Prognostic value of GARS in bladder cancer and its role in the tumor microenvironment
Highlight box
Key findings
• The lower expression of glycyl-tRNA synthetase (GARS) was closely associated with a better prognosis for bladder cancer (BC) patients.
• GARS could be a target for prognosis prediction and treatment of BC patients.
• Tumor microenvironment shows that GARS is enriched in myofibroblasts and is involved in the progression of non-muscle invasive BC to muscle invasive BC by regulating epithelial-mesenchymal transition.
• Patients with high GARS the expression in urinary extracellular vesicles (uEVs) was also at a high level.
What is known and what is new?
• GARS encodes glycyl-tRNA synthetase, one of the aminoacyl-tRNA synthetases that charge tRNAs with their cognate amino acids. The encoded enzyme is an (alpha)2 dimer which belongs to the class II family of tRNA synthetases. It has been shown as a target of autoantibodies in human autoimmune diseases, polymyositis or dermatomyositis.
• We have analyzed GARS not only from the view of tumor prognosis, but also explored the information in its microenvironment. Due to the specificity of BC, we added the study of GARS in urine.
What is the implication, and what should change now?
• GARS can be used as a prognostic and therapeutic marker for BC, we can detect GARS in uEVs instead of tissue, to provide a new, simple, noninvasive way to obtain prognostic and therapeutic markers for BC patients.
Introduction
Bladder cancer (BC) is the 10th most common form of cancer worldwide, with an estimated 549,000 new cases and 200,000 deaths in 2018 and most BC patients have a poor prognosis (1). Conventional therapies such as maximal surgical resection, chemical drug perfusion in bladder and chemotherapy of cisplatin are considered to be the best available therapeutic approaches (2). Immunotherapy has now become a major strategy for the treatment of solid tumors. Five drugs have been approved by Food and Drug Administration (FDA) for metastatic urothelial cancer including three programmed cell-death protein 1 (PD-1) inhibitors and two programmed cell-death ligand 1 (PD-L1) inhibitors in patients who have progressed during or after platinum-based therapy and achieved better treatment (3). Glycyl-tRNA synthetase (GARS) is critical for charging glycine onto cognate tRNA molecules and is required for protein synthesis in the mitochondria and the cytosol. The human GARS protein consists of an N-terminal WHEPTRS domain, a catalytic domain interspersed by three insertion domains and a C-terminal anticodon-binding domain (4). As the research progresses, the study of GARS in tumors is gradually enriched.
Khosh Kish et al. demonstrated that GARS expression is associated with prostate cancer progression and its inhibition decreases migration, and invasion in vitro (5). Wang et al. showed that GARS is implicated in poor survival and immune infiltration of hepatocellular carcinoma (HCC). GARS depletion inhibits HCC cell proliferation and cell cycle progression and promotes apoptosis in vitro. GARS overexpression promotes growth, reduces xenograft apoptosis and enhances CD206+ tumor-associated macrophage (TAM) infiltration in vivo (6). And the prognostic model constructed based on cancer RNA expression also involves GARS (7). The apoptotic activities of naked GARS and GARS-extracellular vesicles (GARS-EVs) had been comparatively evaluated using different cancer cell lines. Of the 15 tested cancer cell lines, GARS and GARS-EVs promoted apoptosis in 9 and 13 cancer cell lines, respectively. Although Xu et al. found that GARS enhances DNA synthesis and promotes cell proliferation in BC cell lines by activating pentose phosphate pathway enzymes (8). However, the studies of GARS in BC tumor microenvironment (TME) and the prognostic value in urine are still lacking. Epithelial-mesenchymal transition (EMT), a biological process by which epithelial cells are transformed into cells with a mesenchymal phenotype through specific programs, plays an important role in cancer progression.
In this study, we investigated the expression of GARS, the mechanism of aberrant GARS expression, the correlation of GARS levels with clinic pathological features, prognosis and immune infiltration, and the signaling pathways and biological processes that GARS may affect in BC. And we explored the expression of GARS in the TME and its effect on EMT. In addition, we examined urinary extracellular vesicles (uEVs) from patients with BC and found that GARS expression in uEVs had the same trend as that was shown in tissues. Our study suggests that GARS could be used as a prognostic and therapeutic target for BC. We present this article in accordance with the MDAR reporting checklist (available at https://tcr.amegroups.com/article/view/10.21037/tcr-23-2148/rc).
Methods
Data acquisition
This study collected BC data from Dataset sets consisting of The Cancer Genome Atlas program (TCGA, n=407), while The TCGA Dataset obtained from University of California Santa Cruz (USCS) Xena (http://xenabroswer.net/hub), included the information of copy number, somatic mutation, DNA methylation. Meanwhile, GSE13507 was used to validate the prognostic value of GARS and all samples for single-cell analysis were obtained from the National Center for Biotechnology Information Gene Expression Omnibus (GEO) Dataset. Single-cell sequencing on tumor-infiltrating cells of four patients with full type cells was performed with GSE211388. The pathologic type of all patients was uroepithelial carcinoma, including three cases of muscle invasive bladder cancer (MIBC) (one with lymph node metastasis) and one case of non-muscle invasive bladder cancer (NMIBC). For the gene expression profiles across all tumor samples and paired normal tissues, the Gene Expression Profiling Interactive Analysis web server (http://gepia.cancer-pku.cn/detail.php) was used.
Analysis of the relationships between GARS, prognosis, methylation, single nucleotide polymorphism (SNP), DNA stemness score (DNAss), RNA stemness score (RNAss) and clinical phenotype
Two indicators—overall survival (OS) and progression-free survival (PFS)—were selected to study the relationship between GARS expression and patient prognosis using the Kaplan-Meier method and Cox regression. Survival curves were analyzed and the plots were produced by the R packages “survival” and “survminer”. RNAss and DNAss of cuproptosis-associated genes were presented using the R packages “limma” and “corrplot”. Spearman’s test was also used during the process.
Differential expression and functional exploration of genes
Samples were divided into high and low expression groups according to the median GARS expression. Analysis of variance was performed using the “limma” package, taking |log fold change (FC)| ≥1, P<0.05. Package “clusterprofiler” was used on all differential genes to perform Gene Ontology (GO) and Kyoto Encyclopedia of Genes and Genomes (KEGG) pathway enrichment analysis. Using Fisher’s exact test, those with P values less than 0.05 were regarded as significant indicators.
For human experiments: the study was conducted in accordance with the Declaration of Helsinki (as revised in 2013). The study was approved by the Medical Ethics Committee of the Second Hospital of Tianjin Medical University, Tianjin, China (KY2023K185).
For animal experiments: experiments were performed under a project license (No. JCPD20221018028) granted by Animal Ethics Committee of the Second Hospital of Tianjin Medical University, Tianjin, China (JCPD20221018028), in compliance with China animal experiments guidelines for the care and use of animals.
Differential analysis of immune cell infiltration, immune function, and immune checkpoint function
The gene set variation analysis (GSVA) package was used for single-sample gene set enrichment analysis (ssGSEA) analysis to obtain a Hallmark gene set score and bulk RNA-seq data were used to analyse GARS-associated immune cell subsets (9). We utilized ssGSEA technique to quantify relative infiltration levels of 28 immunological cells in BC samples. The 28 immunological invading cells had significantly varying expression levels. The “ggplot2” was utilized for visualization after 28 immunological infiltrating cells and hub genes were subjected to Spearman correlation analysis. LAG3, PDL1, PD1, TIGIT, CTLA4, VISTA, BTLA and TIM3 were used as common immune checkpoints to see the relationship between GARS and immunity.
Single-cell data processing
Raw data were converted into a Seurat object by the R package “Seurat” (v 3.1.2) (10). In GSE211388, cells whose percentages of ribosomes or percentage of mitochondria are less than 25 or single cells with less than 200 genes detected were considered low-quality cells and were removed. In order to eliminate potential doublets, single cells with over 5,000 genes detected were also filtered out. 35,388 single cells remained. Samples that did not contain CD45− were removed. Finally, data from four example patients were saved that contained all CD45+ and CD45− cells from the four patients, 23,519 single cells remained.
After quality control, the Seurat object was normalized by the function SCTransform of the “Seurat” package. Since samples were processed and sequenced in batches, their origin tissue was used to remove the potential batch effect. In this progress, the top 2,000 variable genes were used to create potential anchors with the FindIntegration Anchors function of “Seurat”. Subsequently, the Harmony function was used to integrate data and merge a new matrix with 2,000 features, in which potential batch effect was regressed out.
To reduce the dimensionality of the scRNA-Seq dataset, principal component analysis (PCA) was performed on an integrated data matrix. With the elbow plot function of Seurat, the top 30 principal components (PCs) were used to perform the downstream analysis. The main cell clusters were identified with the FindClusters function offered by Seurat, with resolution set as default (GSE211388, res 0.1), and then they were visualized with 2D UMAP Plots (11).
Predicting anti-cancer drug response
To evaluate the ability of the GARS to predict the chemotherapeutic response, the half-maximal inhibitory concentration (IC50) of common chemotherapeutic drugs was first calculated in the BC samples, using the “pRRophetic” package in R software. The Wilcoxon rank test was then used to compare the difference in IC50 between the low- and high-GARS groups.
The Human Protein Atlas (THPA)
Information on GARS protein expression in BC versus normal samples was selected from THPA database (http://www.proteinatlas.org/). The antibody information is HPA019097. Samples’ ids for normal bladder tissue are 3184, 3497 and 3517. Patients’ ids for BC tissue are 1871, 2031, 2053, 2802 and 3265.
Cell lines and lentivirus infection
A normal human bladder uroepithelium cell line (SV-HUC-1) and two BC cell lines (5637 and T24) were obtained from The Cell Bank of Chinese Academy of Sciences (Shanghai, China). SV-HUC-1, T24 and 5637 were cultured in DMEM (Gibco, Carlsbad, CA, USA). All culture media contained 1% penicillin/streptomycin and 10% fetal bovine serum (FBS, Gibco). The cells were incubated in humidified air at 37 ℃ with 5% CO2.
Three human siRNA sequences (siGARS-1, 5'-TGGAAGAAGTTGTTCCGAATGTAAT-3', siGARS-2, 5'-GACAAATTTGCTGACTTCATGGTGA-3') and negative control siRNA (siNC, 5'-GACTTTAGTCGTTCAGTACGAATGA-3') were cloned in pLKO.1-puro vector to endogenously down-regulate GARS. For lentiviral production, 293T cells were transfected with the lentiviral vector along with packaging plasmids using Lipofectamine 2000 (Invitrogen, Shanghai, China). Culture media was collected, pooled and filtered at 48 hours and 72 hours after transfection. Then, the indicated lentivirus was used to infect the BC cell lines.
Western blotting
Proteins were separated from cell lines 5637 and T24 utilizing radio-immunoprecipitation assay (RIPA) lysis buffer. A bicinchoninic acid (BCA) protein kit (Beyotime Biotechnology, Shanghai, China) was then utilized for protein quantifications. Before being transferred to polyvinylidene fluoride (PVDF) membranes, materials were electrophoresed on a 10% SDS polyacrylamide gel (SDS-PAGE) (Merck Millipore, Billerica, MA, USA). Blocking in 5% nonfat milk and tris-buffered saline buffer (TBST), then membranes were treated with GARS (ab125008, Abcam, Shanghai, China), and β-actin (ab8227, Abcam) overnight at 4 ℃, after that incubated with HRP-conjugated secondary antibody (Sangon, Shanghai, China) for 1 h at 37 ℃. Enhanced chemiluminescence revealed protein bands (ECL; Beyotime) followed by analysis utilizing ImageJ software.
Quantitative real-time polymerase chain reaction (QRT-PCR)
Following manufacturer instructions, total RNA was isolated utilizing TRIzol reagent (Invitrogen), dissolved in RNA-free H2O, and frozen at −80 ℃. Each 1 µg RNA sample was utilized to synthesize cDNA utilizing Reverse Transcriptase Kit (Thermo, Waltham, MA, USA). Afterward, qPCR utilizing an SYBR Green PCR kit (Thermo) was carried out on a real-time detector (ABI, Carlsbad, MA, USA). GARS had the following primer sequences:primer F, 5'-AGTGCTGCTTAGAGGTGCTC-3', primer R, 5'-CCTGGGAGTAATCACAATGC 5−3'. Expression data were computed utilizing the 2-ΔΔCt technique and normalization was performed using GAPDH as an internal reference for relative expression level control. Tables 1,2 illustrate the patients information for PCR.
Table 1
ID | Type | Gender | Age (years) | Grade | T | N | M |
---|---|---|---|---|---|---|---|
594042 | BC tissue | Male | 71 | Low | 1 | 0 | 0 |
597966 | BC tissue | Male | 69 | High | 1 | 0 | 0 |
597685 | BC tissue | Female | 73 | High | 2 | 2 | 0 |
594042 | Paracancerous tissue | Male | 71 | Low | 1 | 0 | 0 |
597966 | Paracancerous tissue | Male | 69 | High | 1 | 0 | 0 |
597685 | Paracancerous tissue | Female | 73 | High | 2 | 2 | 0 |
All three pairs of samples were obtained from patients after total cystectomy, none of whom had received preoperative drug therapy. Externally normal mucosa was taken as paracancerous tissue for analysis. BC, bladder cancer.
Table 2
ID | Type | Gender | Age (years) | Grade | T | N | M | Group |
---|---|---|---|---|---|---|---|---|
598669 | BC | Male | 55 | High | 2 | 0 | 0 | High GARS |
538760 | BC | Female | 75 | High | 3 | 0 | 0 | High GARS |
595913 | BC | Male | 81 | High | 3 | 1 | 0 | High GARS |
595068 | BC | Female | 81 | High | 2 | 0 | 0 | High GARS |
594661 | BC | Male | 78 | High | 1 | 0 | 0 | High GARS |
536866 | BC | Male | 69 | High | 2 | 1 | 0 | High GARS |
579977 | BC | Male | 51 | High | 4 | 0 | 0 | Low GARS |
592726 | BC | Male | 75 | High | 3 | 0 | 0 | Low GARS |
568725 | BC | Male | 76 | High | 2 | 0 | 0 | Low GARS |
591632 | BC | Male | 81 | High | 1 | 0 | 0 | Low GARS |
587748 | BC | Male | 43 | High | 2 | 0 | 0 | Low GARS |
598458 | BC | Male | 87 | High | 1 | 0 | 0 | Low GARS |
697669 | ccRCC | Male | 55 | Low | 1b | 0 | 0 | High GARS |
638760 | ccRCC | Female | 75 | High | 2a | 0 | 0 | High GARS |
694913 | ccRCC | Male | 81 | High | 3a | 1 | 0 | High GARS |
695068 | ccRCC | Female | 81 | Low | 2a | 0 | 0 | High GARS |
696661 | ccRCC | Male | 78 | Low | 1a | 0 | 0 | High GARS |
636866 | ccRCC | Male | 69 | Low | 2b | 1 | 0 | High GARS |
692726 | ccRCC | Male | 75 | Low | 3a | 0 | 0 | Low GARS |
663725 | ccRCC | Male | 76 | Low | 2b | 0 | 0 | Low GARS |
691532 | ccRCC | Male | 81 | High | 1b | 0 | 0 | Low GARS |
686748 | ccRCC | Male | 43 | Low | 2a | 0 | 0 | Low GARS |
699458 | ccRCC | Male | 87 | High | 1b | 0 | 0 | Low GARS |
697566 | ccRCC | Male | 69 | Low | 1b | 0 | 0 | Low GARS |
All samples were divided into high and low groups according to the expression of GARS in tissues, and EVs were extracted from patients’ urine and the expression level of GARS was measured. EV, extracellular vesicle; BC, bladder cancer; ccRCC, clear cell renal carcinoma.
Extracellular vesicle isolation
Cells and other debris were separated out of the collected urine and centrifuged at 1,000 L for 15 min. The Exoquick extracellular vesicle precipitation solution (System Biosciences, Palo Alto, CA, USA) was utilized for the isolation of extracellular vesicles. Table 2 illustrates the patients information for urine extracellular vesicle extraction.
Cell Counting Kit-8 (CCK-8)
CCK-8 (APExBIO, Houston, TX, USA) was utilized to assess cell proliferation. About 3×103 target cells were planted into each well of a 96-well culture plate and kept at 37 ℃ for one night. Cells were exposed to CCK-8 (10 µL/well) and incubated for 2 h after being transfected at 24, 48, 72, and 96 h. Then, at 450 nm, the optical densities of all the wells were measured.
Wound healing assays
Cells were inoculated in six-well plates, and after 24 h, 200 µL pipetting head was used to scratch the cells in the plate, and the serum-free medium was changed. By running a plastic tip across single-layered cells, wounds were created. The time of wound infliction was considered as 0 h, and the wounds were photographed under a microscope with an attached digital camera after 0, 12, and 24 h. ImageJ 1.48 v quantified the percentage of migrating cell-covered areas. Triplicates of all experiments were done to ensure accuracy.
Transwell invasion assay
Transwell chambers have been used to conduct invasion tests with and without Matrigel (Corning, Kennebunk, USA). While 5×104 cells were planted in 300 µL of serum-free media through upper chamber, 700 µL of 10% FBS-media was added in lower chamber and let to incubate for 24 h. Following a 20-minute fixation in 4% formaldehyde, we gently scraped off the remaining cells in the upper chamber and gave the cells a quick rinse in phosphate buffered solution (PBS). After staining the cells for 10 min with 0.1% crystal violet in 1 mL/well, the wells were rinsed triplet with PBS to void any residual stain. Through the use of a 200× microscope, the total number of migrating or invading cells may be determined.
Tumor xenograft experiments
We divided 10 mice into two groups: a control group and a knockdown group. Subcutaneously implant tumor cells into the left abdomen of nude mice to establish a xenograft model. Monitor the size of the tumor every 5 days. Tumor volume = (length × Width2)/2.
Statistical analysis
R 4.1.2 and GraphPad Prism 9.3.0 was utilized to conduct all statistical analyses. The Kolmogorov-Smirnov test determined whether to apply a parametric or non-parametric rank-based analysis. The Fisher exact and Wilcoxon rank-sum tests were applied to categorical and continuous variables, respectively, to test hypotheses. Survival analysis estimation was conducted using Kaplan-Meier and Cox regression multivariate analysis, and group comparisons were made utilizing a log-rank test. A cluster profiler package was utilized to determine GO enrichment terms and KEGG pathways. Two groups of data satisfying normal distribution were tested by t-test otherwise using Mann-Whitney U test. P value <0.05 was regarded significant, for all statistical analysis. *, P<0.05; **, P<0.01; ***, P<0.001.
The affiliation shown in the animal experiment ethical approval being inconsistent with the authors’ affiliation was mainly due to the change of the corresponding author’s unit from the Second Hospital of Tianjin Medical University to the General Hospital of Tianjin Medical University.
Results
GARS is highly expressed in BC and is associated with poor prognosis
With the assistance of the shiny online platform, which incorporates sequencing data from TCGA and the GTEx database, we first assessed GARS expression in 33 different cancer types. Interestingly, 32 of 33 cancer types exhibited considerably greater levels of GARS expression in tumor tissue, whereas in the sarcomatoid carcinoma (SARC), the GARS expression levels of the tumor and normal tissues were similar. GARS was highly expressed in most tumor tissues, supporting its oncogene function (Figure 1A). We further validated that GARS was markedly overexpressed in BC compared to corresponding normal tissue using the TCGA database (Figure 1B).
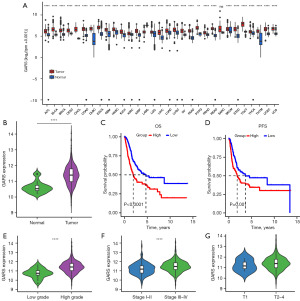
GARS is associated with poor prognosis
Kaplan-Meier survival curves discovered that patients in the GARS-high group had a poor prognosis, with high GARS expression leading to shorter OS and PFS in BC patients (Figure 1C,1D). Additionally, we examined the relationship between GARS expression and the clinical characteristics of BC. Not surprisingly, GARS expression showed a significant positive correlation with tumor grade and stage (Figure 1E-1G). The above findings implied that GARS was a trustworthy biomarker and was most likely implicated in the development of BC. We then used univariate and multivariate Cox regression to investigate if the GARS expression was an independent predictor of the BC patient’s prognosis. The analysis indicated that GARS was a prognostic indicator independent of certain other clinical characteristics (Figure 2A,2B). Moreover, receiver operating characteristic (ROC) curve and decision curve analysis (DCA) curve depict that GARS expression has superior prognostic prediction ability for BC patients compared to traditional tumor staging (Figure 2C,2D). Similarly GARS was validated as BC-independent post factor in GSE13507 (Figure S1).
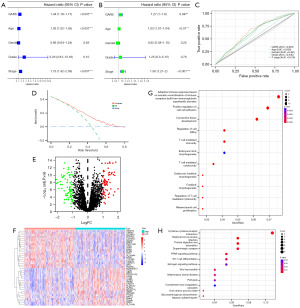
Functional analysis of GARS
To further investigate the biological role of GARS in BC, we separated BC patients into GARS-high and GARS-low groups based on the median value of GARS expression, and then we compared the differentially expressed genes (DEGs) between the two groups. The genes that differ most between the two groups are displayed on the heatmap (Figure 2E,2F).
We noticed that some biomarkers were significantly highly expressed in the GARS-high group. For example, Shh signaling promotes tumorigenicity and stem cell generation by stimulating EMT in BC (12). We further carried out functional enrichment analysis on DEGs between GARS-high and GARS-low groups. GO analysis revealed the DEGs enriched in immune-related pathways (Figure 2G), like adaptive immune response based on somatic recombination of immune receptor built from immunoglobulin superfamily domains, regulation of cell killing, T cell mediated immunity and regulation of T cell mediated cytotoxicity, were significantly enriched in these DEGs. Moreover, KEGG analysis uncovered that these DEGs were significantly enriched in TH17 differentiation and cytokine-cytokine receptor interaction. Thus, GARS predominantly regulates immune cell infiltration in BC (Figure 2H). Additionally, these DEGs displayed enrichment of peroxisome proliferators-activated receptors (PPAR) signaling pathways, suggesting that GARS may be involved in the regulation of tumor lipid metabolism.
GARS is linked to tumor immune infiltration
We further analyzed the correlation between GARS expression and immune cell infiltration scores. The findings revealed that the majority of immune cell infiltration was positively linked with GARS expression, including active B cell, activated CD4 T cell, activated CD8 T cell, immature B cell, macrophage, effector memory CD8 T cell, natural killer (NK) cell and Treg cells, etc. (Figure 3).
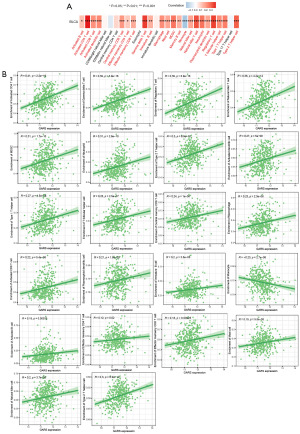
However, when we calculated the degree of immune checkpoint expression, we found that many immune checkpoints, including PD1, PDL1, TIGIT, TIM3 and CTLA4, were significantly overexpressed in GARS-high group (Figure 4A). Despite having a high level of immune infiltration, patients in the GARS-high group may have inadequate anti-tumor status because of the high expression of immunological checkpoints, according to our observation. Patients in the GARS-high group may achieve a high response rate during immunotherapy.
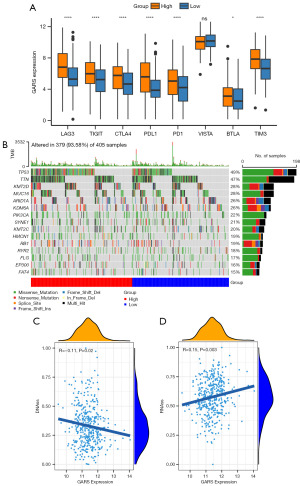
The mutation landscape of GARS and tumor stemness analysis
We assessed the somatic mutation rate in the GARS-high and GARS-low groups due to the oncogenic role of GARS in BC. Somatic mutations occurred in 379 of 405 patients in BC. Patients in the GARS-high group had considerably higher rates of P53 and Titin (TTN) mutations than those in the GARS-low group (Figure 4B). TP53 is a tumor suppressor gene that is mutated in more than half of all human cancers. Tumors with P53 mutations progress more rapidly and have a poorer prognosis (13). P53 mutations are more frequent in GARS-high patients, suggesting that GARS is connected to the P53 pathway. We also investigated the connection between the GARS expression and the tumor stem cell score.
Growing evidence indicates that increased expression of stemness-related biomarkers in tumor cells is highly correlated with drug resistance, cancer recurrence, and tumor proliferation (14). Then, we assessed the correlations of the DNAss and RNAss with GARS (Figure 4C,4D). The results indicated that GARS was positively correlated with RNAss. Finally, we calculated the concentration causing a 50% reduction growth (IC50) of drugs to determine which drugs have significantly different sensitivities between GARS-high and GARS-low groups. Patients in the GARS-high group had a higher sensitivity to cisplatin, epirubicin, vinblastine, and paclitaxel (Figure 5).
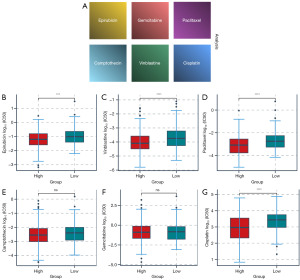
GARS is enriched in myofibroblasts in the TME and promotes the transition of NMIBC to MIBC by participating in EMT.
More and more studies have demonstrated that the microenvironment plays an important role in tumor development (15-18). To further investigate the biological functions of GARS, we categorized 23,519 cells into 9 clusters, which were classified as T/NK cells, epithelial cells, macrophages, myofibroblasts, plasma cells, B cells and endothelial cells according to the cell markers shown in Table 3 (Figure 6A-6C). Myofibroblast transformation refers to a characteristic phenotypic transformation of local fibroblasts, epithelial cells, or bone marrow mesenchymal stem cells migrating to the site of injury under the action of environmental factors of injury, and the special pathologic mesenchymal cells that undergo this transformation are called myofibroblasts (19). Myofibroblasts are seldom found in adult normal tissues, and they are mainly seen in tumors, tissue repair, and other pathological states, which have an important influence on the development of tumor cells, and are a key evolutionary process during tumor progression (20). In this study, we found that GARS was enriched in myofibroblasts (Figure 7A), in addition to epithelial cells, in microenvironment. In the previous enrichment analysis, we observed that GARS and its related genes were functionally enriched in the positive regulation of cell-cell adhesion (Figure 2G). Following these findings, we explored the relationship between GARS and five EMT-related genes and were surprised to find that GARS was positively correlated with the expression of CCL2, EGR1, EIF5A, NUAK1 and SOD2 (Figure 7B). Furthermore, we mapped the myofibroblast differentiation trajectory by utilizing the Monocle2 R package (Figure 7C). Notably, the trajectory clearly demonstrated the progression of BC from non-muscle invasion to muscle invasion, and during the progression from NMIBC to MIBC (Figure 7D), we could clearly see the gradual increase in the expression of CCL2, EGR1, EIF5A, NUAK1 and SOD2 (Figure 7E), and the expression of the above five genes was higher in MIBC than in NMIBC (Figure 7F). This suggested that GARS may have influenced the process of EMT by participating in the biological processes of these five genes, leading to the progression of NMIBC toward MIBC.
Table 3
Cell | Marker |
---|---|
T/NK cell | CD2, CD3D, CD3E, CD3G, CD4, CD8A, CD8B, NKG7, CD56, GNLY |
Myofibroblasts | ATCA2, TAGLN, PDGFRA, PDGFRB |
Epithelial cell | EPCAM, KRT8, KRT1, KRT10 |
B cell | CD19, MS4A1, CD79B, CD79A |
Macrophages | AIF1, CSF1R, TYROBP, FCER1G, LYZ, CST3, PTPRC, MS4A6A, CD68, CD1E, IL3RA, LAMP3 |
plasma cell | TNFRSF17, CD38, IGHG1, IGHG4, IGHG, MZB1 |
Endothelial cell | CDH5, ENG, CLDN5, CD31, PECAM1 |
NK, natural killer.
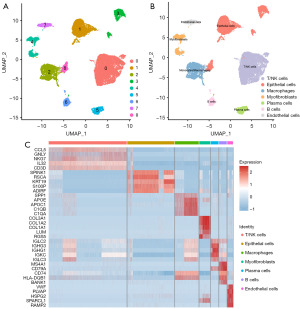
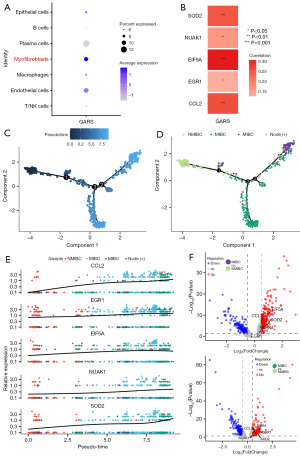
GARS enhances proliferation and invasion of BC cells
To further explore the biological function of GARS in BC, we used 2 GARS-specific shRNA to make GARS knockdown cells in T24 and 5637 cell lines (Figure 8A). The expression of GARS was significantly decreased in T24 and 5637 cells both at mRNA and protein level. CCK-8 assay showed that silencing of GARS using siGARS-1 and siGARS-2 significantly reduced the viability of T24 and 5637 cells (Figure 8B). Wound healing assay and Transwell results showed that all the proliferation and invasion ability of T24 and 5637 cells were decreased after silencing GARS (Figure 8C,8D). In addition, Xenograft tumours from respective groups were depicted following injection with siGARS T24 cells and T24 cells, confirmed the tumorigenic effects of GARS in vivo (Figure 8E).
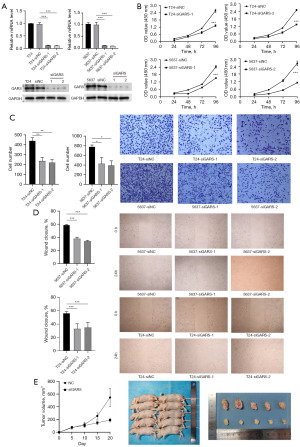
Expression of GARS in urological tumors tissues and uEVs
Kidney is known to contribute a lot uEVs to urine, more than bladder in some donor groups (21). To further explore the prognostic role of GARS plays in BC patients, we added clear cell renal carcinoma (ccRCC) patients as reference. We characterized extracellular vesicles isolated from the urine of BC patients, ccRCC patients and healthy individuals (Figure 9A). The result of RT-PCR showed there was no significant difference between the expression of GARS in the uEVs of ccRCC patients with high expression of GARS and the uEV of ccRCC patients with low expression of GARS (Figure 9B). We performed RT-PCR to detect the expression of GARS in the uEV of BC patients. We were surprised to find that GARS expression was significantly higher in extracellular vesicles of BC patients compared with normal subjects, and the level of GARS expression in uEVs was also high in the GARS high expression group (Figure 9C). GARS protein expression information was obtained from THPA, and the results showed that GARS remained highly expressed at the protein level, in BC (Figure 9D).
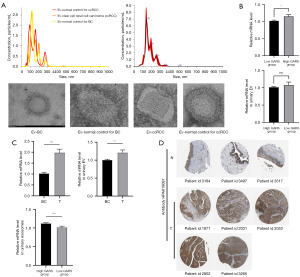
Discussion
BC is the most common and aggressive cancer of the urinary system (22). The standard treatment for MIBC is still early radical cystectomy; however, the 5-year cancer-specific mortality rate for individuals with BC has not decreased significantly, highlighting the surgical approach’s shortcomings (23). First-line chemotherapy regimens have relatively high objective response rates of 36–65% for individuals with advanced BC. However, their 5-year OS is only 13–15%, indicating that chemoresistance will emerge in almost all patients as the cancer advances (24). Patients with advanced cancer who do not respond to first-line chemotherapy have a very dismal prognosis, and there are no effective treatment choices (25). With the advent of immune checkpoint inhibitors and the availability of targeted medicines in recent years, BC treatment has seen considerable advancements. Nevertheless, only PD-1/PD-L1 inhibitors, FGFR3 inhibitors, and antibody-drug conjugates (ADCs) are currently approved by the FDA for the treatment of advanced/metastatic uroepithelial carcinoma in patients who are platinum-refractory or platinum-ineligible (26). We still lack particular therapeutic targets for BC despite the fact that high-throughput sequencing has increased the finding of molecular targets and enhanced our knowledge of their cellular effects. Hence, in order to develop precision medicine, researchers need to dedicate themselves to investigating the molecular mechanisms of BC and identifying new targets for its therapy. Previous studies have demonstrated that GARS is a potential oncogene that is associated with poor OS in a variety of cancers (27). For instance, by regulating the mTOR signaling system, GARS accelerates the proliferation, cell cycle, migration, and invasion of breast cancer cells (28). Importantly, Chen et al. demonstrated that GARS is a reliable urinary biomarker in uroepithelial carcinoma (29). In prostate cancer cell lines, GARS knockdown reduces cell motility and invasion and causes S-phase cell arrest and early apoptotic signals (5). However, the biological function and biomarker role of GARS in BC have not been studied. In our work, we identified that GARS was a reliable biomarker for BC patients and could accurately predict the BC patient’s prognosis. Furthermore, GARS participated in tumor immune infiltration and was associated with immunotherapy efficacy. In order to confirm the role of GARS in modulating tumour cell fate, GARS was knocked down in BC cell lines. The results demonstrated GARS significantly promoted cell proliferation and invasion in vitro. In addition, found that GARS was highly expressed in the uEVs of BC and the trend was consistent with the trend of GARS expression in tissues.
First, we evaluated the GARS’s mRNA expression value in 33 cancers using TCGA and GTEx databases. GARS was highly expressed in almost all cancer tissues, implying that it may play a role in the development of multiple cancers. Next, we demonstrated that GARS was also highly expressed in BC tissues using the TCGA database, suggesting a promising future for GARS in BC diagnosis. Additionally, OS and PFS survival analyses all revealed that GARS was significantly associated with BC patient’s prognosis. Elevated GARS expression resulted in poorer prognosis. To some extent, tumor grading and clinical staging can show how tumors progress. Our results showed that GARS expression was positively correlated with grade and stage of BC patients. According to the above findings, GARS might have an important role in the prognosis of BC patients, and it plays a role in the TME.
Then, compared the DEGs between the GARS-high and GARS-low groups and found that these DEGs were mainly enriched in immune-related pathways, including adaptive immune response based on somatic recombination of immune receptor built from immunoglobulin superfamily domains, regulation of cell killing, T cell mediated immunity and regulation of T cell mediated cytotoxicity, TH17 cell differentiation and cytokine-cytokine receptor interaction. Furthermore, by examining the somatic mutations in BC patients, patients in the GARS-high category had significantly greater rates of P53 and TTN mutations than those in the GARS-low subgroup. Patients with high GARS had a higher prevalence of P53 mutations, indicating that GARS was involved in the P53 pathway.
Meanwhile, single cell analysis confirmed that GARS was not only expressed in epithelial cells but also significantly expressed in macrophages and T cells. The results presented above imply that GARS is involved in controlling the tumor immune microenvironment. The TME refers to the complex and heterogeneous multicellular environment in which tumors grow. TME includes stromal cells, numerous cytokines, and immune cells such as T and B lymphocytes, TAM, NK cells, and others. It is now commonly acknowledged that TME plays a crucial role in dynamically controlling the development of cancer and affecting treatment outcomes, and therapeutic strategies targeting the TME have emerged as a promising approach to cancer treatment (30). In addition, the TME is associated with poor prognosis and treatment resistance, including immunotherapy (31). The clinical potential of immunotherapy may be significantly limited by immunosuppression microenvironment, according to a growing body of research (32). BC is a disease with a unique TME consisting of immune cells, cytokines, and a rich pattern of immune gene expression, making it an ideal candidate for immunotherapy treatment (33). We discovered the majority of immune cell infiltration was positively linked with GARS expression, including active B cell, activated CD4 T cell, activated CD8 T cell, immature B cell, macrophage, effector memory CD8 T cell, NK cell and Treg cells, etc. In contrast, high GARS expression may result in less infiltration of monocytes. The key immune cells for anti-tumor immunity are NK cells and activated CD8 T cells, whereas Treg cells produce a tumor immunosuppressive environment. GARS was significantly associated with multiple immune cell infiltrates, suggesting a complex role in the immune microenvironment. Notably, we found that most of immune check points, including PD1, PDL1, TIGIT, TIM3 and CTLA4, were significantly overexpressed in GARS-high group. Due to the strong expression of immunological checkpoints, patients in the GARS-high group may not have enough anti-tumor status while having a high level of immune infiltration. Patients in the GARS-high category may receive an immunotherapy with a high response rate. In HCC, the investigators confirmed that GARS overexpression promoted tumor growth and enhanced infiltration of CD206+ TAMs in vivo, which was consistent with the results of our study (6).
In addition, we further explored the role of GARS in the TME using GSE211388 and we found that in addition to epithelial cells, GARS was enriched in myofibroblasts. Myofibroblasts have been found to have the following roles during tumor progression: (I) maintaining tumor cells, secreting a large number of soluble cytokines to promote tumor cell proliferation, synthesizing a large amount of extracellular matrix, and constituting a metabolic system suitable for tumor cell growth (34); (II) providing morphological scaffolds, assembling tumor cells, constructing tumor tissues, and promoting spatial development of tumor tissues (35); (III) enabling tumor cells to shed and gain invasive migration through epithelial mesenchymal transformation (36); (IV) secretion of vascular endothelial growth factor, promoting the proliferation of microvessels and microlymphatic vessels, increasing the permeability of blood vessels and lymphatic vessels, ensuring the nutrient supply of tumor cells and assisting the entry of tumor cells into the vascular system etc. (37).
As for clinical BC samples, in NMIBC, E-cadherin expression was slightly diminished, and the expression of both SOX2 and NANOG was negligible. In contrast, in MIBC, E-cadherin expression was highly and heterogeneously diminished, while the expression of both SOX2 and NANOG was increased. This signifies an upward trend in EMT levels during the progression of NMIBC to MIBC (38).
We identified five genes (CCL2, EGR1, EIF5A, NUAK1 and SOD2) positively associated with EMT among the differential genes in the progression of NMIBC toward MIBC. In triple-negative breast cancer, M2-like TAMs promote EMT in triple negative breast cancer (TNBC) through activation of the CCL2/AKT/β-catenin signaling pathway (39). Meanwhile, CCL2/CCR2 activation can promote EMT in prostate cancer (40). Wang et al. demonstrated EGR1 induces EMT via a P300/SNAI2 pathway in pancreatic cancer (41). In contrast, in gastric cancer, downregulation of EGR1 leads to suppression of EMT levels (42). Besides, EGR1-CCL2 feedback loop might exert critical roles on EMT and angiogenesis of chemoresistant gastric cancer (43). Xu et al. showed that down-regulation of EIF5A-2 prevents EMT in non-small-cell lung cancer cells (44). In non-small-cell lung cancer, EIF5A-2 regulates EMT, which causes tumor invasion and metastatic disease. When it comes to NUAK1, Guan et al. indicated that the knockdown of NUAK1 may inhibit prostate cancer cells EMT, migration and invasion (45). And increased expression of NUAK1 in HCC cells through the ceRNA network enhances the ability of mesenchymal transition of epithelial cells, which promotes HCC migration and invasion (46). As for SOD2, RUNX2 promotes EMT and bone metastasis in breast cancer by inhibiting the expression of PPARα and SOD2 (47). In addition to this, we found a positive correlation between GARS and the expression of these five genes in database. In order to investigate the role of GARS in myofibroblasts during the progression of BC from NMIBC to MIBC, we did a proposed pseudotime analysis to simulate the progression from NMIBC to MIBC. We were surprised to find that the expression of the five EMT-related genes gradually increased as the BC progressed, suggesting that GARS regulates EMT in myofibroblasts during the progression from NMIBC to MIBC. In conclusion, GARS plays a complex role in regulating the tumor immune microenvironment.
Beyond what has been mentioned above, we performed GARS knocked down in BC cell lines. The results showed that GARS significantly promoted cell proliferation and invasion in vitro and vivo. In addition, found that GARS had higher expression levels in uEVs of BC patients. extracellular vesicles are nanoscale vesicles derived from endocytosis, formed by fusion of multivesicular bodies with membranes and secreted into the extracellular matrix or body fluids. Extracellular vesicles play an important role in the diagnosis and prognostic markers of the disease (48). To further investigate the significance of GARS in uEVs, we divided 12 patients into two groups based on median GARS expression level and found that patients with high GARS also had higher expression of GARS in uEVs. This suggests that we can measure GARS in uEVs instead of tissues to predict the prognosis of patients.
Conclusions
In sum, bioinformatics analysis was performed to confirm that GARS is a potential biomarker and oncogene for BC that can accurately predict the cancer patient’s prognosis. Furthermore, we identified that GARS is mainly involved in regulating immune cell infiltration in BC and investigated its relationship with immune checkpoints. Besides, also found that GARS is enriched in myofibroblasts in the TME in BC and affects the progression from NMIBC to MIBC by participating in the regulation of EMT. In addition, GARS promotes the proliferation and invasion of BC, and its detection in uEVs may replace the detection in tissues to better assess the prognosis of patients. This is similar to the findings of Chen et al. (29). In conclusion, GARS may be a promising biomarker and therapeutic target for immunotherapy in BC.
Acknowledgments
Funding: This study was supported by
Footnote
Reporting Checklist: The authors have completed the MADR reporting checklist. Available at https://tcr.amegroups.com/article/view/10.21037/tcr-23-2148/rc
Data Sharing Statement: Available at https://tcr.amegroups.com/article/view/10.21037/tcr-23-2148/dss
Peer Review File: Available at https://tcr.amegroups.com/article/view/10.21037/tcr-23-2148/prf
Conflicts of Interest: All authors have completed the ICMJE uniform disclosure form (available at https://tcr.amegroups.com/article/view/10.21037/tcr-23-2148/coif). The authors have no conflicts of interest to declare.
Ethical Statement: The authors are accountable for all aspects of the work in ensuring that questions related to the accuracy or integrity of any part of the work are appropriately investigated and resolved. For human experiments: The study was conducted in accordance with the Declaration of Helsinki (as revised in 2013). The study was approved by the Medical Ethics Committee of the Second Hospital of Tianjin Medical University, Tianjin, China (KY2023K185). For animal experiments: Experiments were performed under a project license (No. JCPD20221018028) granted by Animal Ethics Committee of the Second Hospital of Tianjin Medical University, Tianjin, China (JCPD20221018028), in compliance with China animal experiments guidelines for the care and use of animals.
Open Access Statement: This is an Open Access article distributed in accordance with the Creative Commons Attribution-NonCommercial-NoDerivs 4.0 International License (CC BY-NC-ND 4.0), which permits the non-commercial replication and distribution of the article with the strict proviso that no changes or edits are made and the original work is properly cited (including links to both the formal publication through the relevant DOI and the license). See: https://creativecommons.org/licenses/by-nc-nd/4.0/.
References
- Bray F, Ferlay J, Soerjomataram I, et al. Global cancer statistics 2018: GLOBOCAN estimates of incidence and mortality worldwide for 36 cancers in 185 countries. CA Cancer J Clin 2018;68:394-424. [Crossref] [PubMed]
- Lenis AT, Lec PM, Chamie K, et al. Bladder Cancer: A Review. JAMA 2020;324:1980-91. [Crossref] [PubMed]
- Konala VM, Adapa S, Aronow WS. Immunotherapy in Bladder Cancer. Am J Ther 2022;29:e334-7. [Crossref] [PubMed]
- Markovitz R, Ghosh R, Kuo ME, et al. GARS-related disease in infantile spinal muscular atrophy: Implications for diagnosis and treatment. Am J Med Genet A 2020;182:1167-76. [Crossref] [PubMed]
- Khosh Kish E, Gamallat Y, Choudhry M, et al. Glycyl-tRNA Synthetase (GARS) Expression Is Associated with Prostate Cancer Progression and Its Inhibition Decreases Migration, and Invasion In Vitro. Int J Mol Sci 2023;24:4260. [Crossref] [PubMed]
- Wang J, Yang B, Wang D, et al. GARS is implicated in poor survival and immune infiltration of hepatocellular carcinoma. Cell Signal 2022;94:110302. [Crossref] [PubMed]
- Zhang X, Dong W, Zhang J, et al. A Novel Mitochondrial-Related Nuclear Gene Signature Predicts Overall Survival of Lung Adenocarcinoma Patients. Front Cell Dev Biol 2021;9:740487. [Crossref] [PubMed]
- Xu N, Yao Z, Shang G, et al. Integrated proteogenomic characterization of urothelial carcinoma of the bladder. J Hematol Oncol 2022;15:76. [Crossref] [PubMed]
- Subramanian A, Tamayo P, Mootha VK, et al. Gene set enrichment analysis: a knowledge-based approach for interpreting genome-wide expression profiles. Proc Natl Acad Sci U S A 2005;102:15545-50. [Crossref] [PubMed]
- Vento-Tormo R, Efremova M, Botting RA, et al. Single-cell reconstruction of the early maternal-fetal interface in humans. Nature 2018;563:347-53. [Crossref] [PubMed]
- Sun H, Huang Q, Huang M, et al. Human CD96 Correlates to Natural Killer Cell Exhaustion and Predicts the Prognosis of Human Hepatocellular Carcinoma. Hepatology 2019;70:168-83. [Crossref] [PubMed]
- Islam SS, Mokhtari RB, Noman AS, et al. Sonic hedgehog (Shh) signaling promotes tumorigenicity and stemness via activation of epithelial-to-mesenchymal transition (EMT) in bladder cancer. Mol Carcinog 2016;55:537-51. [Crossref] [PubMed]
- Bykov VJN, Eriksson SE, Bianchi J, et al. Targeting mutant p53 for efficient cancer therapy. Nat Rev Cancer 2018;18:89-102. [Crossref] [PubMed]
- Walcher L, Kistenmacher AK, Suo H, et al. Cancer Stem Cells-Origins and Biomarkers: Perspectives for Targeted Personalized Therapies. Front Immunol 2020;11:1280. [Crossref] [PubMed]
- Xiao Y, Yu D. Tumor microenvironment as a therapeutic target in cancer. Pharmacol Ther 2021;221:107753. [Crossref] [PubMed]
- Bader JE, Voss K, Rathmell JC. Targeting Metabolism to Improve the Tumor Microenvironment for Cancer Immunotherapy. Mol Cell 2020;78:1019-33. [Crossref] [PubMed]
- Ho WJ, Jaffee EM, Zheng L. The tumour microenvironment in pancreatic cancer - clinical challenges and opportunities. Nat Rev Clin Oncol 2020;17:527-40. [Crossref] [PubMed]
- de Visser KE, Joyce JA. The evolving tumor microenvironment: From cancer initiation to metastatic outgrowth. Cancer Cell 2023;41:374-403. [Crossref] [PubMed]
- Xiao X, Huang C, Zhao C, et al. Regulation of myofibroblast differentiation by miR-424 during epithelial-to-mesenchymal transition. Arch Biochem Biophys 2015;566:49-57. [Crossref] [PubMed]
- O'Connor JW, Gomez EW. Cell adhesion and shape regulate TGF-beta1-induced epithelial-myofibroblast transition via MRTF-A signaling. PLoS One 2013;8:e83188. [Crossref] [PubMed]
- Blijdorp CJ, Hartjes TA, Wei KY, et al. Nephron mass determines the excretion rate of urinary extracellular vesicles. J Extracell Vesicles 2022;11:e12181. [Crossref] [PubMed]
- Antoni S, Ferlay J, Soerjomataram I, et al. Bladder Cancer Incidence and Mortality: A Global Overview and Recent Trends. Eur Urol 2017;71:96-108. [Crossref] [PubMed]
- Vlachostergios PJ, Faltas BM. Treatment resistance in urothelial carcinoma: an evolutionary perspective. Nat Rev Clin Oncol 2018;15:495-509. [Crossref] [PubMed]
- Liu S, Chen X, Lin T. Emerging strategies for the improvement of chemotherapy in bladder cancer: Current knowledge and future perspectives. J Adv Res 2022;39:187-202. [Crossref] [PubMed]
- Yafi FA, North S, Kassouf W. First- and second-line therapy for metastatic urothelial carcinoma of the bladder. Curr Oncol 2011;18:e25-34. [Crossref] [PubMed]
- Morales-Barrera R, Suárez C, González M, et al. The future of bladder cancer therapy: Optimizing the inhibition of the fibroblast growth factor receptor. Cancer Treat Rev 2020;86:102000. [Crossref] [PubMed]
- Jiang Y, Ouyang W, Zhang C, et al. Prognosis and Immunotherapy Response With a Novel Golgi Apparatus Signature-Based Formula in Lung Adenocarcinoma. Front Cell Dev Biol 2022;9:817085. [Crossref] [PubMed]
- Li X, Sun H, Liu Q, et al. Conjoint analysis of circulating tumor cells and solid tumors for exploring potential prognostic markers and constructing a robust novel predictive signature for breast cancer. Cancer Cell Int 2021;21:708. [Crossref] [PubMed]
- Chen CJ, Chou CY, Shu KH, et al. Discovery of Novel Protein Biomarkers in Urine for Diagnosis of Urothelial Cancer Using iTRAQ Proteomics. J Proteome Res 2021;20:2953-63. [Crossref] [PubMed]
- Bejarano L, Jordāo MJC, Joyce JA. Therapeutic Targeting of the Tumor Microenvironment. Cancer Discov 2021;11:933-59. [Crossref] [PubMed]
- Binnewies M, Roberts EW, Kersten K, et al. Understanding the tumor immune microenvironment (TIME) for effective therapy. Nat Med 2018;24:541-50. [Crossref] [PubMed]
- Xiang X, Wang J, Lu D, et al. Targeting tumor-associated macrophages to synergize tumor immunotherapy. Signal Transduct Target Ther 2021;6:75. [Crossref] [PubMed]
- Nair SS, Weil R, Dovey Z, et al. The Tumor Microenvironment and Immunotherapy in Prostate and Bladder Cancer. Urol Clin North Am 2020;47:e17-54. [Crossref] [PubMed]
- Castells M, Milhas D, Gandy C, et al. Microenvironment mesenchymal cells protect ovarian cancer cell lines from apoptosis by inhibiting XIAP inactivation. Cell Death Dis 2013;4:e887. [Crossref] [PubMed]
- Hirokawa Y, Yip KH, Tan CW, et al. Colonic myofibroblast cell line stimulates colonoid formation. Am J Physiol Gastrointest Liver Physiol 2014;306:G547-56. [Crossref] [PubMed]
- Liu C, Chen F, Han X, et al. Role of TGF-β1/p38 MAPK pathway in hepatitis B virus-induced tubular epithelial-myofibroblast transdifferentiation. Int J Clin Exp Pathol 2014;7:7923-30. [PubMed]
- Cho JA, Park H, Lim EH, et al. Exosomes from breast cancer cells can convert adipose tissue-derived mesenchymal stem cells into myofibroblast-like cells. Int J Oncol 2012;40:130-8. [PubMed]
- Migita T, Ueda A, Ohishi T, et al. Epithelial-mesenchymal transition promotes SOX2 and NANOG expression in bladder cancer. Lab Invest 2017;97:567-76. [Crossref] [PubMed]
- Chen X, Yang M, Yin J, et al. Tumor-associated macrophages promote epithelial-mesenchymal transition and the cancer stem cell properties in triple-negative breast cancer through CCL2/AKT/β-catenin signaling. Cell Commun Signal 2022;20:92. [Crossref] [PubMed]
- Shen T, Li Y, Wang D, et al. YAP1-TEAD1 mediates the perineural invasion of prostate cancer cells induced by cancer-associated fibroblasts. Biochim Biophys Acta Mol Basis Dis 2022;1868:166540. [Crossref] [PubMed]
- Wang Y, Qin C, Zhao B, et al. EGR1 induces EMT in pancreatic cancer via a P300/SNAI2 pathway. J Transl Med 2023;21:201. [Crossref] [PubMed]
- Zhao J, Geng L, Duan G, et al. REC8 inhibits EMT by downregulating EGR1 in gastric cancer cells. Oncol Rep 2018;39:1583-90. [Crossref] [PubMed]
- Yan J, Gao Y, Lin S, et al. EGR1-CCL2 Feedback Loop Maintains Epithelial-Mesenchymal Transition of Cisplatin-Resistant Gastric Cancer Cells and Promotes Tumor Angiogenesis. Dig Dis Sci 2022;67:3702-13. [Crossref] [PubMed]
- Xu GD, Shi XB, Sun LB, et al. Down-regulation of eIF5A-2 prevents epithelial-mesenchymal transition in non-small-cell lung cancer cells. J Zhejiang Univ Sci B 2013;14:460-7. [Crossref] [PubMed]
- Guan Y, Shi H, Xiao T. NUAK1 knockdown suppresses prostate cancer cell epithelial-mesenchymal transition, migration, and invasion through microRNA-30b-5p. Int J Clin Exp Pathol 2018;11:5694-704. [PubMed]
- Chu DX, Jin Y, Wang BR, et al. LncRNA HOTAIR Enhances Epithelial-to-mesenchymal Transition to Promote the Migration and Invasion of Liver Cancer by Regulating NUAK1 via Epigenetic Inhibition miR-145-5p Expression. J Cancer 2023;14:2329-43. [Crossref] [PubMed]
- Yin X, Teng X, Ma T, et al. RUNX2 recruits the NuRD(MTA1)/CRL4B complex to promote breast cancer progression and bone metastasis. Cell Death Differ 2022;29:2203-17. [Crossref] [PubMed]
- Urabe F, Kosaka N, Ito K, et al. Extracellular vesicles as biomarkers and therapeutic targets for cancer. Am J Physiol Cell Physiol 2020;318:C29-39. [Crossref] [PubMed]