Mechanisms and therapeutic targets of ErbB family receptors in hepatocellular carcinoma: a narrative review
Introduction
Liver cancer is one of the most common malignant tumors. In 2020, the incidence and mortality rates of liver cancer ranked seventh and third, respectively, among all malignant tumors worldwide (1). Hepatocellular carcinoma (HCC) is the most common histological subtype of primary liver cancer, accounting for approximately 85% to 90% of malignant tumors originating in the liver (2). HCC is highly malignant and invasive, and surgery is a potentially effective means to cure this disease. However, because early clinical symptoms are not obvious, most patients are already in the advanced stage of the disease at the time of diagnosis, and the opportunity for radical surgical resection is lost. Even after surgical resection, approximately 70% of patients experience recurrence within 5 years, and approximately two-thirds of these patients will experience relapse within 2 years due to intrahepatic spread (3).
With the advancement of next-generation sequencing (NGS) technology in recent years, a more in-depth understanding of the molecular mechanisms of tumors have been gained (4). This has facilitated the identification of an increasing array of genetic targets implicated in the initiation and progression of tumors. Consequently, the development of targeted therapeutics informed by these discoveries has significantly influenced the enhancement of prognostic outcomes for patients with cancer. In the realm of potential targets for cancer therapy, ErbB receptor family proteins have emerged as significant players. The aberrant expression of members of the transmembrane tyrosine kinase (TK) receptor family plays a role in the initiation and progression of tumors, especially lung cancer, breast cancer, and gastric cancer (5-7). On activation by their respective ligands, these receptors undergo dimerization and autophosphorylation, triggering a cascade of downstream signaling pathways that regulate cellular processes, such as proliferation, differentiation, migration, and survival. In cancer, the overexpression, mutation, or continuous activation of these receptors leads to the dysregulation of these signaling pathways, contributing to oncogenesis and progression (8). Drugs such as trastuzumab and gefitinib have been shown to target these receptors, demonstrating remarkable efficacy in cancer treatment (9,10). Currently, targeted therapeutic strategies against the ErbB family in HCC have been proposed and have undergone preliminary clinical exploration. This article reviews the research progress on ErbB family proteins in HCC to provide a reference for clinical decision making and to guide future research directions. We present this article in accordance with the Narrative Review reporting checklist (available at https://tcr.amegroups.com/article/view/10.21037/tcr-24-837/rc).
Methods
A comprehensive, narrative review of the literature was conducted to examine the current progress of ErbB family receptors in HCC in both the pre-clinical and clinical arenas. Studies from all periods were reviewed from PubMed/MEDLINE using the keywords “liver cancer”, “hepatocellular carcinoma”, “HCC”, “ErbB”, “EGFR”, “HER1”, “HER2”, “HER3”, and “HER4”.
Articles relevant to the topic of this study were fully reviewed. The search strategy is summarized in Table 1.
Table 1
Items | Specification |
---|---|
Date of search | April 20th, 2024 |
Databases and other sources searched | PubMed/MEDLINE |
Search terms used | Liver cancer/hepatocellular carcinoma/HCC/ErbB/EGFR/HER1/HER2/HER3/HER4 |
Timeframe | All periods |
Inclusion criteria | Original Article; Clinical Trial; Meta-analysis; Review; Systematic Review; written in the English language |
Selection process | All the authors selected the studies together |
HCC, hepatocellular carcinoma; EGFR, epidermal growth factor receptor.
ErbB family members in HCC: expression and mechanism pathways
The ErbB family belongs to the TK receptor family that comprises four members: epidermal growth factor receptor (EGFR; ErbB1 or HER1), ErbB2 (HER2), ErbB3 (HER3), and ErbB4 (HER4). These members have similar structures, mainly consisting of the extracellular domain, transmembrane domain, and intracellular TK domain (Figure 1) (11,12). With the exception of ErbB2, which has no known endogenous ligand, the other receptors promote autophosphorylation and downstream signaling cascades by binding to their respective ligands to form homodimers or heterodimers. However, ErbB2 forms heterodimers with the other three receptors (8). These distinct activated signaling pathways, such as the phosphoinositide 3-kinase (PI3K)/protein kinase B (Akt) signaling pathway, the Janus kinase (JAK)/signal transducers and activators of transcription (STAT) signaling pathway, and the mitogen-activated protein kinase (MAPK) signaling pathway, govern various cellular functions, including proliferation, cell cycle regulation, and migration (13,14). In living organisms, intercellular communication generates spatially heterogeneous gene expression patterns that are crucial for the development of tissues and organs. Cells can engage in autocrine signaling, where ligands produced by the cell directly activate receptors on the same cell, allowing for a rapid response to its own signals. Another mechanism is paracrine signaling, in which ligands are released into the extracellular environment, diffuse to neighboring cells, and interact with their receptors, facilitating communication between different cell types. In the endocrine mechanism, ERBB ligands secreted by cells are transported through the bloodstream or other body fluids to distant cells, activating ERBB receptors on those cells. This process is similar to hormone action, transmitting signals over long distances within the body to regulate the functions of various tissues and organs (15). Elevated levels of ErbB ligands have been reported in several cancers, including ovarian, gastric, and breast cancer, and are suspected to promote tumor invasiveness (16,17). For instance, amphiregulin (AREG) is overexpressed in various cancers, including HCC, and its role in tumor development and prognosis is well established (18-20). The trafficking, processing, and release of ERBB family ligands determine the scope, intensity, and duration of downstream signal transduction. Endocrine, paracrine, and autocrine mechanisms are recognized modes of ERBB ligand action (21). A critical step in these mechanisms is the ectodomain shedding of membrane-bound EGFR ligands. Protease-mediated cleavage releases soluble EGFR ligands into the extracellular environment, enabling them to participate in autocrine, paracrine, and endocrine signaling. This shedding process not only generates soluble ligands but also leaves a free cytoplasmic tail that can interact with other cytoplasmic proteins to regulate gene expression (22). For example, a gene replacement study on HBEGF has shown that mice expressing non-cleavable HBEGF develop severe heart failure and enlarged heart valves, resembling the phenotype of full HBEGF knockout (23). In contrast, mice expressing constitutively soluble HBEGF exhibit severe hyperplasia in the skin and heart (23). G-protein-coupled receptors (GPCRs) can utilize a metalloprotease-dependent process to transactivate EGFR, generating potent mitogenic signals. This signaling mechanism, known as the “triple membrane-passing signal”, is widely observed in various cancers and other diseases, modulating cell proliferation, apoptosis, and migration (24). ERBB family ligands can activate EGFR and its downstream signaling pathways through autocrine, paracrine, and endocrine mechanisms, thereby regulating cell proliferation, migration, and survival in different ways.
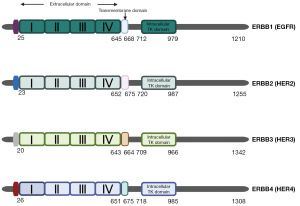
The development of liver cancer is a complex, multistep process characterized by alterations in several signaling cascades, culminating in the emergence of heterogeneous neoplasms. Research indicates that the ErbB signaling pathway is one of the most commonly mutated pathways in HCC (25). Therefore, exploring the ErbB signaling pathway in HCC could provide greater insights into the biology and progression of this disease, and potential therapeutic strategies.
EGFR in HCC
EGFR, also known as HER1 or ErbB1, belongs to the receptor TK ErbB family and plays a carcinogenic role in various tumors (26). It is known that seven ligands [i.e., epidermal growth factor (EGF), AREG, epigen, epiregulin, betacellulin, heparin-binding EGF-like growth factor, and transforming growth factor (TGF)-alpha] can bind to the extracellular domain of EGFR, inducing receptor dimerization and TK activation, thus triggering downstream signaling pathways (Figure 2). This pathway is referred to as the canonical ligand-dependent EGFR signaling pathway, which transduces various signaling pathways, including the renin-angiotensin system (RAS)/rapidly accelerated fibrosarcoma (RAF)/MAP kinase-ERK kinase (MEK)/extracellular signal-regulated kinase (ERK) pathway, PI3K/Akt/mammalian target of rapamycin (mTOR) pathway, and phospholipase C (PLC)/protein kinase C (PKC) pathway (27). As a crucial hub for external growth and survival signals, the EGFR system primarily regulates biological processes, such as cell proliferation, apoptosis, differentiation, and migration (28).
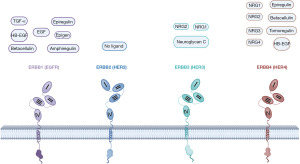
As a member of the ErbB family, EGFR frequently undergoes mutation and/or overexpression in various types of human cancers. EGFR can be phosphorylated at multiple sites, and in the majority of HCCs, the tyrosine residue at position 845 of EGFR is phosphorylated, and its phosphorylation sites are related to the subsequently activated signaling pathways (29). Mutations in EGFR have been shown to promote tumor development both in vitro and in animal models (30,31). In 2003, Fitch et al. (32) identified EGFR (Leu863Gln and Dsk5) as a new class of pigmentation mutation (Dsk) phenotype that results in elevated basal EGFR kinase activity and signaling levels, leading to spontaneous primary HCC in mice older than 8 months (33).
EGFR is overexpressed in 68–96% of HCC cases, while mutations occur in only 1% of cases (34-36). A study has shown that EGFR expression is closely related to the degree of differentiation, proliferative activity, incidence of intrahepatic metastasis, and prognosis of HCC patients, with high EGFR expression possibly indicating poor prognosis in HCC patients (37). Song et al. (35) discovered that the EGFR/mesenchymal-epithelial transition factor (MET)-induced RAS/MAPK pathway stabilized HCC cells and participated in distant metastasis through circulating tumor cells, while also partially inhibiting the killing ability of immune cells. High phosphorylation levels of EGFR/MET, such as platelet-derived growth factor receptor βand fibroblast growth factor receptor 1, also preserve the elevated phosphorylation status of clinical targets in HCC treatment, which may result in the ineffectiveness of targeted therapies and facilitate extrahepatic metastasis. Ji et al. (38) discovered that EGF-EGFR upregulates the expression of T-box (TBX) 19 via the ERK/ nuclear factor kappa-light-chain-enhancer of activated B cells (NF-κB) pathway, and TBX19 further upregulates the expression of EGFR and Rac family small GTPase 1 (RAC1), forming an EGF-TBX19-EGFR-positive feedback loop that promotes liver cancer metastasis.
Abnormal DNA methylation is closely linked to the occurrence, development, and malignant transformation of tumors, and EGFR also plays a role in this process. For example, the hypermethylation of empty spiracle homeobox 1 (EMX1), which binds to the EGFR promoter, promotes EGFR transcription and activates the EGFR-ERK signaling pathway, triggering the onset and metastasis of HCC (39). Su et al. (40) reported that sublethal heat treatment boosts EGFR N6-methyladenosine (m6A) modification and its binding with YTH N6-methyladenosine RNA binding protein 1, enhancing EGFR messenger RNA translation and elevating EGFR levels, which in turn fosters malignancy in HCC cells. Concurrently, Quiescin sulfhydryl oxidase 1 (QSOX1) inhibits nuclear factor erythroid 2-related factor 2 (NRF2) activation by promoting EGFR ubiquitination and endosomal trafficking, increasing oxidative stress sensitivity in HCC cells and decreasing their viability (41). The overexpression of 5’-nucleotidase domain containing 2 has been shown to fuel liver cancer cell proliferation and tumor growth by directly engaging with EGFR and augmenting its expression through the modulation of EGFR ubiquitination, thereby stimulating downstream signal transduction (42). These findings highlight the pivotal role of EGFR in the survival and progression of HCC.
EGFR-mediated activation of the PI3K/AKT/mTOR pathway regulates HCC cell survival, proliferation, and the cell cycle, illustrating its central function in cancer cell biology (43). VersicanV1 serves as an upstream activator of EGFR, activating this signaling pathway to enhance the Warburg effect in liver cancer cells, thereby promoting the malignant phenotype of liver cancer cells (44). The PI3K/AKT signaling pathway not only conveys external proliferation signals from receptors on the cell membrane to the nucleus, enhancing cell growth and division, but also elevates the expression of glucose transport proteins on the cell surface, affecting glucose uptake and metabolism, thereby providing the energy and material foundation for rapid cancer cell proliferation (45). In addition, EGFR has been demonstrated to facilitate aerobic glycolysis through a variety of kinase-dependent and kinase-independent mechanisms, profoundly affecting cancer metabolism and progression. Moreover, a study revealed that in cirrhotic mouse models, an interaction between the RAS and the EGFR signaling pathway activates the MAPK pathway, facilitating the transition from liver cirrhosis to HCC.
The angiotensin-converting enzyme inhibitor captopril has been found to effectively mitigate liver fibrosis and decrease the incidence of HCC (46). Additionally, polymorphisms in the EGF gene lead to increased human EGF expression, which exacerbates liver cirrhosis and hastens progression to HCC by activating additional EGFR signaling pathways (47,48). The internalization of EGFR is a critical step in the cellular signaling process, and a study has shown that the ubiquitination of the ultraviolet (UV) radiation resistance-associated gene enhances the lysosomal degradation of EGFR, thereby inhibiting the initiation and development of HCC (49). Sorting nexin 5 facilitates the growth and spread of liver cancer cells through the suppression of EGFR endocytosis and degradation, which in turn triggers the activation of the ERK1/2 signaling pathway (50). The EGFR-related signaling pathway effectively substantiates the concept of “oncogene addiction”, whereby cancer cells rely on the sustained specific activation or overexpression of oncogenes to maintain their proliferation, invasion, and other phenotypes, thereby accelerating cancer progression (51). In addition, cholestatic bile acids activate Nicotinamide Adenine Dinucleotide Phosphate Hydrogen (NADPH) oxidase, triggering YES-mediated EGFR phosphorylation through a ligand-independent pathway, which in turn activates hepatic stellate cells, thereby promoting the development of liver fibrosis and cirrhosis and increasing the incidence of HCC (52,53). EGFR and ephrin receptor A2 act as cofactors to facilitate hepatitis C virus (HCV) infection in the host, indirectly inducing liver cirrhosis and HCC (54). In summary, EGFR plays a crucial role in the development of HCC, and its underlying mechanisms are worthy of further investigation.
ErbB2 in HCC
ErbB2 (HER2) is a membrane receptor protein that belongs to the EGFR family and regulates cell growth, differentiation, and survival. In a variety of malignancies, including breast cancer, gastric cancer, colorectal cancer, and other cancers, the HER2 gene is overexpressed, and has been identified as an oncogenic driver that is closely associated with poor patient prognosis (55-57). ErbB2 differs structurally from other family members and lacks known natural ligands. Its extracellular domain maintains constitutive activity, allowing spontaneous homodimerization (ErbB2 binding to ErbB2) in cells overexpressing ErbB2 (Figure 2). Additionally, ErbB2 tends to form heterodimers with other members of the EGFR family, exhibiting stronger signaling capacity than homodimerization, thus playing a significant role in cellular signaling (58). HER2 typically primarily activates downstream MAPK and PI3K/AKT pathways, regulating tumor cell proliferation and suppressing apoptosis (59).
The overexpression of HER2 typically manifests in tumors through gene amplification. HER2 overexpression has been observed in approximately 20–40% of patients with HCC (34,60). In early studies, it was generally believed that HER2 played a limited role in the occurrence and progression of HCC (61,62). However, with increasing research, new evidence has revealed the complex and diverse role of HER2 in the pathogenesis of HCC. These studies suggest that the overexpression of HER2 is closely associated with poor clinical prognosis in HCC patients, and HER2 may influence the development of HCC through multiple pathways, including promoting the proliferation, invasion, and migration of tumor cells. A bioinformatics analysis of differentially expressed genes in HCC demonstrated that HER2 is a key gene closely associated with the occurrence and progression of HCC (63). Therefore, the role and clinical significance of HER2 in the pathophysiology of HCC are being re-evaluated and further studied.
Additionally, HER2 is considered a factor that promotes tumor metastasis. It is involved in multiple biological processes related to tumor invasiveness and metastatic capability, including matrix degradation, increased proteolytic activity, enhanced vascular permeability, and the growth, proliferation, migration, and differentiation of endothelial cells. These processes not only contribute to the growth of tumors at the primary site but also facilitate the spread of cancer cells through the vascular and lymphatic systems, leading to the formation of metastatic lesions in other parts of the body. A recent study indicated that the overexpression or activation of HER2 leads to the upregulation of β-catenin levels, resulting in increased accumulation of β-catenin in the nucleus (60). This process concurrently disrupts the TGF-β signaling pathway by inhibiting the activation of the small mothers against decapentaplegic 2/3 (SMAD2/3) complex. Such modulation of cellular responses diminishes the growth-inhibitory effects of the TGF-β pathway, thereby facilitating tumor proliferation, invasion, and metastasis (60).
Epithelial-mesenchymal transition (EMT) refers to the primary cellular process by which epithelial cells lose their cell polarity and cell-to-cell adhesion and acquire mesenchymal cell traits, thereby increasing their motility and invasiveness. EMT plays a significant role in tumor metastasis and progression, and HER2 participates in HCC cell EMT through the MAPK/ERK pathway, thereby promoting tumor invasion and migration (64). Moreover, it has been reported that the HER2 protein is upregulated in patients with HCC with a background of hepatitis B virus (HBV) infection, and such patients typically exhibit a poorer prognosis. The HBV-encoded X protein (HBx) is associated with the upregulation of the HER2 protein, leading to increased HER2 expression. Subsequently, this elevated expression may activate Akt activity, thus facilitating IκB kinase-α (IKK-α) nuclear translocation and ultimately enhancing the migratory capability of liver cancer cells (65).
With the in-depth exploration of the multifaceted roles of HER2 in HCC, significant strides can be made toward its clinical application, offering new hope to patients through targeted treatment options. Concurrently, the ongoing debate surrounding whether HER2 can serve as a prognostic factor in HCC needs further exploration to optimize treatment strategies for HCC patients.
ErbB3 and ErbB4 in HCC
The ErbB3 (or HER3) gene is located on the long arm of human chromosome 12 (12q13), and it encodes a protein with a molecular weight of either 160 or 180 kDa (66). Previously, ErbB3 was thought to lack intrinsic kinase activity, but a recent study has revealed that it possesses weak kinase activity (33). It interacts with other members of the ErbB family to form heterodimers, thereby exerting its function (67). Neuregulin 1 (NRG1) and NRG2 act as ligands that bind to ErbB3, causing a conformational change in ErbB3, thereby facilitating the dimerization, phosphorylation, and activation of signaling pathways (68). There are nine classical ligands capable of activating ErbB4, among which NRG3 and NRG4 are unique (69,70).
ErbB3 and ErbB4 do not play as prominent roles in the TK family as EGFR and HER2; however, due to the overexpression of ErbB3 in certain cancers, it still garners significant interest as a potential therapeutic target. A study on a biocomputational model of the ErbB signaling network revealed that ErbB3 is a critical node in the activation of the ligand-induced ErbB receptor-PI3K axis, and plays a crucial role in the onset and development of cancer (71). Recent studies have also confirmed the critical role of ErbB3 in cellular transformation and cancer. Genetic mutations and methylations of ErbB3 exert a broad regulatory effect on the tumor microenvironment (TME), promoting the onset of cancer (72-74). ErbB3 has six YXXM motifs that bind to the p85 regulatory subunit of PI3K, activating it. The p85 regulatory subunit of PI3K (PI3KR) is one of the most thoroughly studied targets of ErbB3. PI3KR has been shown to promote cell growth and metastasis in a variety of tumors, including HCC. In addition, ErbB3 is significantly overexpressed in 70.4% of HCC patients and is closely associated with microvascular invasion, early recurrence and poor prognosis in HCC patients, while ErbB4 is not significantly expressed (75). The primary mechanism involves the autocrine activation of ErbB3 in HCC through the NRG1/ErbB3 autocrine loop, which in turn induces the PI3K/AKT and MAPK/ERK signaling pathways, playing a key role in the regulation of invasion and migration in HCC. Additionally, the study revealed a secreted isoform of ErbB3 in the serum of HCC patients, and the expression levels of this isoform significantly affected portal vein invasion and metastasis (75). The results of this study indicate that as a potential therapeutic target or co-target, the ErbB3 signaling pathway may be more effective in preventing and treating HCC recurrence and metastasis than in treating advanced HCC. ErbB3 has been reported to be crucial for the formation of HCC tumors and cellular proliferation (33). Ni et al. (76) explored the role of epithelial V-like antigen 1 (EVA1) in HCC and found that EVA1 promoted the growth and migration of HCC cells in vitro through the ErbB3-PI3K-AKT signaling pathway and induced metastasis in vivo.
ErbB4 (or HER4) is a unique member of the ErbB family with growth-inhibitory properties and plays a dual role in cancer initiation and progression. Its constitutively dimerization mutant, HER4 Q646C, has been shown to inhibit pancreatic tumors, breast cancer, and prostate cancer cell lines (77-79). Meanwhile, research also suggests that HER4 may reduce tumor formation and progression by enhancing differentiation and inhibiting growth through the expression of splice variants (80). HER4 may play a protective role in cancer by attenuating the proliferation and oncogenic effects of heterodimerization between other ErbB receptors (81). Recently, Liu et al. (82) used carbon tetrachloride and diethylnitrosamine to induce models of liver inflammation and tumors, respectively, in mice. These findings suggest that the absence of ErbB4 contributes to the development of HCC, and ErbB4 deficiency leads to poor differentiation and a poor prognosis in HCC patients. Reduced levels of TP53INP1, which are possibly linked to diminished ErbB4 expression or its absence, indirectly reduce the stability and apoptotic or cell cycle arrest functions of p53, thereby fostering the proliferation, survival, and advancement of HCC tumor cells. Similarly, additional research has reported that the overexpression of ErbB4 inhibits the proliferation and promotes the apoptosis of HCC cells by reducing cell viability and clonogenicity and inducing cell cycle arrest (83). In the context of immunity, the activation of ErbB2 and ErbB4 receptors mediates the induction of interleukin-10 (IL-10) production by immune cells in response to NRG1, thereby exerting inhibitory effects on tumor cells (84). Therefore, ErbB4 may play a protective role in the occurrence and development of HCC, and its specific mechanisms warrant further exploration.
Membrane receptor trafficking and signaling are frequently altered in cancer. Clathrin is an essential structural protein in eukaryotic cells, predominantly involved in endocytosis and vesicle trafficking processes. It forms clathrin-coated vesicles to facilitate the intracellular and extracellular transport of various substances (85). Clathrin-mediated endocytosis (CME) is the primary pathway for EGFR internalization (86,87). ERBB endocytosis may also involve clathrin-independent endocytosis (CIE) pathways, depending on the ligand bound to ERBB and its concentration (88). For instance, when EGF binds to EGFR, it induces EGFR dimerization and autophosphorylation. This activated form of EGFR dimer undergoes rapid endocytosis, and the internalized EGF-EGFR complex is transported to early endosomes. CME preferentially sorts EGFR to the recycling pathway, where some internalized EGFR is dephosphorylated and recycled back to the plasma membrane, preparing for another round of signaling. A small portion of EGFR, however, is directed to the lysosomal degradation pathway, where it is degraded, thereby regulating the termination of signaling. The CME mechanism of EGF-EGFR, mediated by clathrin and associated adaptor proteins, helps maintain and regulate EGFR signaling and recycling, which is crucial for cell proliferation and tumor development (89,90). Liu et al. (91) studied the effect of Clathrin on ErbB receptor signaling in HCC cell lines and found that clathrin knockdown reduced ErbB receptor phosphorylation and, in response to ligand AR, significantly reduced AKT phosphorylation. Meanwhile, clathrin knockdown increased STAT3 phosphorylation regardless of ligand stimulation. A recent study found that during ligand stimulation, atypical CME of ligand-free EGFR monomers occurs parallel to the typical CME of ligand-bound EGFR dimers. The atypical CME of ligand-free EGFR monomers operates via a p38-dependent pathway under stress conditions, requiring phosphorylation at specific sites (Ser-1015, Thr-1017, and Ser-1018) on EGFR, ultimately leading to EGFR internalization and recycling (92). The regulation of ErbB signaling by clathrin in HCC is cell-specific and ligand-specific, further investigation into the relationship between grid and ErbB signaling proteins will contribute to enhancing targeted treatment strategies for the ErbB receptor family.
The ERBB signaling pathway promotes tumor growth by influencing antitumor immune responses within the TME, a complex ecosystem that provides conditions for tumor cell survival and proliferation. T lymphocytes (such as CD8 + T cells and Tregs), myeloid cells (such as macrophages and myeloid-derived suppressor cells), cytokines (such as IL-6 and TNF-α), and exosomes collectively construct a complex immunoregulatory network within the TME, affecting tumor immune evasion and progression (93). In the early stages of tumor development, T lymphocytes recognize and kill tumor cells through immune surveillance. However, as the tumor progresses, tumor cells employ various mechanisms to evade immune surveillance, resulting in altered T lymphocyte function and even immune suppression (94). Increasing evidence indicates that genomic alterations acquired during tumor development can modulate the TME, aiding tumor cells in evading immune surveillance (95). A study has found that ERBB signaling is one of the most extensively mutated pathways mediating antitumor immunity, with elevated levels of ERBB mutations correlating with poor prognosis in cancer patients (96). Mutations in the ERBB signaling pathway lead to complex interactions among different cell types (such as epithelial cells, macrophages, and T cells), creating a microenvironment conducive to tumor growth. In tumors with ERBB pathway mutations, the secretion of midkine (MDK) is significantly increased. MDK interacts with its receptor LRP1, promoting the differentiation of tumor-infiltrating macrophages into immunosuppressive M2 macrophages. These M2 macrophages inhibit antitumor immune responses and promote tumor growth and progression. Additionally, the interaction between macrophage-secreted CXCL10 and its receptor CXCR3 on regulatory T cells (Tregs) activates Tregs, further suppressing antitumor immune responses and leading to tumor progression (97). Wang et al. (98) discovered that AREG regulates Treg suppressive function and induces immune tolerance and evasion through the EGFR/GSK-3/Foxp3 axis. CD8 + T cells play a major antitumor role in the TME by releasing various effector molecules to attack and destroy tumor cells. However, mutations in the ERBB signaling pathway can significantly reduce the number of CD8+ tumor-infiltrating lymphocytes (TILs), leading to immunosuppression (99). Moreover, MHC molecules are crucial for tumor antigen presentation. MHC I activates CD8+ T cells to specifically kill tumor cells, while MHC II enhances cytotoxicity through cytokine secretion and participates in the body’s positive feedback regulation against tumors (100). EGFR mutations can decrease MHC levels, contributing to immune evasion (101).
Future studies may further elucidate the roles of these receptors in HCC, thereby providing support for the development of new treatment strategies. In summary, the ErbB pathway is one of the most prominently mutated pathways in HCC and plays a crucial role in the development and progression of HCC (Figure 3). These findings serve as a crucial foundation for assessing the effectiveness of ErbB pathway-targeted therapies in the clinic or those currently under development for patients with HCC.
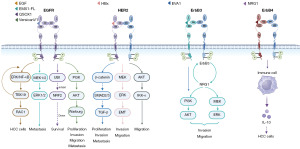
ErbB inhibitors: clinical applications and exploration
With continuous advancements in science and technology, precision medicine has increasingly become a major trend in the field of cancer treatment. This approach largely relies on high-throughput NGS technologies and systematic analyses to achieve highly precise treatments tailored to the individual characteristics of cancers (102). The overexpression and/or functional alteration of oncogenes are among the primary drivers of cancer cell proliferation and tumor development. Consequently, molecular targeted therapies against these key genes have been extensively researched and applied in recent years, proving to be an effective strategy for cancer treatment (103). In particular, as critical signaling molecules in tumor progression, members of the ErbB family have emerged as focal points in targeted therapy research (Table 2). Over the past few decades, small-molecule drugs targeting the ErbB family have been developed and successfully applied to various types of cancer, such as lung, gastric, colorectal, and pancreatic cancers, and have demonstrated significant efficacy (122-125). Currently, with a deeper understanding of the pathogenesis and pathological heterogeneity of cancers, such as HCC, a new era has been initiated for the use of novel targeted treatment strategies, including ErbB small-molecule inhibitors.
Table 2
Study | Drugs | Phase | Targets | Design | Sample size | Results |
---|---|---|---|---|---|---|
Philip 2005, (104) | Erlotinib | II | EGFR | Single-arm | 38 | Positive |
Thomas 2007, (105) | Erlotinib | II | EGFR | Single-arm | 40 | Positive |
Thomas 2009, (106) | Erlotinib + bevacizumab | II | EGFR; VEGFR | Single-arm | 40 | Positive |
Kaseb 2012, (107) | Erlotinib + bevacizumab | II | EGFR; VEGFR | Single-arm | 59 | Positive |
Philip 2012, (108) | Erlotinib + bevacizumab | II | EGFR; VEGFR | Single-arm | 27 | Negative |
Yau 2012, (109) | Erlotinib + bevacizumab | II | EGFR; VEGFR | Single-arm | 10 | Negative |
Chiorean 2012, (110) | Erlotinib + bevacizumab | II | EGFR | Single-arm | 14 | Negative |
Govindarajan 2013, (111) | Erlotinib + bevacizumab | II | EGFR; VEGFR | Single-arm | 21 | Negative |
Hsu 2013, (112) | Erlotinib + bevacizumab | II | EGFR; VEGFR | Single-arm | 51 | Positive |
Zhu 2015, (113) | Erlotinib + sorafenib | III | EGFR; RAF; VEGFR; PDGFR; KIT | RCT | 720 | Negative |
Placebo + sorafenib | ||||||
Kaseb 2016, (114) | Erlotinib + bevacizumab | II | EGFR; VEGFR | Single-arm | 44 | Positive |
Patt 2017, (115) | Erlotinib + GEMOX | II | EGFR | Single-arm | 26 | Negative |
Thomas 2018, (116) | Erlotinib + bevacizumab | II | EGFR; RAF; VEGFR; PDGFR; KIT | Single-arm | 90 | Negative |
Sorafenib | ||||||
Zhu 2007, (117) | Cetuximab | II | EGFR | Single-arm | 30 | Negative |
Asnacios 2008, (118) | Cetuximab + GEMOX | II | EGFR | Single-arm | 45 | Positive |
Weekes 2019, (119) | Cetuximab + regorafenib | Ib | EGFR; RAF; VEGFR; PDGFR; KIT | Single-arm | 42 | Positive |
Ramanathan 2009, (120) | Lapatinib | II | EGFR; HER2 | Single-arm | 40 | Negative |
Bekaii-Saab 2009, (121) | Lapatinib | II | EGFR; HER2 | Single-arm | 26 | Negative |
EGFR, epidermal growth factor receptor; VEGFR, vascular endothelial growth factor receptor; RAF, rapidly accelerated fibrosarcoma; PDGFR, platelet-derived growth factor receptor; KIT, receptor tyrosine kinase; HER2, human epidermal growth factor receptor 2; RCT, randomized controlled trial.
Targeting EGFR for HCC treatment
Gefitinib and erlotinib, first-generation EGFR tyrosine kinase inhibitors (TKIs), were approved by the Food and Drug Administration (FDA) for cancer treatment in 2003 and 2004, respectively, and demonstrated significant therapeutic efficacy, particularly in the treatment of advanced non-small cell lung cancer (NSCLC) in the realm of oncology (126,127). However, the therapeutic efficacy of EGFR-TKIs for HCC still requires further exploration.
In a phase II clinical trial involving 38 HCC patients, erlotinib was effective in controlling disease progression in only a minority of patients who were EGFR-positive (104). A subsequent phase II clinical trial also revealed that monotherapy with erlotinib exhibited moderate disease control benefits in approximately half of the 40 patients with advanced HCC (105). In a 16-week phase II single-arm clinical trial of advanced stage HCC patients treated with bevacizumab [a humanized monoclonal antibody against vascular endothelial growth factor (VEGF) α] and erlotinib, the primary endpoint of progression-free survival (PFS) was 62.5%, with an overall clinical response rate of 25%, demonstrating significant clinical efficacy (106). Similar results were also achieved in another 16-week phase II clinical trial (107).
Three phase II studies investigating the efficacy of combining bevacizumab with erlotinib for advanced HCC treatment yielded disappointing results, but this regimen can be used as an alternative for some patients who are intolerant to sorafenib treatment (108,109,111). The potential reasons for the failure of the experiments include the relatively small number of the patients recruited, coupled with the fact that all the participants were recruited from a single institution. Such a scenario significantly limits the extrapolation and accuracy of the findings. Further, the lack of any significant therapeutic response among patients during the administration of sorafenib suggests that the participants might possess intrinsic resistance to antiangiogenic strategies. This intrinsic resistance may have contributed to the unsuccessful outcomes observed in the study.
A subsequent multicenter phase II study conducted in Asian countries revealed that the combination of bevacizumab and erlotinib demonstrated certain efficacy and tolerability in Asian patients (112). Another study evaluating the efficacy and safety of erlotinib combined with docetaxel in patients with refractory hepatobiliary cancer demonstrated preliminary safety and tolerability but no significant difference in efficacy compared to erlotinib monotherapy (110). In a randomized, multicenter, double-blind, placebo-controlled phase III clinical trial (SEARCH III), 720 patients were randomly assigned to receive either a combination treatment of sorafenib and erlotinib or sorafenib with a placebo. The results indicated that the combination of sorafenib and erlotinib did not improve the survival of patients with advanced HCC, and there was a greater incidence of adverse events in the early stages of treatment in the sorafenib and erlotinib groups (113).
Subsequently, Kaseb et al. (114) evaluated the efficacy and tolerability of bevacizumab and erlotinib as second-line treatments in 44 patients with sorafenib-resistant HCC. The results showed a median overall survival (OS) of 9.9 months, with a 16-week PFS rate of 43%, demonstrating encouraging efficacy outcomes. Patt et al.’s phase II clinical study (115) did not demonstrate a significant survival benefit among HCC patients receiving combination therapy of oxaliplatin, gemcitabine, and erlotinib. Recent results from a multicenter phase II clinical trial indicated that the combination of bevacizumab and erlotinib, compared to sorafenib alone, did not affect the OS of patients with advanced HCC, but the combination therapy resulted in a longer median event-free survival and demonstrated superior safety and tolerability profiles (116).
Rodent models of chronic liver disease and human cirrhosis exhibit similar liver fibrosis/cirrhosis-associated molecular pathway patterns. Consistent with the findings of studies of humans, Fuchs et al. (128) evaluated the therapeutic effects of erlotinib in various animal liver injury models and reported that erlotinib effectively inhibits EGFR signaling and prevents the development of HCC. Another animal model study revealed that gefitinib reduces the formation of HCC tumor nodules, effectively inhibiting the progression of HCC (129).
Cetuximab is an EGFR-targeting monoclonal antibody that was first approved by the United States FDA in 2004 for the treatment of advanced colorectal cancer. Common side effects include skin reactions, fatigue, diarrhea, and hypomagnesemia (130). A phase II clinical trial targeting advanced HCC patients demonstrated that while cetuximab exhibited good safety and tolerability, it showed no significant anti-tumor activity against HCC (117). Subsequently, Asnacios et al. (118) conducted a phase II clinical trial for advanced HCC using a combination of gemcitabine and oxaliplatin with cetuximab. The results indicated a disease control rate of 20%, with 40% of patients achieving disease stabilization, demonstrating some therapeutic efficacy and good tolerability. A recent phase Ib clinical trial of regorafenib in combination with cetuximab for the treatment of advanced, refractory solid tumors, including HCC, showed that patients treated with regorafenib and a standard dose of cetuximab had good tolerance and a low incidence of adverse toxic reactions, and thus the therapy was efficacious (119).
Targeting ErbB2/3/4 for HCC treatment
Compared to other cancer types, HCC typically exhibits lower levels of HER2 expression, resulting in less research on HER2 inhibitors in HCC. Trastuzumab, a humanized monoclonal antibody, targets the HER2 receptor by binding to its extracellular subdomain IV. This interaction inhibits signal transduction and promotes antibody-dependent cellular cytotoxicity, among other mechanisms, effectively combating tumor growth (131). Currently, it is primarily used in the treatment of patients with HER2-positive breast cancer and gastric cancer (132,133).
A study conducted in vitro revealed that trastuzumab alone has certain anti-tumor effects on liver cancer cell lines (134). Sharafutdinova et al. (135) developed an HER2/neu-expressing mouse liver cancer model and showed that trastuzumab treatment significantly curtails tumor growth, underscoring its potential therapeutic efficacy. Shi et al. (60) demonstrated that the use of trastuzumab in a rat model for HCC treatment can reduce tumor size and inhibit tumor metastasis. in vitro analyses showed that high concentrations of trastuzumab inhibit cell proliferation. In contrast to the aforementioned findings, Hsu et al. (136) reported that trastuzumab did not have significant anti-tumor effects on liver cancer cell lines. Currently, there are no clinical trials evaluating the use of trastuzumab for the treatment of HCC, an area that remains to be explored.
Lapatinib, a dual small-molecule TKI targeting EGFR and HER2 developed by GlaxoSmithKline, has been approved by the FDA for the treatment of HER2-positive advanced or metastatic breast cancer following chemotherapy or trastuzumab failure (137). An in vitro study has shown that lapatinib induces autophagic cell death in human liver cancer cells, demonstrating its ability to inhibit HCC proliferation and metastasis (138). However, in phase II clinical trials, lapatinib did not show significant efficacy in treating patients with HCC (120,121).
Afatinib, a second-generation irreversible inhibitor targeting the ErbB family, including EGFR, HER2, and HER4, was approved by the FDA and European Medicine Agency in 2013 for treating advanced EGFR mutation-positive NSCLC in adults (139). A pre-clinical study has confirmed that afatinib exerts a significant inhibitory effect on liver cancer cells, leading to the notable suppression of their proliferation, invasion, and migration (140). Specifically, Chen et al. (141) showed that afatinib reduces the activity of the ERK signaling pathway in liver cancer cell lines and decreases the expression levels of VEGF and matrix metalloproteinase 9, leading to reduced viability, migration, and invasion of liver cancer cells, thereby inhibiting tumor development. This finding underscores the potential of afatinib as a promising candidate for the treatment of liver cancer.
Canertinib is a panTKI that simultaneously suppresses the activity of HER1 (EGFR), HER2, and HER4. Similar to lapatinib, it achieves favorable therapeutic outcomes in HCC cell lines characterized by mutation signature cluster 1 expression (142). Concurrent research has shown that canertinib inhibits the growth of xenograft tumors in MAN2A1-FER cells and prevents their metastasis in mice (143).
Neratinib is an oral irreversible inhibitor of ErbB1/2/4 that was approved by the FDA in 2017 as an adjuvant treatment for HER2-positive breast cancer (144). Notably, 11% of HCC patients have the HER2 H878Y mutation (145). Hu et al. (146) reported that neratinib exhibits compelling sensitivity to this mutation, suggesting that these patients may be a potential beneficiary group.
Based on studies conducted on various HCC cell lines, Lee et al. (147) discovered that pelitinib, an EGFR small-molecule TKI, induces Twist1 by inhibiting the MAPK and Akt signaling pathways, thereby suppressing the EMT activity of HCC cells and consequently inhibiting the migration and invasion of Huh7 cells. Additionally, the novel EGFR-TKI EKB-569 significantly blocks the cell cycle of HCC cells, exhibiting greater efficacy than first-generation reversible EGFR-TKIs (148). AST1306 is an orally active, irreversible small-molecule inhibitor that targets the EGFR, HER2, and HER4 signaling pathways. A phase I trial investigating AST1306 demonstrated that the oral administration of AST1306 was safe and well tolerated, and AST1306 exhibited preliminary anti-tumor activity (149).
Overall, HCC is a highly heterogeneous tumor, the occurrence of which can be triggered by a variety of factors, including viral infections (e.g., HBV and HCV), alcohol consumption, metabolism, and other genetic and environmental factors. As a result, the functions of pathways, such as the ErbB pathway, may vary among different tumors or subgroups, leading to variations in the efficacy of these drugs. As we gain a deeper understanding of the role of the ErbB pathway in the pathogenesis of HCC, an increasing number of localized and systemic targeted drugs may emerge, bringing new hope to patients with HCC. Beyond the drugs mentioned, many targeted therapies against the ErbB pathway, such as tucatinib and dacomitinib, have yet to be explored in pre-clinical trials for HCC. The advent of novel targeted therapies holds tremendous therapeutic potential for HCC, necessitating ongoing research and exploration to develop more effective and safer treatment methods. This is crucial for improving the therapeutic landscape for HCC and enhancing patient outcomes.
ErbB-driven resistance and related targeted therapies
Targeted therapies, which address pathways, such as tumor angiogenesis, tumor cell proliferation, and the TME, are a crucial treatment modality for advanced HCC, and effectively mitigate disease progression and improve patient prognosis. However, over time, some patients develop reduced efficacy and treatment insensitivity, posing a significant challenge of acquired resistance in targeted therapy. Investigating resistance mechanisms and seeking novel therapeutic approaches or strategies to overcome drug resistance are critical issues that targeted therapies need to address. Primary and acquired resistance in HCC is a complex phenomenon involving various factors, such as signaling pathways, hypoxia, cellular autophagy, EMT, and the TME (150).
Hypoxia is a common phenomenon in the TME that significantly affects the biological behavior and metabolism of cancer cells by activating hypoxia-inducible factors (HIFs), particularly HIF-1α, to promote metabolic reprogramming, thus enhancing multidrug resistance in tumors (151). In HCC, hypoxia-mediated pleomorphic adenoma gene-like 2 and HIF-1/2α signaling pathways increase resistance to erlotinib, and 2-methoxyestradiol (2ME2) restores the efficacy of erlotinib by disrupting this signaling pathway (152). An experiment investigating the efficacy of erlotinib and sorafenib in treating HCC organoids and cell lines revealed that erlotinib induces VEGF production in liver cancer cells, leading to drug resistance (153). The overexpression of C-X-C chemokine receptor 4 (CXCR4) has been found to be associated with resistance to gefitinib in HCC. CXCR4 promotes gefitinib resistance in HCC through the caveolin-1 signaling pathway and c-Met signaling pathway (154). The treatment of HCC cells with gefitinib may induce cancer stem cell resistance through the increased nuclear translocation of insulin-like growth factor 1 receptor and the upregulation of Cluster of Differentiation 133 (CD133) expression (155).
Autophagy is an essential intracellular process of self-digestion that degrades and recycles damaged organelles, protein aggregates, and other cellular components through the lysosomal pathway to maintain cellular stability and meet the demands of biosynthesis (156). Autophagy plays dual roles in tumor initiation and progression, acting as a tumor-suppressive mechanism in the early stages but aiding tumor cells in adapting to stress and promoting their survival and growth once a tumor forms (157). Tumor cells enhance autophagy to counteract the cytotoxicity induced by chemotherapy, radiotherapy, or targeted therapy, thereby reducing the effectiveness of drugs. Autophagy is a significant factor in resistance to EGFR-targeted therapy in HCC. Li et al. (158) demonstrated that the upregulation of p57 activates the PI3K/AKT/mTOR signaling pathway, thereby attenuating the protective effects of autophagy and promoting the response of HCC cell lines to erlotinib or cetuximab.
EMT not only plays a critical role in the metastatic process of cancer but also contributes to the development of resistance to various anti-tumor treatments, leading to a loss of sensitivity. KIAA1199 is an intracellular protein that is overexpressed in a variety of tumor types. Xu et al. (159) reported that KIAA1199 enhances the transmission of EGF signals, accelerates the activation of EMT-related transcription factors, directly affects the loss of cell adhesion and cell polarity, and promotes the transition of epithelial cells to mesenchymal cells. This not only enhances the migration and invasion capabilities of liver cancer cells but also exacerbates cell resistance to targeted therapeutic drugs. EMT has been reported to induce resistance to erlotinib and gefitinib in various types of tumors (160,161). Fuchs et al. (162) analyzed the sensitivity of 12 human liver cancer cell lines to erlotinib, gefitinib, and cetuximab by categorizing them into epithelial and mesenchymal types, and found that drug sensitivity was greater in epithelial cell lines. Additionally, reducing integrin-linked kinase activity and AKT activation in mesenchymal cell lines increases the sensitivity to EGFR inhibitors. Another study revealed that the ECM molecule laminin-5 counteracts the continuous dephosphorylation of AKT induced by gefitinib (restoring p-AKT), thereby reducing the sensitivity of HCC to gefitinib (163).
The mutation of EGFR T790M plays a critical role in the rapid onset of resistance to EGFR-TKIs among patients (164). Third-generation EGFR-TKIs, such as osimertinib, initially proved effective in treating these mutated tumors, but resistance emerged shortly thereafter, possibly due to the cis-p.Cys797Ser (C797S) mutation, inactivation of Retinoblastoma 1, and transformation of tumor tissue (165). EGFR C797S mutations, located in the kinase-binding site, also lead to resistance to third-generation irreversible EGFR inhibitors (166). EGFR, HER2, and MET amplification not only affects the initial response to treatment but also plays a role in tumor evasion by targeted therapies, affecting sensitivity and resistance to EGFR-TKIs while driving tumor growth and survival (167,168).
The heterogeneity of tumors, the plasticity of HCC tumor cells, and escape mechanisms pose additional challenges for HCC-targeted therapy. The ErbB signaling pathway also mediates resistance to other targeted drugs. Currently, drugs such as sorafenib and lenvatinib are approved as first-line treatments for patients with unresectable advanced HCC (169). EGFR plays a pivotal role in mediating sorafenib resistance by activating downstream signaling pathways, such as the RAF/MEK/ERK and PI3K/AKT pathways (170). Kruppel-like factor 4 (KLF4) is a zinc-finger transcription factor involved in cell growth, differentiation, and cell cycle regulation (171). Pang et al. (172) reported a positive feedback loop between EGFR and KLF4, where nuclear EGFR induces KLF4 transcription by binding to its promoter, and vice versa. Additionally, EGFR may promote KLF4 expression by activating the RAF/MEK/ERK signaling pathway, thus contributing to sorafenib resistance in HCC. Research has revealed that lenvatinib achieves an overall response rate of approximately 24% in patients with HCC, with inevitable resistance to subsequent treatments affecting patient prognosis (173). A recent study suggests that the activation of EGFR may contribute to lenvatinib resistance (174). Further, clinical trials have demonstrated that combining lenvatinib with gefitinib significantly improves clinical outcomes (175). EGFR is significantly expressed in lenvatinib-resistant HCC cell lines, and Miyazaki et al. (176) discovered through whole-genome transcriptome analysis that the EGFR-PI3K-AKT pathway is overly activated. By inhibiting this pathway, resistance to lenvatinib can be overcome (176).
In summary, the abnormal activation of the ErbB family signaling pathway plays a crucial role in the occurrence and development of cancer. Targeting these specific molecules can effectively block their aberrant signal transduction, inhibiting tumor cell proliferation and survival. Moreover, targeting molecules or pathways relevant to cancer can reduce side effects, improving patient tolerance. The abnormal activation of the ErbB signaling pathway also affects the function of immune cells in the tumor microenvironment. Inhibiting ErbB signaling can improve the infiltration and function of immune cells, disrupt tumor cell immune escape mechanisms, restore the immune system’s ability to recognize and kill tumors, and enhance anti-tumor immune responses. Therefore, these molecules are promising targets for anti-cancer drugs. The continuous development of novel inhibitors and antibody drugs targeting the ErbB signaling pathway in the future will provide new hope for patients.
To date, the exploration and understanding of resistance mechanisms, particularly those concerning the ErbB pathway, in targeted therapies for liver cancer are still in their early stages. Therefore, more reliable clinical model studies are needed to deepen our understanding of HCC oncology, reduce the occurrence of drug treatment resistance, and benefit more patients with advanced liver cancer.
Advances in and prospects of ErbB combinational therapies
In clinical practice, the combined application of pharmacological treatments or their combination with interventional therapies has demonstrated significant benefits in prolonging patient survival and enhancing quality of life. This not only brings hope to patients but also gradually shifts the traditional treatment paradigms for HCC. With the rise and evolution of precision medicine, the implementation of combined treatment strategies, particularly among patients with advanced HCC, has shown greater therapeutic effects compared to single treatment modalities.
ErbB family inhibitors combined with other therapies have shown significant therapeutic potential in most cancers, including breast cancer, and head and neck cancer (177,178). Based on the results of the CLEOPATRA phase III clinical trial, the combination of pertuzumab and trastuzumab with chemotherapy has been established as the standard first-line treatment for HER2-positive breast cancer, achieving significant success (179). Breast cancer research has shown that the combination of lapatinib and paclitaxel slows the occurrence of brain metastasis compared to the combination of trastuzumab and paclitaxel as a first-line treatment (180). The combination of cetuximab and radiotherapy is an effective treatment regimen for patients with locally advanced head and neck cancer, significantly improving their survival rates (181). Additionally, studies conducted both in vitro and in vivo have shown that both cetuximab and radiation therapy synergistically reduce cell proliferation and induce apoptosis (182,183).
Multiple studies have shown the potential of applying anti-ErbB-targeted drugs in combination with other therapeutic strategies for HCC treatment. miR-34a is a microRNA that inhibits tumor growth. Erlotinib and miR-34a exhibit strong synergistic effects on HCC cell models, significantly inhibiting tumor cell growth compared to monotherapy (184). As mentioned earlier, a previous study has shown that the combination of erlotinib with doxorubicin, docetaxel, or SN-38 exhibits significant synergistic anti-tumor effects on HCC cell lines (Huh-7 and HepG2) (185). Current phase II and III clinical trials have investigated the use of erlotinib in combination with bevacizumab or docetaxel. However, due to various factors, such as sample size, the clinical efficacy of these treatments remains controversial. Recent research has revealed that the combined use of erlotinib and 2ME2 has synergistic effects on combating liver cancer, inducing apoptosis in hypoxic liver cancer cells and inhibiting their stem cell characteristics (152). Yu et al. (140) reported that the combination of afatinib and an anti-programmed death-1 (PD1) agent inhibits the ErbB2 signaling pathway and indirectly activates the STAT3 pathway, leading to the upregulation of programmed death ligand-1 (PD-L1) expression. This process not only suppresses the direct growth and spread of tumor cells but also modulates the immune microenvironment, enhancing immunotherapy efficacy in liver cancer. The use of natural remedies in adjunctive cancer therapy is also receiving increasing attention. Ethoxy-erianin phosphate (EBTP) is a low-toxicity, antiangiogenic compound derived from the structural modification of the natural product erianin. When combined with afatinib, EBTP effectively targets the VEGF and EGFR signaling pathways, inhibiting the proliferation, motility, and angiogenesis of liver cancer cells and tumor vasculature (186).
Challenges and future directions
In this article, we discussed ErbB signaling, particularly that involving the EGFR family, which plays a significant role in the development and progression of HCC. One of the major challenges in targeting ErbB signaling in HCC is the inherent tumor heterogeneity. HCC tumors exhibit diverse molecular profiles, including variations in ErbB receptor expression levels and downstream signaling pathways. This heterogeneity complicates the development of targeted therapies and necessitates personalized treatment approaches. In addition, resistance to EGFR-targeted therapies is another significant challenge in HCC treatment. Tumor cells can develop various mechanisms to evade EGFR inhibition, such as the upregulation of alternative signaling pathways, mutations in downstream effectors, or activation of compensatory feedback loops. Overcoming these resistance mechanisms requires a deeper understanding of the molecular drivers of HCC progression and the development of combination therapies that target multiple signaling pathways simultaneously.
Conclusions
In conclusion, in the era of precision medicine, exploration into tumor treatment has entered a new age, particularly in the treatment of malignant tumors, where ErbB-targeted therapy has demonstrated tremendous potential and prospects. Despite numerous challenges, including the singularity of treatment strategies, suboptimal clinical efficacy, and controversies in treatment, advancements in technology and in-depth research on targeted therapy and immunotherapy hold promise for the continuous improvement of treatment strategies through the ongoing discovery and validation of new therapeutic approaches and targets. In the future, we believe that through in-depth research and the application of ErbB-targeted therapy, broader avenues will be opened for the treatment of HCC and other tumors, leading to more personalized and precise treatment approaches.
Acknowledgments
The authors would like to thank Biorender.com for providing illustration support.
Funding: This research was funded by
Footnote
Reporting Checklist: The authors have completed the Narrative Review reporting checklist. Available at https://tcr.amegroups.com/article/view/10.21037/tcr-24-837/rc
Peer Review File: Available at https://tcr.amegroups.com/article/view/10.21037/tcr-24-837/prf
Conflicts of Interest: All authors have completed the ICMJE uniform disclosure form (available at https://tcr.amegroups.com/article/view/10.21037/tcr-24-837/coif). The authors have no conflicts of interest to declare.
Ethical Statement: The authors are accountable for all aspects of the work in ensuring that questions related to the accuracy or integrity of any part of the work are appropriately investigated and resolved.
Open Access Statement: This is an Open Access article distributed in accordance with the Creative Commons Attribution-NonCommercial-NoDerivs 4.0 International License (CC BY-NC-ND 4.0), which permits the non-commercial replication and distribution of the article with the strict proviso that no changes or edits are made and the original work is properly cited (including links to both the formal publication through the relevant DOI and the license). See: https://creativecommons.org/licenses/by-nc-nd/4.0/.
References
- Sung H, Ferlay J, Siegel RL, et al. Global Cancer Statistics 2020: GLOBOCAN Estimates of Incidence and Mortality Worldwide for 36 Cancers in 185 Countries. CA Cancer J Clin 2021;71:209-49. [Crossref] [PubMed]
- Nagaraju GP, Dariya B, Kasa P, et al. Epigenetics in hepatocellular carcinoma. Semin Cancer Biol 2022;86:622-32. [Crossref] [PubMed]
- Kulik L, El-Serag HB. Epidemiology and Management of Hepatocellular Carcinoma. Gastroenterology 2019;156:477-491.e1. [Crossref] [PubMed]
- Mishra S, Kumari S, Srivastava P, et al. Genomic profiling of gallbladder carcinoma: Targetable mutations and pathways involved. Pathol Res Pract 2022;232:153806. [Crossref] [PubMed]
- Sharma SV, Settleman J. ErbBs in lung cancer. Exp Cell Res 2009;315:557-71. [Crossref] [PubMed]
- Elizalde PV, Cordo Russo RI, Chervo MF, et al. ErbB-2 nuclear function in breast cancer growth, metastasis and resistance to therapy. Endocr Relat Cancer 2016;23:T243-57. [Crossref] [PubMed]
- Van Cutsem E, Sagaert X, Topal B, et al. Gastric cancer. Lancet 2016;388:2654-64. [Crossref] [PubMed]
- Kumar R, George B, Campbell MR, et al. HER family in cancer progression: From discovery to 2020 and beyond. Adv Cancer Res 2020;147:109-60. [Crossref] [PubMed]
- Cameron D, Piccart-Gebhart MJ, Gelber RD, et al. 11 years’ follow-up of trastuzumab after adjuvant chemotherapy in HER2-positive early breast cancer: final analysis of the HERceptin Adjuvant (HERA) trial. Lancet 2017;389:1195-205. [Crossref] [PubMed]
- Yi M, He T, Wang K, et al. Comparison of gefitinib plus chemotherapy versus gefitinib alone for advanced non small cell lung cancer: A meta analysis. Clinics (Sao Paulo) 2023;78:100152. [Crossref] [PubMed]
- Cymer F, Schneider D. Transmembrane helix-helix interactions involved in ErbB receptor signaling. Cell Adh Migr 2010;4:299-312. [Crossref] [PubMed]
- VanSlyke JK, Boswell BA, Musil LS. ErbBs in Lens Cell Fibrosis and Secondary Cataract. Invest Ophthalmol Vis Sci 2023;64:6. [Crossref] [PubMed]
- Li X, Wu C, Chen N, et al. PI3K/Akt/mTOR signaling pathway and targeted therapy for glioblastoma. Oncotarget 2016;7:33440-50. [Crossref] [PubMed]
- Komurasaki T, Toyoda H, Uchida D, et al. Epiregulin binds to epidermal growth factor receptor and ErbB-4 and induces tyrosine phosphorylation of epidermal growth factor receptor, ErbB-2, ErbB-3 and ErbB-4. Oncogene 1997;15:2841-8. [Crossref] [PubMed]
- Singh AB, Harris RC. Autocrine, paracrine and juxtacrine signaling by EGFR ligands. Cell Signal 2005;17:1183-93. [Crossref] [PubMed]
- Révillion F, Lhotellier V, Hornez L, et al. ErbB/HER ligands in human breast cancer, and relationships with their receptors, the bio-pathological features and prognosis. Ann Oncol 2008;19:73-80. [Crossref] [PubMed]
- Yotsumoto F, Yagi H, Suzuki SO, et al. Validation of HB-EGF and amphiregulin as targets for human cancer therapy. Biochem Biophys Res Commun 2008;365:555-61. [Crossref] [PubMed]
- Busser B, Sancey L, Brambilla E, et al. The multiple roles of amphiregulin in human cancer. Biochim Biophys Acta 2011;1816:119-31. [PubMed]
- Castillo J, Erroba E, Perugorría MJ, et al. Amphiregulin contributes to the transformed phenotype of human hepatocellular carcinoma cells. Cancer Res 2006;66:6129-38. [Crossref] [PubMed]
- Berasain C, Avila MA. Amphiregulin. Semin Cell Dev Biol 2014;28:31-41. [Crossref] [PubMed]
- Chung E, Graves-Deal R, Franklin JL, et al. Differential effects of amphiregulin and TGF-alpha on the morphology of MDCK cells. Exp Cell Res 2005;309:149-60. [Crossref] [PubMed]
- Schneider MR, Wolf E. The epidermal growth factor receptor ligands at a glance. J Cell Physiol 2009;218:460-6. [Crossref] [PubMed]
- Yamazaki S, Iwamoto R, Saeki K, et al. Mice with defects in HB-EGF ectodomain shedding show severe developmental abnormalities. J Cell Biol 2003;163:469-75. [Crossref] [PubMed]
- Prenzel N, Zwick E, Daub H, et al. EGF receptor transactivation by G-protein-coupled receptors requires metalloproteinase cleavage of proHB-EGF. Nature 1999;402:884-8. [Crossref] [PubMed]
- Llovet JM, Villanueva A, Lachenmayer A, et al. Advances in targeted therapies for hepatocellular carcinoma in the genomic era. Nat Rev Clin Oncol 2015;12:408-24. [Crossref] [PubMed]
- Jang J, To C, De Clercq DJH, et al. Mutant-Selective Allosteric EGFR Degraders are Effective Against a Broad Range of Drug-Resistant Mutations. Angew Chem Int Ed Engl 2020;59:14481-9. [Crossref] [PubMed]
- Kang L, Zhang ZH, Zhao Y. SCAMP3 is regulated by miR-128-3p and promotes the metastasis of hepatocellular carcinoma cells through EGFR-MAPK p38 signaling pathway. Am J Transl Res 2020;12:7870-84. [PubMed]
- Lemmon MA, Schlessinger J. Cell signaling by receptor tyrosine kinases. Cell 2010;141:1117-34. [Crossref] [PubMed]
- Kannangai R, Sahin F, Torbenson MS. EGFR is phosphorylated at Ty845 in hepatocellular carcinoma. Mod Pathol 2006;19:1456-61. [Crossref] [PubMed]
- Greulich H, Chen TH, Feng W, et al. Oncogenic transformation by inhibitor-sensitive and -resistant EGFR mutants. PLoS Med 2005;2:e313. [Crossref] [PubMed]
- Politi K, Zakowski MF, Fan PD, et al. Lung adenocarcinomas induced in mice by mutant EGF receptors found in human lung cancers respond to a tyrosine kinase inhibitor or to down-regulation of the receptors. Genes Dev 2006;20:1496-510. [Crossref] [PubMed]
- Fitch KR, McGowan KA, van Raamsdonk CD, et al. Genetics of dark skin in mice. Genes Dev 2003;17:214-28. [Crossref] [PubMed]
- Scheving LA, Zhang X, Stevenson MC, et al. Loss of hepatocyte ERBB3 but not EGFR impairs hepatocarcinogenesis. Am J Physiol Gastrointest Liver Physiol 2015;309:G942-54. [Crossref] [PubMed]
- Alqahtani A, Khan Z, Alloghbi A, et al. Hepatocellular Carcinoma: Molecular Mechanisms and Targeted Therapies. Medicina (Kaunas) 2019;55:526. [Crossref] [PubMed]
- Song S, Yu Z, You Y, et al. EGFR/MET promotes hepatocellular carcinoma metastasis by stabilizing tumor cells and resisting to RTKs inhibitors in circulating tumor microemboli. Cell Death Dis 2022;13:351. [Crossref] [PubMed]
- Llovet JM, Pinyol R, Kelley RK, et al. Molecular pathogenesis and systemic therapies for hepatocellular carcinoma. Nat Cancer 2022;3:386-401. [Crossref] [PubMed]
- Nikolova D, Chalovska V, Ivanova MG, et al. Immunohistochemical Expression of Epidermal Growth Factor Receptor in Hepatocellular Carcinoma. Pril 2018;39:21-8. (Makedon Akad Nauk Umet Odd Med Nauki). [Crossref] [PubMed]
- Ji X, Chen X, Zhang B, et al. T-box transcription factor 19 promotes hepatocellular carcinoma metastasis through upregulating EGFR and RAC1. Oncogene 2022;41:2225-38. [Crossref] [PubMed]
- Wen DS, Huang LC, Bu XY, et al. DNA methylation-activated full-length EMX1 facilitates metastasis through EMX1-EGFR-ERK axis in hepatocellular carcinoma. Cell Death Dis 2023;14:769. [Crossref] [PubMed]
- Su T, Huang M, Liao J, et al. Insufficient Radiofrequency Ablation Promotes Hepatocellular Carcinoma Metastasis Through N6-Methyladenosine mRNA Methylation-Dependent Mechanism. Hepatology 2021;74:1339-56. [Crossref] [PubMed]
- Sun J, Zhou C, Zhao Y, et al. Quiescin sulfhydryl oxidase 1 promotes sorafenib-induced ferroptosis in hepatocellular carcinoma by driving EGFR endosomal trafficking and inhibiting NRF2 activation. Redox Biol 2021;41:101942. [Crossref] [PubMed]
- Li KS, Zhu XD, Liu HD, et al. NT5DC2 promotes tumor cell proliferation by stabilizing EGFR in hepatocellular carcinoma. Cell Death Dis 2020;11:335. [Crossref] [PubMed]
- Villanueva A, Chiang DY, Newell P, et al. Pivotal role of mTOR signaling in hepatocellular carcinoma. Gastroenterology 2008;135:1972-83, 1983.e1-11.
- Zhangyuan G, Wang F, Zhang H, et al. VersicanV1 promotes proliferation and metastasis of hepatocellular carcinoma through the activation of EGFR-PI3K-AKT pathway. Oncogene 2020;39:1213-30. [Crossref] [PubMed]
- Buzzai M, Bauer DE, Jones RG, et al. The glucose dependence of Akt-transformed cells can be reversed by pharmacologic activation of fatty acid beta-oxidation. Oncogene 2005;24:4165-73. [Crossref] [PubMed]
- Crouchet E, Li S, Sojoodi M, et al. Hepatocellular carcinoma chemoprevention by targeting the angiotensin-converting enzyme and EGFR transactivation. JCI Insight 2022;7:e159254. [Crossref] [PubMed]
- Cmet S, Fabris C, Fattovich G, et al. Carriage of the EGF rs4444903 A>G functional polymorphism associates with disease progression in chronic HBV infection. Clin Exp Immunol 2012;167:296-302. [Crossref] [PubMed]
- Tanabe KK, Lemoine A, Finkelstein DM, et al. Epidermal growth factor gene functional polymorphism and the risk of hepatocellular carcinoma in patients with cirrhosis. JAMA 2008;299:53-60. [Crossref] [PubMed]
- Feng X, Jia Y, Zhang Y, et al. Ubiquitination of UVRAG by SMURF1 promotes autophagosome maturation and inhibits hepatocellular carcinoma growth. Autophagy 2019;15:1130-49. [Crossref] [PubMed]
- Zhou Q, Huang T, Jiang Z, et al. Upregulation of SNX5 predicts poor prognosis and promotes hepatocellular carcinoma progression by modulating the EGFR-ERK1/2 signaling pathway. Oncogene 2020;39:2140-55. [Crossref] [PubMed]
- Gazdar AF, Shigematsu H, Herz J, et al. Mutations and addiction to EGFR: the Achilles ‘heal’ of lung cancers? Trends Mol Med 2004;10:481-6. [Crossref] [PubMed]
- Sommerfeld A, Reinehr R, Häussinger D. Bile acid-induced epidermal growth factor receptor activation in quiescent rat hepatic stellate cells can trigger both proliferation and apoptosis. J Biol Chem 2009;284:22173-83. [Crossref] [PubMed]
- Yang C, Zeisberg M, Mosterman B, et al. Liver fibrosis: insights into migration of hepatic stellate cells in response to extracellular matrix and growth factors. Gastroenterology 2003;124:147-59. [Crossref] [PubMed]
- Lupberger J, Zeisel MB, Xiao F, et al. EGFR and EphA2 are host factors for hepatitis C virus entry and possible targets for antiviral therapy. Nat Med 2011;17:589-95. [Crossref] [PubMed]
- Takada M, Toi M. Neoadjuvant treatment for HER2-positive breast cancer. Chin Clin Oncol 2020;9:32. [Crossref] [PubMed]
- Wang HB, Liao XF, Zhang J. Clinicopathological factors associated with HER2-positive gastric cancer: A meta-analysis. Medicine (Baltimore) 2017;96:e8437. [Crossref] [PubMed]
- Ahcene Djaballah S, Daniel F, Milani A, et al. HER2 in Colorectal Cancer: The Long and Winding Road From Negative Predictive Factor to Positive Actionable Target. Am Soc Clin Oncol Educ Book 2022;42:1-14. [Crossref] [PubMed]
- Raghav KPS, Moasser MM. Molecular Pathways and Mechanisms of HER2 in Cancer Therapy. Clin Cancer Res 2023;29:2351-61. [Crossref] [PubMed]
- Tsumura T, Doi K, Marusawa H. Precision Medicine of Hepatobiliary and Pancreatic Cancers: Focusing on Clinical Trial Outcomes. Cancers (Basel) 2022;14:3674. [Crossref] [PubMed]
- Shi JH, Guo WZ, Jin Y, et al. Recognition of HER2 expression in hepatocellular carcinoma and its significance in postoperative tumor recurrence. Cancer Med 2019;8:1269-78. [Crossref] [PubMed]
- Altimari A, Fiorentino M, Gabusi E, et al. Investigation of ErbB1 and ErbB2 expression for therapeutic targeting in primary liver tumours. Dig Liver Dis 2003;35:332-8. [Crossref] [PubMed]
- Xian ZH, Zhang SH, Cong WM, et al. Overexpression/amplification of HER-2/neu is uncommon in hepatocellular carcinoma. J Clin Pathol 2005;58:500-3. [Crossref] [PubMed]
- Zhang C, Peng L, Zhang Y, et al. The identification of key genes and pathways in hepatocellular carcinoma by bioinformatics analysis of high-throughput data. Med Oncol 2017;34:101. [Crossref] [PubMed]
- Zhang H, Yuan X, Yang Y, et al. Cathelicidin LL-37 promotes EMT, migration and metastasis of hepatocellular carcinoma cells in vitro and mouse model. Cell Adh Migr 2023;17:20-34. [Crossref] [PubMed]
- Hung CM, Huang WC, Pan HL, et al. Hepatitis B virus X upregulates HuR protein level to stabilize HER2 expression in hepatocellular carcinoma cells. Biomed Res Int 2014;2014:827415. [Crossref] [PubMed]
- Maurer CA, Friess H, Kretschmann B, et al. Increased expression of erbB3 in colorectal cancer is associated with concomitant increase in the level of erbB2. Hum Pathol 1998;29:771-7. [Crossref] [PubMed]
- Steinkamp MP, Low-Nam ST, Yang S, et al. erbB3 is an active tyrosine kinase capable of homo- and heterointeractions. Mol Cell Biol 2014;34:965-77. [Crossref] [PubMed]
- Sithanandam G, Anderson LM. The ERBB3 receptor in cancer and cancer gene therapy. Cancer Gene Ther 2008;15:413-48. [Crossref] [PubMed]
- El-Gamal MI, Mewafi NH, Abdelmotteleb NE, et al. A Review of HER4 (ErbB4) Kinase, Its Impact on Cancer, and Its Inhibitors. Molecules 2021;26:7376. [Crossref] [PubMed]
- Androutsopoulos G, Styliara I, Zarogianni E, et al. The ErbB Signaling Network and Its Potential Role in Endometrial Cancer. Epigenomes 2023;7:24. [Crossref] [PubMed]
- Schoeberl B, Pace EA, Fitzgerald JB, et al. Therapeutically targeting ErbB3: a key node in ligand-induced activation of the ErbB receptor-PI3K axis. Sci Signal 2009;2:ra31. [Crossref] [PubMed]
- Hyman DM, Piha-Paul SA, Won H, et al. HER kinase inhibition in patients with HER2- and HER3-mutant cancers. Nature 2018;554:189-94. [Crossref] [PubMed]
- Stevenson BW, Gorman MA, Koach J, et al. A structural view of PA2G4 isoforms with opposing functions in cancer. J Biol Chem 2020;295:16100-12. [Crossref] [PubMed]
- Yang X, Chen Y, Li M, et al. ERBB3 methylation and immune infiltration in tumor microenvironment of cervical cancer. Sci Rep 2022;12:8112. [Crossref] [PubMed]
- Hsieh SY, He JR, Hsu CY, et al. Neuregulin/erythroblastic leukemia viral oncogene homolog 3 autocrine loop contributes to invasion and early recurrence of human hepatoma. Hepatology 2011;53:504-16. [Crossref] [PubMed]
- Ni Q, Chen Z, Zheng Q, et al. Epithelial V-like antigen 1 promotes hepatocellular carcinoma growth and metastasis via the ERBB-PI3K-AKT pathway. Cancer Sci 2020;111:1500-13. [Crossref] [PubMed]
- Thybusch-Bernhardt A, Beckmann S, Juhl H. Comparative analysis of the EGF-receptor family in pancreatic cancer: expression of HER-4 correlates with a favourable tumor stage. Int J Surg Investig 2001;2:393-400. [PubMed]
- Vidal GA, Clark DE, Marrero L, et al. A constitutively active ERBB4/HER4 allele with enhanced transcriptional coactivation and cell-killing activities. Oncogene 2007;26:462-6. [Crossref] [PubMed]
- Williams EE, Trout LJ, Gallo RM, et al. A constitutively active ErbB4 mutant inhibits drug-resistant colony formation by the DU-145 and PC-3 human prostate tumor cell lines. Cancer Lett 2003;192:67-74. [Crossref] [PubMed]
- Muraoka-Cook RS, Sandahl MA, Strunk KE, et al. ErbB4 splice variants Cyt1 and Cyt2 differ by 16 amino acids and exert opposing effects on the mammary epithelium in vivo. Mol Cell Biol 2009;29:4935-48. [Crossref] [PubMed]
- Kaushansky A, Gordus A, Budnik BA, et al. System-wide investigation of ErbB4 reveals 19 sites of Tyr phosphorylation that are unusually selective in their recruitment properties. Chem Biol 2008;15:808-17. [Crossref] [PubMed]
- Liu Y, Song L, Ni H, et al. ERBB4 acts as a suppressor in the development of hepatocellular carcinoma. Carcinogenesis 2017;38:465-73. [Crossref] [PubMed]
- Li Y, Wu B, Sun R, et al. miR-93-5p knockdown repressed hepatocellular carcinoma progression via increasing ERBB4 and TETs-dependent DNA demethylation. Autoimmunity 2021;54:547-60. [Crossref] [PubMed]
- Kataria H, Alizadeh A, Shahriary GM, et al. Neuregulin-1 promotes remyelination and fosters a pro-regenerative inflammatory response in focal demyelinating lesions of the spinal cord. Glia 2018;66:538-61. [Crossref] [PubMed]
- Robinson MS. Forty Years of Clathrin-coated Vesicles. Traffic 2015;16:1210-38. [Crossref] [PubMed]
- Vieira AV, Lamaze C, Schmid SL. Control of EGF receptor signaling by clathrin-mediated endocytosis. Science 1996;274:2086-9. [Crossref] [PubMed]
- Huang F, Khvorova A, Marshall W, et al. Analysis of clathrin-mediated endocytosis of epidermal growth factor receptor by RNA interference. J Biol Chem 2004;279:16657-61. [Crossref] [PubMed]
- Sigismund S, Argenzio E, Tosoni D, et al. Clathrin-mediated internalization is essential for sustained EGFR signaling but dispensable for degradation. Dev Cell 2008;15:209-19. [Crossref] [PubMed]
- Banushi B, Joseph SR, Lum B, et al. Endocytosis in cancer and cancer therapy. Nat Rev Cancer 2023;23:450-73. [Crossref] [PubMed]
- Haglund K, Dikic I. The role of ubiquitylation in receptor endocytosis and endosomal sorting. J Cell Sci 2012;125:265-75. [Crossref] [PubMed]
- Liu Y, Calmel C, Desbois-Mouthon C, et al. Regulation of the EGFR/ErbB signalling by clathrin in response to various ligands in hepatocellular carcinoma cell lines. J Cell Mol Med 2020;24:8091-102. [Crossref] [PubMed]
- Zhou Y, Sakurai H. New trend in ligand-induced EGFR trafficking: A dual-mode clathrin-mediated endocytosis model. J Proteomics 2022;255:104503. [Crossref] [PubMed]
- Quail DF, Joyce JA. Microenvironmental regulation of tumor progression and metastasis. Nat Med 2013;19:1423-37. [Crossref] [PubMed]
- Schreiber RD, Old LJ, Smyth MJ. Cancer immunoediting: integrating immunity’s roles in cancer suppression and promotion. Science 2011;331:1565-70. [Crossref] [PubMed]
- Li M, Liu F, Zhang F, et al. Genomic ERBB2/ERBB3 mutations promote PD-L1-mediated immune escape in gallbladder cancer: a whole-exome sequencing analysis. Gut 2019;68:1024-33. [Crossref] [PubMed]
- Li M, Zhang Z, Li X, et al. Whole-exome and targeted gene sequencing of gallbladder carcinoma identifies recurrent mutations in the ErbB pathway. Nat Genet 2014;46:872-6. [Crossref] [PubMed]
- Zhang Y, Zuo C, Liu L, et al. Single-cell RNA-sequencing atlas reveals an MDK-dependent immunosuppressive environment in ErbB pathway-mutated gallbladder cancer. J Hepatol 2021;75:1128-41. [Crossref] [PubMed]
- Wang S, Zhang Y, Wang Y, et al. Amphiregulin Confers Regulatory T Cell Suppressive Function and Tumor Invasion via the EGFR/GSK-3β/Foxp3 Axis. J Biol Chem 2016;291:21085-95. [Crossref] [PubMed]
- Dong ZY, Zhang JT, Liu SY, et al. EGFR mutation correlates with uninflamed phenotype and weak immunogenicity, causing impaired response to PD-1 blockade in non-small cell lung cancer. Oncoimmunology 2017;6:e1356145. [Crossref] [PubMed]
- Pollack BP, Sapkota B, Cartee TV. Epidermal growth factor receptor inhibition augments the expression of MHC class I and II genes. Clin Cancer Res 2011;17:4400-13. [Crossref] [PubMed]
- Watanabe S, Hayashi H, Haratani K, et al. Mutational activation of the epidermal growth factor receptor down-regulates major histocompatibility complex class I expression via the extracellular signal-regulated kinase in non-small cell lung cancer. Cancer Sci 2019;110:52-60. [Crossref] [PubMed]
- Collins FS, Varmus H. A new initiative on precision medicine. N Engl J Med 2015;372:793-5. [Crossref] [PubMed]
- Dahl E, Sadr-Nabavi A, Klopocki E, et al. Systematic identification and molecular characterization of genes differentially expressed in breast and ovarian cancer. J Pathol 2005;205:21-8. [Crossref] [PubMed]
- Philip PA, Mahoney MR, Allmer C, et al. Phase II study of Erlotinib (OSI-774) in patients with advanced hepatocellular cancer. J Clin Oncol 2005;23:6657-63. [Crossref] [PubMed]
- Thomas MB, Chadha R, Glover K, et al. Phase 2 study of erlotinib in patients with unresectable hepatocellular carcinoma. Cancer 2007;110:1059-67. [Crossref] [PubMed]
- Thomas MB, Morris JS, Chadha R, et al. Phase II trial of the combination of bevacizumab and erlotinib in patients who have advanced hepatocellular carcinoma. J Clin Oncol 2009;27:843-50. [Crossref] [PubMed]
- Kaseb AO, Garrett-Mayer E, Morris JS, et al. Efficacy of bevacizumab plus erlotinib for advanced hepatocellular carcinoma and predictors of outcome: final results of a phase II trial. Oncology 2012;82:67-74. [Crossref] [PubMed]
- Philip PA, Mahoney MR, Holen KD, et al. Phase 2 study of bevacizumab plus erlotinib in patients with advanced hepatocellular cancer. Cancer 2012;118:2424-30. [Crossref] [PubMed]
- Yau T, Wong H, Chan P, et al. Phase II study of bevacizumab and erlotinib in the treatment of advanced hepatocellular carcinoma patients with sorafenib-refractory disease. Invest New Drugs 2012;30:2384-90. [Crossref] [PubMed]
- Chiorean EG, Ramasubbaiah R, Yu M, et al. Phase II trial of erlotinib and docetaxel in advanced and refractory hepatocellular and biliary cancers: Hoosier Oncology Group GI06-101. Oncologist 2012;17:13. [Crossref] [PubMed]
- Govindarajan R, Siegel E, Makhoul I, et al. Bevacizumab and erlotinib in previously untreated inoperable and metastatic hepatocellular carcinoma. Am J Clin Oncol 2013;36:254-7. [Crossref] [PubMed]
- Hsu CH, Kang YK, Yang TS, et al. Bevacizumab with erlotinib as first-line therapy in Asian patients with advanced hepatocellular carcinoma: a multicenter phase II study. Oncology 2013;85:44-52. [Crossref] [PubMed]
- Zhu AX, Rosmorduc O, Evans TR, et al. SEARCH: a phase III, randomized, double-blind, placebo-controlled trial of sorafenib plus erlotinib in patients with advanced hepatocellular carcinoma. J Clin Oncol 2015;33:559-66. [Crossref] [PubMed]
- Kaseb AO, Morris JS, Iwasaki M, et al. Phase II trial of bevacizumab and erlotinib as a second-line therapy for advanced hepatocellular carcinoma. Onco Targets Ther 2016;9:773-80. [Crossref] [PubMed]
- Patt YZ, Murad W, Fekrazad MH, et al. INST OX-05-024: first line gemcitabine, oxaliplatin, and erlotinib for primary hepatocellular carcinoma and bile duct cancers: a multicenter Phase II trial. Cancer Med 2017;6:2042-51. [Crossref] [PubMed]
- Thomas MB, Garrett-Mayer E, Anis M, et al. A Randomized Phase II Open-Label Multi-Institution Study of the Combination of Bevacizumab and Erlotinib Compared to Sorafenib in the First-Line Treatment of Patients with Advanced Hepatocellular Carcinoma. Oncology 2018;94:329-39. [Crossref] [PubMed]
- Zhu AX, Stuart K, Blaszkowsky LS, et al. Phase 2 study of cetuximab in patients with advanced hepatocellular carcinoma. Cancer 2007;110:581-9. [Crossref] [PubMed]
- Asnacios A, Fartoux L, Romano O, et al. Gemcitabine plus oxaliplatin (GEMOX) combined with cetuximab in patients with progressive advanced stage hepatocellular carcinoma: results of a multicenter phase 2 study. Cancer 2008;112:2733-9. [Crossref] [PubMed]
- Weekes C, Lockhart AC, Lee JJ, et al. A phase 1b study evaluating the safety and pharmacokinetics of regorafenib in combination with cetuximab in patients with advanced solid tumors. Int J Cancer 2019;145:2450-8. [Crossref] [PubMed]
- Ramanathan RK, Belani CP, Singh DA, et al. A phase II study of lapatinib in patients with advanced biliary tree and hepatocellular cancer. Cancer Chemother Pharmacol 2009;64:777-83. [Crossref] [PubMed]
- Bekaii-Saab T, Markowitz J, Prescott N, et al. A multi-institutional phase II study of the efficacy and tolerability of lapatinib in patients with advanced hepatocellular carcinomas. Clin Cancer Res 2009;15:5895-901. [Crossref] [PubMed]
- Albanell J, Codony J, Rovira A, et al. Mechanism of action of anti-HER2 monoclonal antibodies: scientific update on trastuzumab and 2C4. Adv Exp Med Biol 2003;532:253-68. [Crossref] [PubMed]
- Qi M, Tian Y, Li W, et al. ERK inhibition represses gefitinib resistance in non-small cell lung cancer cells. Oncotarget 2018;9:12020-34. [Crossref] [PubMed]
- Hammel P, Huguet F, van Laethem JL, et al. Effect of Chemoradiotherapy vs Chemotherapy on Survival in Patients With Locally Advanced Pancreatic Cancer Controlled After 4 Months of Gemcitabine With or Without Erlotinib: The LAP07 Randomized Clinical Trial. JAMA 2016;315:1844-53. [Crossref] [PubMed]
- Bang YJ, Van Cutsem E, Feyereislova A, et al. Trastuzumab in combination with chemotherapy versus chemotherapy alone for treatment of HER2-positive advanced gastric or gastro-oesophageal junction cancer (ToGA): a phase 3, open-label, randomised controlled trial. Lancet 2010;376:687-97. Erratum in: Lancet 2010;376:1302. [Crossref] [PubMed]
- Kris MG, Natale RB, Herbst RS, et al. Efficacy of gefitinib, an inhibitor of the epidermal growth factor receptor tyrosine kinase, in symptomatic patients with non-small cell lung cancer: a randomized trial. JAMA 2003;290:2149-58. [Crossref] [PubMed]
- Shepherd FA, Rodrigues Pereira J, Ciuleanu T, et al. Erlotinib in previously treated non-small-cell lung cancer. N Engl J Med 2005;353:123-32. [Crossref] [PubMed]
- Fuchs BC, Hoshida Y, Fujii T, et al. Epidermal growth factor receptor inhibition attenuates liver fibrosis and development of hepatocellular carcinoma. Hepatology 2014;59:1577-90. [Crossref] [PubMed]
- Schiffer E, Housset C, Cacheux W, et al. Gefitinib, an EGFR inhibitor, prevents hepatocellular carcinoma development in the rat liver with cirrhosis. Hepatology 2005;41:307-14. [Crossref] [PubMed]
- Cunningham D, Humblet Y, Siena S, et al. Cetuximab monotherapy and cetuximab plus irinotecan in irinotecan-refractory metastatic colorectal cancer. N Engl J Med 2004;351:337-45. [Crossref] [PubMed]
- Barish R, Gates E, Barac A. Trastuzumab-Induced Cardiomyopathy. Cardiol Clin 2019;37:407-18. [Crossref] [PubMed]
- von Minckwitz G, Huang CS, Mano MS, et al. Trastuzumab Emtansine for Residual Invasive HER2-Positive Breast Cancer. N Engl J Med 2019;380:617-28. [Crossref] [PubMed]
- Chung HC, Bang YJ, S, Fuchs C, et al. First-line pembrolizumab/placebo plus trastuzumab and chemotherapy in HER2-positive advanced gastric cancer: KEYNOTE-811. Future Oncol 2021;17:491-501. [Crossref] [PubMed]
- Tatebe H, Shimizu M, Shirakami Y, et al. Synergistic growth inhibition by 9-cis-retinoic acid plus trastuzumab in human hepatocellular carcinoma cells. Clin Cancer Res 2008;14:2806-12. [Crossref] [PubMed]
- Sharafutdinova TA, Petrov AV. Creation of a model of HER2/neu-positive transplantable tumor on the immunocompetent mice. Vopr Onkol 2016;62:133-7. [PubMed]
- Hsu C, Huang CL, Hsu HC, et al. HER-2/neu overexpression is rare in hepatocellular carcinoma and not predictive of anti-HER-2/neu regulation of cell growth and chemosensitivity. Cancer 2002;94:415-20. [Crossref] [PubMed]
- Kopper L. Lapatinib: a sword with two edges. Pathol Oncol Res 2008;14:1-8. [Crossref] [PubMed]
- Chen YJ, Chi CW, Su WC, et al. Lapatinib induces autophagic cell death and inhibits growth of human hepatocellular carcinoma. Oncotarget 2014;5:4845-54. [Crossref] [PubMed]
- Wecker H, Waller CF. Afatinib. Recent Results Cancer Res 2018;211:199-215. [Crossref] [PubMed]
- Yu C, Zhang X, Wang M, et al. Afatinib combined with anti-PD1 enhances immunotherapy of hepatocellular carcinoma via ERBB2/STAT3/PD-L1 signaling. Front Oncol 2023;13:1198118. [Crossref] [PubMed]
- Chen Y, Chen X, Ding X, et al. Afatinib, an EGFR inhibitor, decreases EMT and tumorigenesis of Huh 7 cells by regulating the ERK VEGF/MMP9 signaling pathway. Mol Med Rep 2019;20:3317-25. [Crossref] [PubMed]
- Zhang Y, Liu Z, Li J, et al. Prognosis and Personalized Treatment Prediction in Different Mutation-Signature Hepatocellular Carcinoma. J Hepatocell Carcinoma 2023;10:241-55. [Crossref] [PubMed]
- Chen ZH, Yu YP, Tao J, et al. MAN2A1-FER Fusion Gene Is Expressed by Human Liver and Other Tumor Types and Has Oncogenic Activity in Mice. Gastroenterology 2017;153:1120-1132.e15. [Crossref] [PubMed]
- Echavarria I, López-Tarruella S, Márquez-Rodas I, et al. Neratinib for the treatment of HER2-positive early stage breast cancer. Expert Rev Anticancer Ther 2017;17:669-79. [Crossref] [PubMed]
- Bekaii-Saab T, Williams N, Plass C, et al. A novel mutation in the tyrosine kinase domain of ERBB2 in hepatocellular carcinoma. BMC Cancer 2006;6:278. [Crossref] [PubMed]
- Hu Z, Wan X, Hao R, et al. Phosphorylation of mutationally introduced tyrosine in the activation loop of HER2 confers gain-of-function activity. PLoS One 2015;10:e0123623. [Crossref] [PubMed]
- Lee S, Kang E, Lee U, et al. Role of pelitinib in the regulation of migration and invasion of hepatocellular carcinoma cells via inhibition of Twist1. BMC Cancer 2023;23:703. [Crossref] [PubMed]
- Kim H, Lim HY. Novel EGFR-TK inhibitor EKB-569 inhibits hepatocellular carcinoma cell proliferation by AKT and MAPK pathways. J Korean Med Sci 2011;26:1563-8. [Crossref] [PubMed]
- Zhang J, Cao J, Li J, et al. A phase I study of AST1306, a novel irreversible EGFR and HER2 kinase inhibitor, in patients with advanced solid tumors. J Hematol Oncol 2014;7:22. [Crossref] [PubMed]
- Tang W, Chen Z, Zhang W, et al. The mechanisms of sorafenib resistance in hepatocellular carcinoma: theoretical basis and therapeutic aspects. Signal Transduct Target Ther 2020;5:87. [Crossref] [PubMed]
- Rankin EB, Giaccia AJ. The role of hypoxia-inducible factors in tumorigenesis. Cell Death Differ 2008;15:678-85. [Crossref] [PubMed]
- Zheng S, Ni J, Li Y, et al. 2-Methoxyestradiol synergizes with Erlotinib to suppress hepatocellular carcinoma by disrupting the PLAGL2-EGFR-HIF-1/2α signaling loop. Pharmacol Res 2021;169:105685. [Crossref] [PubMed]
- Sieghart W, Pinter M, Dauser B, et al. Erlotinib and sorafenib in an orthotopic rat model of hepatocellular carcinoma. J Hepatol 2012;57:592-9. [Crossref] [PubMed]
- Zhao D, Yang Z, Chen C, et al. CXCR4 promotes gefitinib resistance of Huh7 cells by activating the c-Met signaling pathway. FEBS Open Bio 2021;11:3115-25. [Crossref] [PubMed]
- Bodzin AS, Wei Z, Hurtt R, et al. Gefitinib resistance in HCC mahlavu cells: upregulation of CD133 expression, activation of IGF-1R signaling pathway, and enhancement of IGF-1R nuclear translocation. J Cell Physiol 2012;227:2947-52. [Crossref] [PubMed]
- Yang Z, Klionsky DJ. Mammalian autophagy: core molecular machinery and signaling regulation. Curr Opin Cell Biol 2010;22:124-31. [Crossref] [PubMed]
- Akkoç Y, Gözüaçık D. Autophagy and liver cancer. Turk J Gastroenterol 2018;29:270-82. [Crossref] [PubMed]
- Li WY, Li Q, Jing L, et al. P57-mediated autophagy promotes the efficacy of EGFR inhibitors in hepatocellular carcinoma. Liver Int 2019;39:147-57. [Crossref] [PubMed]
- Xu Y, Xu H, Li M, et al. KIAA1199 promotes sorafenib tolerance and the metastasis of hepatocellular carcinoma by activating the EGF/EGFR-dependent epithelial-mesenchymal transition program. Cancer Lett 2019;454:78-89. [Crossref] [PubMed]
- Thomson S, Buck E, Petti F, et al. Epithelial to mesenchymal transition is a determinant of sensitivity of non-small-cell lung carcinoma cell lines and xenografts to epidermal growth factor receptor inhibition. Cancer Res 2005;65:9455-62. [Crossref] [PubMed]
- Frederick BA, Helfrich BA, Coldren CD, et al. Epithelial to mesenchymal transition predicts gefitinib resistance in cell lines of head and neck squamous cell carcinoma and non-small cell lung carcinoma. Mol Cancer Ther 2007;6:1683-91. [Crossref] [PubMed]
- Fuchs BC, Fujii T, Dorfman JD, et al. Epithelial-to-mesenchymal transition and integrin-linked kinase mediate sensitivity to epidermal growth factor receptor inhibition in human hepatoma cells. Cancer Res 2008;68:2391-9. [Crossref] [PubMed]
- Giannelli G, Azzariti A, Fransvea E, et al. Laminin-5 offsets the efficacy of gefitinib (‘Iressa’) in hepatocellular carcinoma cells. Br J Cancer 2004;91:1964-9. [Crossref] [PubMed]
- Yu HA, Arcila ME, Rekhtman N, et al. Analysis of tumor specimens at the time of acquired resistance to EGFR-TKI therapy in 155 patients with EGFR-mutant lung cancers. Clin Cancer Res 2013;19:2240-7. [Crossref] [PubMed]
- Ren X, Cai X, Li J, et al. Histological transformation of lung adenocarcinoma to small cell lung cancer with mutant C797S conferring acquired resistance to osimertinib. J Int Med Res 2020;48:300060520927918. [Crossref] [PubMed]
- Planchard D, Loriot Y, André F, et al. EGFR-independent mechanisms of acquired resistance to AZD9291 in EGFR T790M-positive NSCLC patients. Ann Oncol 2015;26:2073-8. [Crossref] [PubMed]
- Takezawa K, Pirazzoli V, Arcila ME, et al. HER2 amplification: a potential mechanism of acquired resistance to EGFR inhibition in EGFR-mutant lung cancers that lack the second-site EGFRT790M mutation. Cancer Discov 2012;2:922-33. [Crossref] [PubMed]
- Lutterbach B, Zeng Q, Davis LJ, et al. Lung cancer cell lines harboring MET gene amplification are dependent on Met for growth and survival. Cancer Res 2007;67:2081-8. [Crossref] [PubMed]
- Vogel A, Qin S, Kudo M, et al. Lenvatinib versus sorafenib for first-line treatment of unresectable hepatocellular carcinoma: patient-reported outcomes from a randomised, open-label, non-inferiority, phase 3 trial. Lancet Gastroenterol Hepatol 2021;6:649-58. [Crossref] [PubMed]
- Nishida N, Kitano M, Sakurai T, et al. Molecular Mechanism and Prediction of Sorafenib Chemoresistance in Human Hepatocellular Carcinoma. Dig Dis 2015;33:771-9. [Crossref] [PubMed]
- Ghaleb AM, Yang VW. Krüppel-like factor 4 (KLF4): What we currently know. Gene 2017;611:27-37. [Crossref] [PubMed]
- Pang L, Xu L, Yuan C, et al. Activation of EGFR-KLF4 positive feedback loop results in acquired resistance to sorafenib in hepatocellular carcinoma. Mol Carcinog 2019;58:2118-26. [Crossref] [PubMed]
- Kudo M, Finn RS, Qin S, et al. Lenvatinib versus sorafenib in first-line treatment of patients with unresectable hepatocellular carcinoma: a randomised phase 3 non-inferiority trial. Lancet 2018;391:1163-73. [Crossref] [PubMed]
- He X, Hikiba Y, Suzuki Y, et al. EGFR inhibition reverses resistance to lenvatinib in hepatocellular carcinoma cells. Sci Rep 2022;12:8007. [Crossref] [PubMed]
- Jin H, Shi Y, Lv Y, et al. EGFR activation limits the response of liver cancer to lenvatinib. Nature 2021;595:730-4. [Crossref] [PubMed]
- Miyazaki K, Morine Y, Xu C, et al. Curcumin-Mediated Resistance to Lenvatinib via EGFR Signaling Pathway in Hepatocellular Carcinoma. Cells 2023;12:612. [Crossref] [PubMed]
- Bianchini G, Kiermaier A, Bianchi GV, et al. Biomarker analysis of the NeoSphere study: pertuzumab, trastuzumab, and docetaxel versus trastuzumab plus docetaxel, pertuzumab plus trastuzumab, or pertuzumab plus docetaxel for the neoadjuvant treatment of HER2-positive breast cancer. Breast Cancer Res 2017;19:16. [Crossref] [PubMed]
- Bonner JA, Harari PM, Giralt J, et al. Radiotherapy plus cetuximab for squamous-cell carcinoma of the head and neck. N Engl J Med 2006;354:567-78. [Crossref] [PubMed]
- Swain SM, Miles D, Kim SB, et al. Pertuzumab, trastuzumab, and docetaxel for HER2-positive metastatic breast cancer (CLEOPATRA): end-of-study results from a double-blind, randomised, placebo-controlled, phase 3 study. Lancet Oncol 2020;21:519-30. [Crossref] [PubMed]
- Loibl S, Poortmans P, Morrow M, et al. Breast cancer. Lancet 2021;397:1750-69. [Crossref] [PubMed]
- Bonner JA, Harari PM, Giralt J, et al. Radiotherapy plus cetuximab for locoregionally advanced head and neck cancer: 5-year survival data from a phase 3 randomised trial, and relation between cetuximab-induced rash and survival. Lancet Oncol 2010;11:21-8. Erratum in: Lancet Oncol 2010;11:14. [Crossref] [PubMed]
- Bonner JA, Raisch KP, Trummell HQ, et al. Enhanced apoptosis with combination C225/radiation treatment serves as the impetus for clinical investigation in head and neck cancers. J Clin Oncol 2000;18:47S-53S. [PubMed]
- Saleh MN, Raisch KP, Stackhouse MA, et al. Combined modality therapy of A431 human epidermoid cancer using anti-EGFr antibody C225 and radiation. Cancer Biother Radiopharm 1999;14:451-63. [Crossref] [PubMed]
- Zhao J, Kelnar K, Bader AG. In-depth analysis shows synergy between erlotinib and miR-34a. PLoS One 2014;9:e89105. [Crossref] [PubMed]
- Huether A, Höpfner M, Sutter AP, et al. Erlotinib induces cell cycle arrest and apoptosis in hepatocellular cancer cells and enhances chemosensitivity towards cytostatics. J Hepatol 2005;43:661-9. [Crossref] [PubMed]
- Chen J, Liu J, Xu B, et al. Ethoxy-erianin phosphate and afatinib synergistically inhibit liver tumor growth and angiogenesis via regulating VEGF and EGFR signaling pathways. Toxicol Appl Pharmacol 2022;438:115911. [Crossref] [PubMed]