protein kinase B (PI3K/AKT), mitogen activated protein kinase/extracellular signal regulated kinase (MAPK/ERK), and Rho GTPases, which involve in the development of some cancer types, including bladder cancer, colorectal cancer, lung cancer, and head and neck squamous carcinomas (45-47). In addition, overexpression of laminins has been reported in breast cancer for facilitating cell metastasis (48). Thus, we speculated that the rebounded expression of laminins and integrins could be one of the main reasons for breast cancer progression, especially for claudin-low expression breast cancer. Furthermore, our continuous validated results suggested that above-mentioned dynamic expression is mainly characteristic for laminins with β1 and γ1 subunits and their integrin receptors with α1 and α6 subunits (Figure 6).
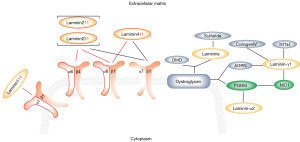
The claudin-low breast cancer is characterized by decreased expression of claudin-3, claudinin-4 and claudin-7, enrichment for markers involved in epithelial-mesenchymal transition and possessing features involved in mammary cancer stem cells (39), and usually shows poor prognosis (37,49). We also found the negative correlation between laminins, integrins and claudin3, claudin4, and claudin7. Moreover, it has been reported that targeting integrin-β1 can sensitize claudin-low cells to mitogen-activated protein kinase kinase (MEK) inhibition (50), indicating its oncogenic role in claudin-low breast cancer. However, there is no reported proof about the correlation and regulation between other laminins, integrins and claudins in breast cancer, which needs our further investigation.
Conclusions
Here, through proteomics detection and experiment validation, we found contrary expression levels of laminins and their integrin receptors according to different breast cancer: they exhibited lower expression levels in luminal breast cancer with no or lower metastatic ability but achieved higher expression levels in claudin-low breast cancer with higher metastatic ability and their higher expression could be related with the low claudin expression.
Acknowledgments
Funding: The study was funded by
Footnote
Reporting Checklist: The authors have completed the MDAR reporting checklist. Available at https://tcr.amegroups.com/article/view/10.21037/tcr-23-2214/rc
Data Sharing Statement: Available at https://tcr.amegroups.com/article/view/10.21037/tcr-23-2214/dss
Peer Review File: Available at https://tcr.amegroups.com/article/view/10.21037/tcr-23-2214/prf
Conflicts of Interest: All authors have completed the ICMJE uniform disclosure form (available at https://tcr.amegroups.com/article/view/10.21037/tcr-23-2214/coif). The authors have no conflicts of interest to declare.
Ethical Statement: The authors are accountable for all aspects of the work in ensuring that questions related to the accuracy or integrity of any part of the work are appropriately investigated and resolved.
Open Access Statement: This is an Open Access article distributed in accordance with the Creative Commons Attribution-NonCommercial-NoDerivs 4.0 International License (CC BY-NC-ND 4.0), which permits the non-commercial replication and distribution of the article with the strict proviso that no changes or edits are made and the original work is properly cited (including links to both the formal publication through the relevant DOI and the license). See: https://creativecommons.org/licenses/by-nc-nd/4.0/.
References
- Giaquinto AN, Sung H, Miller KD, et al. Breast Cancer Statistics, 2022. CA Cancer J Clin 2022;72:524-41. [Crossref] [PubMed]
- Sung H, Ferlay J, Siegel RL, et al. Global Cancer Statistics 2020: GLOBOCAN Estimates of Incidence and Mortality Worldwide for 36 Cancers in 185 Countries. CA Cancer J Clin 2021;71:209-49. [Crossref] [PubMed]
- Duffy MJ, Synnott NC, Crown J. Mutant p53 in breast cancer: potential as a therapeutic target and biomarker. Breast Cancer Res Treat 2018;170:213-9. [Crossref] [PubMed]
- Simon K, Geigl JB, Pristauz G. Genes beyond BRCA1 and BRCA2 for hereditary breast cancer. Wien Med Wochenschr 2010;160:478-82. [Crossref] [PubMed]
- Sharma B, Preet Kaur R, Raut S, et al. BRCA1 mutation spectrum, functions, and therapeutic strategies: The story so far. Curr Probl Cancer 2018;42:189-207. [Crossref] [PubMed]
- Filippini SE, Vega A. Breast cancer genes: beyond BRCA1 and BRCA2. Front Biosci (Landmark Ed) 2013;18:1358-72. [Crossref] [PubMed]
- Breast Cancer Association Consortium. Breast Cancer Risk Genes - Association Analysis in More than 113,000 Women. N Engl J Med 2021;384:428-39. [Crossref] [PubMed]
- Wang Y, Yi K, Chen B, et al. Elucidating the susceptibility to breast cancer: an in-depth proteomic and transcriptomic investigation into novel potential plasma protein biomarkers. Front Mol Biosci 2024;10:1340917. [Crossref] [PubMed]
- Shi X, Liu C, Zheng W, et al. Proteomic Analysis Revealed the Potential Role of MAGE-D2 in the Therapeutic Targeting of Triple-Negative Breast Cancer. Mol Cell Proteomics 2024;23:100703. [Crossref] [PubMed]
- Zhou Y, Lih TM, Yang G, et al. An Integrated Workflow for Global, Glyco-, and Phospho-proteomic Analysis of Tumor Tissues. Anal Chem 2020;92:1842-9. [Crossref] [PubMed]
- Liu C, Liu Y, Chen L, et al. Quantitative proteome and lysine succinylome analyses provide insights into metabolic regulation in breast cancer. Breast Cancer 2019;26:93-105. [Crossref] [PubMed]
- Minic Z, Li Y, Hüttmann N, et al. Lysine Acetylome of Breast Cancer-Derived Small Extracellular Vesicles Reveals Specific Acetylation Patterns for Metabolic Enzymes. Biomedicines 2023;11:1076. [Crossref] [PubMed]
- Tobiasz J, Polanska J. Proteomic Profile Distinguishes New Subpopulations of Breast Cancer Patients with Different Survival Outcomes. Cancers (Basel) 2023;15:4230. [Crossref] [PubMed]
- Hacking S, Chou C, Baykara Y, et al. MMR Deficiency Defines Distinct Molecular Subtype of Breast Cancer with Histone Proteomic Networks. Int J Mol Sci 2023;24:5327. [Crossref] [PubMed]
- Yuan Q, Sun N, Zheng J, et al. Prognostic and Immunological Role of FUN14 Domain Containing 1 in Pan-Cancer: Friend or Foe? Front Oncol 2020;9:1502. [Crossref] [PubMed]
- Feng H, Gu ZY, Li Q, et al. Identification of significant genes with poor prognosis in ovarian cancer via bioinformatical analysis. J Ovarian Res 2019;12:35. [Crossref] [PubMed]
- Zhang N, Ji J, Zhou D, et al. The Interaction of the Senescent and Adjacent Breast Cancer Cells Promotes the Metastasis of Heterogeneous Breast Cancer Cells through Notch Signaling. Int J Mol Sci 2021;22:849. [Crossref] [PubMed]
- Colombié M, Jézéquel P, Rubeaux M, et al. The EPICURE study: a pilot prospective cohort study of heterogeneous and massive data integration in metastatic breast cancer patients. BMC Cancer 2021;21:333. [Crossref] [PubMed]
- Holm J, Eriksson L, Ploner A, et al. Assessment of Breast Cancer Risk Factors Reveals Subtype Heterogeneity. Cancer Res 2017;77:3708-17. [Crossref] [PubMed]
- Goldhirsch A, Winer EP, Coates AS, et al. Personalizing the treatment of women with early breast cancer: highlights of the St Gallen International Expert Consensus on the Primary Therapy of Early Breast Cancer 2013. Ann Oncol 2013;24:2206-23. [Crossref] [PubMed]
- Oliveira NC, Gomig TH, Milioli HH, et al. Comparative proteomic analysis of ductal and lobular invasive breast carcinoma. Genet Mol Res 2016; [Crossref] [PubMed]
- Gao X, Bao H, Liu L, et al. Systematic analysis of lysine acetylome and succinylome reveals the correlation between modification of H2A.X complexes and DNA damage response in breast cancer. Oncol Rep 2020;43:1819-30. [Crossref] [PubMed]
- Tang Z, Li C, Kang B, et al. GEPIA: a web server for cancer and normal gene expression profiling and interactive analyses. Nucleic Acids Res 2017;45:W98-W102. [Crossref] [PubMed]
- Pathan M, Keerthikumar S, Ang CS, et al. FunRich: An open access standalone functional enrichment and interaction network analysis tool. Proteomics 2015;15:2597-601. [Crossref] [PubMed]
- R Core Team. R: A language and environment for statistical computing. R Foundation for Statistical Computing, Vienna 2017.
- UniProt: a worldwide hub of protein knowledge. Nucleic Acids Res 2019;47:D506-15. [Crossref] [PubMed]
- Richardson AL, Wang ZC, De Nicolo A, et al. X chromosomal abnormalities in basal-like human breast cancer. Cancer Cell 2006;9:121-32. [Crossref] [PubMed]
- Curtis C, Shah SP, Chin SF, et al. The genomic and transcriptomic architecture of 2,000 breast tumours reveals novel subgroups. Nature 2012;486:346-52. [Crossref] [PubMed]
- Schuetz CS, Bonin M, Clare SE, et al. Progression-specific genes identified by expression profiling of matched ductal carcinomas in situ and invasive breast tumors, combining laser capture microdissection and oligonucleotide microarray analysis. Cancer Res 2006;66:5278-86. [Crossref] [PubMed]
- Berndt A, Gaßler N, Franz M. Invasion-Associated Reorganization of Laminin 332 in Oral Squamous Cell Carcinomas: The Role of the Laminin γ2 Chain in Tumor Biology, Diagnosis, and Therapy. Cancers (Basel) 2022;14:4903. [Crossref] [PubMed]
- Banerjee S, Lo WC, Majumder P, et al. Multiple roles for basement membrane proteins in cancer progression and EMT. Eur J Cell Biol 2022;101:151220. [Crossref] [PubMed]
- Sevilla CA, Dalecki D, Hocking DC. Extracellular matrix fibronectin stimulates the self-assembly of microtissues on native collagen gels. Tissue Eng Part A 2010;16:3805-19. [Crossref] [PubMed]
- Kusuma N, Denoyer D, Eble JA, et al. Integrin-dependent response to laminin-511 regulates breast tumor cell invasion and metastasis. Int J Cancer 2012;130:555-66. [Crossref] [PubMed]
- Kim BG, Gao MQ, Choi YP, et al. Invasive breast cancer induces laminin-332 upregulation and integrin β4 neoexpression in myofibroblasts to confer an anoikis-resistant phenotype during tissue remodeling. Breast Cancer Res 2012;14:R88. [Crossref] [PubMed]
- Lepucki A, Orlińska K, Mielczarek-Palacz A, et al. The Role of Extracellular Matrix Proteins in Breast Cancer. J Clin Med 2022;11:1250. [Crossref] [PubMed]
- Halder SK, Sapkota A, Milner R. The impact of genetic manipulation of laminin and integrins at the blood-brain barrier. Fluids Barriers CNS 2022;19:50. [Crossref] [PubMed]
- Holliday DL, Speirs V. Choosing the right cell line for breast cancer research. Breast Cancer Res 2011;13:215. [Crossref] [PubMed]
- Neve RM, Chin K, Fridlyand J, et al. A collection of breast cancer cell lines for the study of functionally distinct cancer subtypes. Cancer Cell 2006;10:515-27. [Crossref] [PubMed]
- Prat A, Parker JS, Karginova O, et al. Phenotypic and molecular characterization of the claudin-low intrinsic subtype of breast cancer. Breast Cancer Res 2010;12:R68. [Crossref] [PubMed]
- Johansson HJ, Socciarelli F, Vacanti NM, et al. Breast cancer quantitative proteome and proteogenomic landscape. Nat Commun 2019;10:1600. [Crossref] [PubMed]
- Tyanova S, Albrechtsen R, Kronqvist P, et al. Proteomic maps of breast cancer subtypes. Nat Commun 2016;7:10259. [Crossref] [PubMed]
- Lam SW, Jimenez CR, Boven E. Breast cancer classification by proteomic technologies: current state of knowledge. Cancer Treat Rev 2014;40:129-38. [Crossref] [PubMed]
- Alcaraz J, Xu R, Mori H, et al. Laminin and biomimetic extracellular elasticity enhance functional differentiation in mammary epithelia. EMBO J 2008;27:2829-38. [Crossref] [PubMed]
- Paszek MJ, Zahir N, Johnson KR, et al. Tensional homeostasis and the malignant phenotype. Cancer Cell 2005;8:241-54. [Crossref] [PubMed]
- Qin Y, Shembrey C, Smith J, et al. Laminin 521 enhances self-renewal via STAT3 activation and promotes tumor progression in colorectal cancer. Cancer Lett 2020;476:161-9. [Crossref] [PubMed]
- Hao N, Yang D, Liu T, et al. Laminin-integrin a6b4 interaction activates notch signaling to facilitate bladder cancer development. BMC Cancer 2022;22:558. [Crossref] [PubMed]
- Meireles Da Costa N, Mendes FA, Pontes B, et al. Potential Therapeutic Significance of Laminin in Head and Neck Squamous Carcinomas. Cancers (Basel) 2021;13:1890. [Crossref] [PubMed]
- Qiu X, Tan H, Fu D, et al. Laminin is over expressed in breast cancer and facilitate cancer cell metastasis. J Cancer Res Ther 2018;14:S1170-2. [Crossref] [PubMed]
- Herschkowitz JI, Simin K, Weigman VJ, et al. Identification of conserved gene expression features between murine mammary carcinoma models and human breast tumors. Genome Biol 2007;8:R76. [Crossref] [PubMed]
- Zawistowski JS, Nakamura K, Parker JS, et al. MicroRNA 9-3p targets beta1 integrin to sensitize claudin-low breast cancer cells to MEK inhibition. Mol Cell Biol 2013;33:2260-74. [Crossref] [PubMed]