Naringin inhibits cisplatin resistance of ovarian cancer cells by inhibiting autophagy mediated by the TGF-β2/smad2 pathway
Highlight box
Key findings
• Naringin inhibits cisplatin (DDP) resistance in ovarian cancer (OC) cells by inhibiting autophagy mediated by the transforming growth factor-β2 (TGF-β2)/SMAD family member 2 (smad2) pathway.
What is known and what is new?
• OC is a common cancer of the reproductive system, and DDP resistance is a major challenge to improve the quality of life of OC patients.
• Naringin can induce apoptosis of OC cells and delay the occurrence of drug resistance in OC cells.
What is the implication, and what should change now?
• Our findings implied that naringin plays an essential role in OC resistance, and indicates that naringin can block autophagy to reverse DDP resistance in OC cells with TGF-β2/smad2-related factors. Combining naringin with DDP may serve as an effective adjutant therapy for OC.
Introduction
Ovarian cancer (OC), as a disease of the reproductive system, has caused great trouble to female survival and is also one of the major female diseases (1). Some research institutes predicted that the OC mortality rate would be 4.2/100,000 of all ages in the European Union in 2022 (2). Early-stage OC cannot be detected with the aid of current diagnostic tools, so OC patients are often treated in the late stage (3). Nowadays, cytoreductive surgery combined with cisplatin (DPP) is the initial intervention mode for most patients (4). However, chemotherapy resistance is a major factor contributing to poor therapeutic efficacy, leading to tumor recurrence and metastasis, as well as patient mortality, which is the main cause for poor treatment outcomes of OC (5). Therefore, the search for novel DDP-based combination therapy has become a top priority.
Traditional Chinese medicine (TCM) has long been used for cancer treatment in Asian countries. TCM can not only improve the life quality and survival period of cancer patients, but also reduce chemotherapy resistance and chemotherapy-induced adverse effects (6,7). As a natural flavonoid drug, the Chinese herbal medicine, naringin, has been shown to have anti-cancer potential. It is involved in and regulates various activities and signal transduction processes in cancer development (8). Naringin is commonly used as a phosphatase and tensin homolog agonist to interfere with the biological action of OC cells (9). In animal experiments, naringin inhibits tumor growth (10). Importantly, naringin can reverse DDP resistance in OC cells (11). While the regulatory effect of naringin on OC has been previously demonstrated from multiple angles, the specific molecular mechanism by which naringin inhibits DDP resistance has not yet been reported.
DNA damage and inhibition of DNA synthesis are cytotoxic mechanisms of DDP in tumors, after which various signal transduction pathways in cancer cells, including autophagy, are activated. Autophagy induced by extracellular regulated kinase (ERK) or YES proto-oncogene 1 (YES1) can promote DDP resistance of OC cells (12,13). At the same time, TCM intervention in autophagy activity is also found to alter drug sensitivity of OC cells. Artesunate regulates drug-sensitive activity by interfering with autophagy in colon cancer cells (14). Solamargine also regulates cancer cell resistance via autophagy (15). In previous reports, naringin was also demonstrated to inhibit autophagy (16,17). Therefore, it is possible that naringin may inhibit DDP resistance by blocking autophagy in OC. This study focused on the effect of naringin on DDP resistance in OC cells and the specific mechanism of naringin mediating autophagy. We present this article in accordance with the MDAR and ARRIVE reporting checklists (available at https://tcr.amegroups.com/article/view/10.21037/tcr-23-2156/rc).
Methods
Cells and reagents
Human OC cells (SKOV3 and A2780) were purchased from the Chinese Academy of Sciences (Beijing, China). Human OC DDP-resistant cell line (SKOV3-DDP and A2780-DDP) was purchased from Jinyuan Biotechnology (Shanghai, China). Roswell Park Memorial Institute (RPMI) 1640 culture-medium was purchased from Thermo Fisher Scientific (cat. No.11875119, Gibco, NY, USA). Fetal bovine serum (FBS) was also purchased from Thermo Fisher Scientific (cat. No.10100, Gibco). Penicillin Streptomycin Liquid was obtained from Beijing Solaibo Technology Co., LTD. (cat. No. P1400, Beijing, China). Naringin (cat. No. HY-N0153), DDP (cat. No. HY-17394) and SRI-011381 (cat. No. HY-100347) were respectively purchased from MedChemExpress (New Jersey, USA). Rapamycin (cat. No. 553210) was purchased from Sigma-Aldrich (St. Louis, MO, USA). The antibodies of transforming growth factor-β2 (TGF-β2) (ab238249), phosphorylation SMAD family member 2 (p-smad2) (ab280888), SMAD family member 2 (smad2) (ab40855), Sequestosome 1 (SQSTM1/p62, hereinafter referred to as p62) (ab56416) and horseradish peroxidase (HRP) conjugated secondary (ab6721, 1b6789) were obtained from abcam (Cambridge, UK). The antibody of microtubule-associated protein 1 light chain 3 (LC3) (cat. No. 4108S) was purchased from Cell Signaling Technology (Boston, USA).
3-(4,5-dimethylthiazol-2-yl)-2,5-diphenyltetrazolium bromide (MTT) assay
The MTT (Sigma, St. Louis, MO, USA) was dissolved into a 5 mg/mL solution using phosphate buffered saline (PBS) and stored at 4 °C for use. The cell count was adjusted to a concentration of 5×104/mL, seeded in a 96-well plate with 100 µL per well, and treated with DDP (0, 1, 2, 4, 8, 16, or 32 µg/mL) for 48 h in cytoxicity assays. In addition, cells were also treated with 18 µg/mL DDP, 20 µmol/L naringin (11) and 10 nmol/L rapamycin for 48 h. Then, 10 µL of MTT solution (5 mg/mL, 0.5% MTT) was added to each well for a continued culture for 4 h. The cell viability was measured at 490 nm by enzyme-labeled assay, and the half maximal inhibitory concentration (IC50) value of DDP was calculated by fitting the equation.
Apoptosis assay
The Alexa fluor 488 Annexin V/dead cell apoptosis Kit (Thermo Fisher Scientific) was used for performing flow cytometry. To analyze the apoptosis of OC cells, samples were fixed using 70% ethanol at 4 °C for 4 h and incubated with Annexin V-fluorescein isothiocyanate (FITC)-propidium iodide (PI) for 5 min in the dark. The apoptosis level was evaluated by flow cytometry (Becon Dickinson FACS Calibur, NY, USA).
Immunofluorescence assay
Cells were added onto EZ slides (Millipore, Boston, MA, USA) and treated with 4% paraformaldehyde (Sigma) for 0.5 h. Subsequently, the cells were permeabilized with 0.5% Triton X-100 (Sigma). The samples were incubated with LC3 antibody (1:1,000) overnight at 4 °C, washed three times with phosphate buffer for 5 min each time, and incubated with the secondary antibody (1:200) at room temperature for 2 h in the dark. Nuclei were stained with 4’,6-diamidino-2’-phenylindole (DAPI) for 5 min at room temperature, and BX53 Fluorescence Microscope was used for imaging (Olympus, Tokyo, Japan).
Western blot
Total protein was extracted with radio immunoprecipitation assay (RIPA) lysis buffer (Beyotime Institute of Biotechnology, Shanghai, China). 10% sodium dodecyl sulfate-polyacrylamide gel electrophoresis (SDS-PAGE) was used for protein isolation. The isolated proteins were transferred onto polyvinylidene fluoride (PVDF) membrane (Millipore, Bedford, MA, USA), and first incubated with antibodies p62 (1:1,000), LC3 (1:1,000), TGF-β2 (1:1,000), smad2 (1:1,000), p-smad2 (1:1,000) and GAPDH (1:1,000) overnight at 4 °C. Following three times of washing with phosphate buffer (5 min each time), the membrane was then incubated with the secondary antibodies (1:5,000) at room temperature in the dark for 2 h. Immunoblots were visualized using an enhanced chemiluminescence kit. ImageJ software was used to analyze the protein expression gray value.
Xenograft model of OC cells
To establish a tumor model of OC, SKOV3-DDP cells (1×106 cells) were subcutaneously implanted into 18 BALB/c nude mice [Hangzhou Medical College Laboratory Animal Center, China. Production License: SCXK(Zhe)2019-0002; License number: SYXK(Zhe)2019-0011]. The mice were grouped using a random number table method (n=6). After the tumor volume reached over 50 mm3, the model mice are intraperitoneally injected with 2 mg/kg naringin and/or 3 mg/kg DDP every day for 10 consecutive days. Tumor volume was evaluated with slide calipers (0.5 × length × width × height) at 5-day intervals up to day 20. Later, the mice were euthanized, and samples were collected, photographed, and weighed. The experiment was approved by the Institutional Animal Care and Use Committee (IACUC) of Zhejiang Center of Laboratory Animals (ZJCLA) (Approval No. ZJCLA-IACUC-20040156), in compliance with institutional guidelines for the care and use of animals. A protocol was prepared before the study without registration.
Statistical analysis
The data were processed with GraphPad Prism software (version 8.0) and analyzed as mean ± standard deviation (SD). One-way analysis of variance with Bonferroni correction post-hoc was performed for the comparison of multiple independent variables. Each experiment was repeated at least three times. This study followed the principle of blind method, and neither the experimental operator nor the data analyst knew the specific experimental grouping. P values <0.05 were considered statistically significant.
Results
Naringin inhibited the drug resistance of SKOV3-DDP and A2780-DDP cell lines
To determine the sensitivity and resistance of DDP resistant cells to DDP, SKOV3/SKOV3-DDP and A2780/A2780-DDP cells were treated with different doses of DDP. SKOV3-DPP (IC50 =17.32 µg/mL) and A2780-DDP (IC50 =19.36 µg/mL) cells were shown to have higher IC50 values than SKOV3 (IC50 =5.56 µg/mL) and A2780 (IC50 =6.31 µg/mL) cells (Figure 1A). Further, the OC resistant cell lines were treated with naringin. Compared with the untreated OC resistant cell lines, naringin-treated cell lines were more sensitive to DDP (Figure 1B). Since the IC50 value of both OC cells was close to 18 µg/mL, 18 µg/mL DDP was defined for cell treatment in subsequent experiments. In addition, we observed that DDP and naringin individually increased the apoptosis level of both OC cells. Moreover, the combination of DDP and naringin showed a more significant promoting effect on apoptosis (Figure 1C). These results suggest that naringin can enhance the sensitivity of OC resistant cells to DDP, and the efficacy can be enhanced when used in combination with DDP.
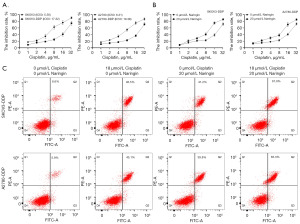
Naringin inhibited autophagy in OC resistant cell lines
When SKOV3-DDP and A2780-DDP cells were treated with naringin, the level of p62 was upregulated while the level of LC3 was inhibited (Figure 2A,2B). Immunofluorescence assay further confirmed that naringin could reduce the proportion of LC3 positive cells (Figure 2C). Subsequent studies further clarified whether naringin regulates DDP resistance in OC by inhibiting autophagy.
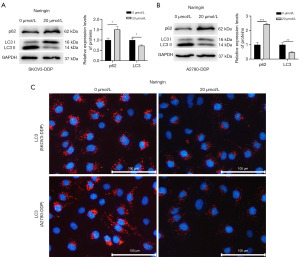
Naringin inhibited the autophagy of OC cell lines to inhibit DDP resistance
To confirm whether naringin regulates DDP resistance in OC by inhibiting autophagy, the autophagy agonist, rapamycin, was used. Compared to the use of naringin or DDP alone, the combination of naringin and DDP enhanced the inhibition of SKOV3-DDP cells by DDP, while rapamycin reversed this effect (Figure 3A). Flow cytometry results also revealed higher levels of apoptosis following combined treatment with DDP and naringin as compared to treatment with DDP alone (Figure 3B). The cell apoptosis in the combined treatment group was inhibited by rapamycin (Figure 3B).
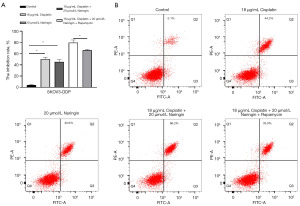
Naringin regulated the activity of OC cell autophagy via inhibiting the TGF-β2/smad2 pathway
Next, the effect of naringin on the TGF-β2/smad2 signal axis was further investigated. The level of TGF-β2 and p-smad2 in SKOV3-DDP cell lines was inhibited by naringin (Figure 4A). The TGF-β agonist, SRI-011381, promoted the level of TGF-β2/smad2-related factors (Figure 4A). In order to clarify whether the autophagy of OC resistant cell lines would be inhibited after the TGF-β2/smad2 signaling axis was blocked, Western blot and immunofluorescence assay were used. The results showed that the level of LC3 in SKOV3-DDP cells was downregulated by naringin, and such inhibitory effect was reversed after treatment with SRI-011381 (Figure 4B,4C). p62 level was higher in naringin-treated cells. Compared with naringin-treated cells, cells after the combined treatment of SRI-011381 and naringin showed lower expression of p62 (Figure 4B).
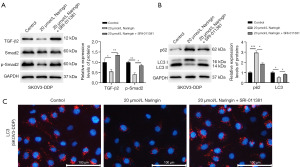
In vivo verification of the inhibition of drug resistance and autophagy in OC by naringin
The conclusion drawn from in vitro experiments that naringin could regulate autophagy and reverse DDP resistance in OC resistant cell lines was further verified in vivo. The SKOV3-DDP cell line was used for construction of a mouse tumor xenograft model. After the tumor model was successfully established, the model mice were treated with DDP. There were no significant changes in the tumor volume and weight after DDP treatment (Figure 5A,5B), suggesting that the mice were resistant to DDP. When being treated with naringin and DDP, the tumor volume and weight decreased significantly (Figure 5A,5B). Protein levels revealed naringin blocked autophagy in the tumor tissues of mice (Figure 5C). The levels of TGF-β2 and p-smad2 in the tumor tissues also decreased significantly (Figure 5D).
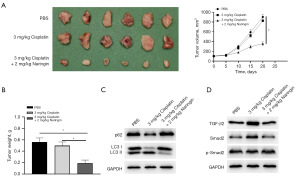
Discussion
Surgery combined with DDP is a common treatment option for patients with advanced OC. However, some OC patients may develop resistance to DDP during treatment, leading to tumor recurrence or even death (18). In the studies of DDP resistance in OC cells, many TCMs, including naringin, have exhibited remarkable properties in reversing DDP resistance (19,20). Compared with other chemotherapy drugs, TCMs has lower toxicity and is more suitable. At the same time, due to the complexity of components in TCMs, the regulatory mechanism of DDP resistance in OC cells deserve attention. In the present study, naringin enhanced the activity of DDP resistant cells to DDP. Naringin-treated OC cells were less resistant to DDP. Combined treatment with naringin and DDP resulted in higher levels of apoptosis of DDP resistant cells. Further, our results demonstrated that naringin can regulate the DDP activity of OC cells via blocking autophagy activity.
TCMs are often used in combination with chemotherapy agents in the treatment of OC by virtue of their low toxicity. Moreover, there are diverse molecular mechanisms of DDP-based combination therapy for OC patients. Some researchers found that bitter melon may enhance the DDP activity in OC cells by inducing ferroptosis and activating the AMP-activated protein kinase (AMPK) signaling pathway (21). Shikonin was found to enhance DDP sensitivity by inducing ferroptosis and reactive oxygen species (ROS) activity in OC cells (22). In the present study, naringin was found to inhibit DDP resistance in OC. Previous studies on the relationship between naringin and DDP in OC have rarely suggested that naringin may reverse DDP resistance by regulating factor-kB or Wnt/β-catenin pathways (11,19). This study investigated whether the change of DDP resistance in OC is caused by autophagy.
Autophagy is a lysosome-mediated degradation in all eukaryotic cells (23). Autophagy plays completely different roles at different stages of cancer development. There is increasing evidence that autophagy has complex effects on cancer (24,25). It has been found that the chemoresistance of OC cells to certain chemotherapy drugs is caused by inducing autophagy. Paclitaxel resistance could be promoted via inducing autophagy (26). DDP resistance was also related to autophagy in OC (27). According to the results of this study, the autophagy agonist, rapamycin, could promote drug resistance in SKOV3-DDP cells, and naringin may also inhibit OC resistance by inhibiting autophagy. The naringin-treated OC cells showed significantly increased p62 expression and reduced LC3 expression. Previous studies have focused on the influence of naringin on autophagy (28,29). Naringin can also inhibit autophagy via phosphatidylinositol 3-kinase (PI3K)/protein kinase B (AKT)/mechanistic target of rapamycin (mTOR) (16). The aforementioned research all reveals that naringin can block the autophagy of OC cells and thus inhibit DDP resistance.
The specific molecular mechanism of naringin in regulating autophagy in OC cells was further investigated. TGF-β could induce autophagy via the smad in pancreatic cancer (30). TGF-β/smad2 also activates autophagy in hepatocellular carcinoma (31). Some TCMs have also been found to regulate autophagy by blocking the TGF-β/smad related factors. Curcumin regulates oxidative stress and autophagy via inhibiting TGF-β/smad related factors (32). Quercetin reduces autophagy via TGF-β/smads signaling (33). Additionally, naringin is considered to regulate the TGF-β/smad axis (34). In our study, we identified that naringin could also inhibit TGF-β2/smad2 signal transduction. In addition, TGF-β pathway agonists also reversed the inhibition of autophagy by naringin in SKOV3-DDP cells. In vivo experimental results also revealed that naringin could inhibit the TGF-β2/smad2 signal transduction and autophagy-related factors in OC tumor tissues. These results strengthened the evidence that naringin could inhibit autophagy via the TGF-β2/smad2 signaling pathway.
Conclusions
In conclusion, the current research demonstrates that naringin plays an essential role in OC resistance, and indicates that naringin can block autophagy to reverse DDP resistance in OC cells with TGF-β2/smad2-related factors. It is worth noting the certain limitations of this study. Firstly, although the effect of naringin on the DDP resistance in OC was investigated, the specific therapeutic effect of naringin on OC patients and the possible adverse effects of naringin remain to be studied clinically. Secondly, naringin was revealed to regulate DDP resistance in OC through autophagy, and more possible molecular mechanisms including ferroptosis also need to be investigated.
Acknowledgments
Funding: This work was supported by
Footnote
Reporting Checklist: The authors have completed the MDAR and ARRIVE reporting checklists. Available at https://tcr.amegroups.com/article/view/10.21037/tcr-23-2156/rc
Data Sharing Statement: Available at https://tcr.amegroups.com/article/view/10.21037/tcr-23-2156/dss
Peer Review File: Available at https://tcr.amegroups.com/article/view/10.21037/tcr-23-2156/prf
Conflicts of Interest: All authors have completed the ICMJE uniform disclosure form (available at https://tcr.amegroups.com/article/view/10.21037/tcr-23-2156/coif). The authors have no conflicts of interest to declare.
Ethical Statement: The authors are accountable for all aspects of the work in ensuring that questions related to the accuracy or integrity of any part of the work are appropriately investigated and resolved. The experiment was approved by the Institutional Animal Care and Use Committee (IACUC) of Zhejiang Center of Laboratory Animals (ZJCLA) (Approval No. ZJCLA-IACUC-20040156), in compliance with institutional guidelines for the care and use of animals.
Open Access Statement: This is an Open Access article distributed in accordance with the Creative Commons Attribution-NonCommercial-NoDerivs 4.0 International License (CC BY-NC-ND 4.0), which permits the non-commercial replication and distribution of the article with the strict proviso that no changes or edits are made and the original work is properly cited (including links to both the formal publication through the relevant DOI and the license). See: https://creativecommons.org/licenses/by-nc-nd/4.0/.
References
- Sung H, Ferlay J, Siegel RL, et al. Global Cancer Statistics 2020: GLOBOCAN Estimates of Incidence and Mortality Worldwide for 36 Cancers in 185 Countries. CA Cancer J Clin 2021;71:209-49. [Crossref] [PubMed]
- Dalmartello M, La Vecchia C, Bertuccio P, et al. European cancer mortality predictions for the year 2022 with focus on ovarian cancer. Ann Oncol 2022;33:330-9. [Crossref] [PubMed]
- Menon U, Gentry-Maharaj A, Burnell M, et al. Ovarian cancer population screening and mortality after long-term follow-up in the UK Collaborative Trial of Ovarian Cancer Screening (UKCTOCS): a randomised controlled trial. Lancet 2021;397:2182-93. [Crossref] [PubMed]
- Li J, Qi F, Su H, et al. GRP75-faciliated Mitochondria-associated ER Membrane (MAM) Integrity controls Cisplatin-resistance in Ovarian Cancer Patients. Int J Biol Sci 2022;18:2914-31. [Crossref] [PubMed]
- Bogani G, Lopez S, Mantiero M, et al. Immunotherapy for platinum-resistant ovarian cancer. Gynecol Oncol 2020;158:484-8. [Crossref] [PubMed]
- Zhao F, Vakhrusheva O, Markowitsch SD, et al. Artesunate Impairs Growth in Cisplatin-Resistant Bladder Cancer Cells by Cell Cycle Arrest, Apoptosis and Autophagy Induction. Cells 2020;9:2643. [Crossref] [PubMed]
- Cheng Z, Li Z, Gu L, et al. Ophiopogonin B alleviates cisplatin resistance of lung cancer cells by inducing Caspase-1/GSDMD dependent pyroptosis. J Cancer 2022;13:715-27. [Crossref] [PubMed]
- Zhou J, Xia L, Zhang Y. Naringin inhibits thyroid cancer cell proliferation and induces cell apoptosis through repressing PI3K/AKT pathway. Pathol Res Pract 2019;215:152707. [Crossref] [PubMed]
- Ke X, Li L, Li J, et al. Anti-oncogenic PTEN induces ovarian cancer cell senescence by targeting P21. Cell Biol Int 2022;46:118-28. [Crossref] [PubMed]
- Farhan M. Naringin's Prooxidant Effect on Tumor Cells: Copper's Role and Therapeutic Implications. Pharmaceuticals (Basel) 2022;15:1431. [Crossref] [PubMed]
- Zhu H, Zou X, Lin S, et al. Effects of naringin on reversing cisplatin resistance and the Wnt/β-catenin pathway in human ovarian cancer SKOV3/CDDP cells. J Int Med Res 2020;48:300060519887869. [Crossref] [PubMed]
- Ferraresi A, Esposito A, Girone C, et al. Resveratrol Contrasts LPA-Induced Ovarian Cancer Cell Migration and Platinum Resistance by Rescuing Hedgehog-Mediated Autophagy. Cells 2021;10:3213. [Crossref] [PubMed]
- Zhou Y, Wang C, Ding J, et al. miR-133a targets YES1 to reduce cisplatin resistance in ovarian cancer by regulating cell autophagy. Cancer Cell Int 2022;22:15. [Crossref] [PubMed]
- Huang Z, Gan S, Zhuang X, et al. Artesunate Inhibits the Cell Growth in Colorectal Cancer by Promoting ROS-Dependent Cell Senescence and Autophagy. Cells 2022;11:2472. [Crossref] [PubMed]
- Yin S, Jin W, Qiu Y, et al. Solamargine induces hepatocellular carcinoma cell apoptosis and autophagy via inhibiting LIF/miR-192-5p/CYR61/Akt signaling pathways and eliciting immunostimulatory tumor microenvironment. J Hematol Oncol 2022;15:32. [Crossref] [PubMed]
- Wang K, Peng S, Xiong S, et al. Naringin inhibits autophagy mediated by PI3K-Akt-mTOR pathway to ameliorate endothelial cell dysfunction induced by high glucose/high fat stress. Eur J Pharmacol 2020;874:173003. [Crossref] [PubMed]
- Li Q, Liu S, Yang G, et al. Naringenin inhibits autophagy and epithelial-mesenchymal transition of human lens epithelial cells by regulating the Smad2/3 pathway. Drug Dev Res 2022;83:389-96. [Crossref] [PubMed]
- Song M, Cui M, Liu K. Therapeutic strategies to overcome cisplatin resistance in ovarian cancer. Eur J Med Chem 2022;232:114205. [Crossref] [PubMed]
- Zhu H, Gao J, Wang L, et al. In vitro study on reversal of ovarian cancer cell resistance to cisplatin by naringin via the nuclear factor-κB signaling pathway. Exp Ther Med 2018;15:2643-8. [Crossref] [PubMed]
- Wang G, Li L, Li Y, et al. Toosendanin reduces cisplatin resistance in ovarian cancer through modulating the miR-195/ERK/β-catenin pathway. Phytomedicine 2023;109:154571. [Crossref] [PubMed]
- Chan DW, Yung MM, Chan YS, et al. MAP30 protein from Momordica charantia is therapeutic and has synergic activity with cisplatin against ovarian cancer in vivo by altering metabolism and inducing ferroptosis. Pharmacol Res 2020;161:105157. [Crossref] [PubMed]
- Ni M, Zhou J, Zhu Z, et al. Shikonin and cisplatin synergistically overcome cisplatin resistance of ovarian cancer by inducing ferroptosis via upregulation of HMOX1 to promote Fe(2+) accumulation. Phytomedicine 2023;112:154701. [Crossref] [PubMed]
- Levine B, Kroemer G. Biological Functions of Autophagy Genes: A Disease Perspective. Cell 2019;176:11-42. [Crossref] [PubMed]
- Li X, He S, Ma B. Autophagy and autophagy-related proteins in cancer. Mol Cancer 2020;19:12. [Crossref] [PubMed]
- Ferro F, Servais S, Besson P, et al. Autophagy and mitophagy in cancer metabolic remodelling. Semin Cell Dev Biol 2020;98:129-38. [Crossref] [PubMed]
- Mosca L, Ilari A, Fazi F, et al. Taxanes in cancer treatment: Activity, chemoresistance and its overcoming. Drug Resist Updat 2021;54:100742. [Crossref] [PubMed]
- Zhou F, Yang X, Zhao H, et al. Down-regulation of OGT promotes cisplatin resistance by inducing autophagy in ovarian cancer. Theranostics 2018;8:5200-12. [Crossref] [PubMed]
- Guo P, Wang P, Liu L, et al. Naringin Alleviates Glucose-Induced Aging by Reducing Fat Accumulation and Promoting Autophagy in Caenorhabditis elegans. Nutrients 2023;15:907. [Crossref] [PubMed]
- Raha S, Kim SM, Lee HJ, et al. Naringin Induces Lysosomal Permeabilization and Autophagy Cell Death in AGS Gastric Cancer Cells. Am J Chin Med 2020;48:679-702. [Crossref] [PubMed]
- He R, Wang M, Zhao C, et al. TFEB-driven autophagy potentiates TGF-β induced migration in pancreatic cancer cells. J Exp Clin Cancer Res 2019;38:340. [Crossref] [PubMed]
- Duan SF, Zhang MM, Dong Q, et al. A Water-Soluble Hydrogen Sulfide Donor Suppresses the Growth of Hepatocellular Carcinoma via Inhibiting the AKT/GSK-3β/β-Catenin and TGF-β/Smad2/3 Signaling Pathways. J Oncol 2023;2023:8456852. [Crossref] [PubMed]
- Kong D, Zhang Z, Chen L, et al. Curcumin blunts epithelial-mesenchymal transition of hepatocytes to alleviate hepatic fibrosis through regulating oxidative stress and autophagy. Redox Biol 2020;36:101600. [Crossref] [PubMed]
- Wu L, Zhang Q, Mo W, et al. Quercetin prevents hepatic fibrosis by inhibiting hepatic stellate cell activation and reducing autophagy via the TGF-β1/Smads and PI3K/Akt pathways. Sci Rep 2017;7:9289. [Crossref] [PubMed]
- Ye C, Chen J, Qu Y, et al. Naringin in the repair of knee cartilage injury via the TGF-β/ALK5/Smad2/3 signal transduction pathway combined with an acellular dermal matrix. J Orthop Translat 2021;32:1-11. [Crossref] [PubMed]