MFAP2 induces epithelial-mesenchymal transformation of osteosarcoma cells by activating the Notch1 pathway
Highlight box
Key findings
• Microfibril-associated protein 2 (MFAP2) was an upstream regulator of the Notch1 signaling pathway to promote epithelial-mesenchymal transition (EMT) in osteosarcoma (OS) cells.
What is known and what is new?
• EMT is a pivotal biological process that participates in the initiation, progression, and metastasis of OS.
• The manuscript revealed that MFAP2 induces EMT in OS by activating the Notch1 pathway.
What is the implication, and what should change now?
• The findings highlight the key player of MFAP2 in EMT and its promotion of Notch1 signaling, providing valuable insights into the molecular mechanisms underlying OS progression. Targeting MFAP2 may offer potential therapeutic strategies for inhibiting EMT and impeding OS tumor growth and metastasis.
• Given the manuscript’s findings based on the in vitro experiments, additional studies should be conducted to further explore in vivo metastasis.
Introduction
Osteosarcoma (OS), an exceptionally aggressive bone tumor, predominantly afflicts adolescents and young adults, comprising approximately 35% of all primary malignant bone tumors (1,2). The combination of neoadjuvant chemotherapy and advancements in surgical techniques has significantly increased overall survival rates, now approaching 70% (2). Despite treatment advances, the outlook for metastatic OS patients remains grim, highlighting the urgent need for a deeper understanding of the molecular mechanisms underlying its pathogenesis and progression (3).
Distant tumor cell metastasis continues to be a significant impediment to the effective treatment of OS patients (4). Epithelial-mesenchymal transition (EMT) is a pivotal biological process that participates in the initiation, progression, and metastasis of OS (5,6). This process leads to the loss of polarity and adhesive properties in epithelial cells, endowing them with migratory and invasive characteristics as they transform into mesenchymal-like cells (7). Capobianco et al. found that vitamin D can inhibit OS through EMT, suggesting the potential for targeting EMT to treat OS (8).
Microfibril-associated protein 2 (MFAP2) is a protein-coding gene located at 1p36.13 (9). It plays a significant role in influencing cell motility (10). Studies have shown that MFAP2 promotes EMT in tumor cells, which are involved in tumor invasion and metastasis, such as in liver cancer (11) and gastric cancer (12). Wang et al. highlighted that MFAP2 contributed to facilitating the EMT process in gastric cancer (12). Additionally, Chen et al. discovered that silencing MFAP2 resulted in heightened expression of the epithelial marker, E-cadherin, and decreased expression of the mesenchymal marker, vimentin, in B16 melanoma cells. This process implied that MFAP2 promoted the development of EMT, which in turn regulated the migration and invasion of melanoma cells (13). However, whether MFAP2 can promote the development of EMT in OS remains to be determined.
The protein product of the MFAP2 gene, MAGP1 (14), has been demonstrated to bind to the Notch1 receptor, thereby activating signaling (15). Notch1 signaling is a highly conservative pathway that plays essential roles in cell fate determination and tissue development (16). Upon the binding of the Notch receptor to its corresponding ligand on the surface of neighboring cells, the intracellular domain is released from the cell membrane, translocated into the cell nucleus, and interacts with downstream molecules to regulate the Notch cascade (17). Research has demonstrated the significant involvement of the Notch1 pathway in the process of EMT (18). Activation of Notch1 signaling promotes EMT, which allows epithelial cells to acquire mesenchymal features and enhances their migratory and invasive abilities, thereby promoting tumor metastasis and invasion in breast cancer (18). Gao et al. demonstrated that Notch1 promoted the malignant progression of OS through activation of the cell division cycle 20 (19). Nevertheless, it is currently uncertain whether MFAP2 promotes EMT in OS by mediating the Notch1 pathway.
This study aimed to investigate the impact of MFAP2 on EMT and its potential relationship with the Notch1 signaling in OS. Our findings may provide valuable insights into the complex regulatory network that drives OS progression and metastasis, ultimately contributing to the development of targeted therapies and improved clinical outcomes for OS patients. We present this article in accordance with the ARRIVE reporting checklist (available at https://tcr.amegroups.com/article/view/10.21037/tcr-23-2035/rc).
Methods
Network pharmacology analysis
Data collection and pre-processing
The gene chip data for OS, including GSE28424 (comprising 4 normal samples and 19 disease samples), GSE42572 (comprising 5 normal samples and 7 disease samples), and GSE126209 (comprising 11 normal samples and 12 disease samples), were downloaded from the Gene Expression Omnibus (GEO) database (https://www.ncbi.nlm.nih.gov/geo/). Subsequently, gene annotation was performed for the probe sets based on the gene chip platform. Then, the data from all datasets were merged and batch effects were removed using the sva package in R software version 4.2.2. The study was conducted in accordance with the Declaration of Helsinki (as revised in 2013). A protocol was prepared before the study without registration.
Validation of differential expression of MFAP2 based on the merged dataset
Gene chip data for MFAP2 were extracted from the merged dataset. The ‘Wilcox. test’ method was employed to assess its differential expression between the normal group and the OS group.
Enrichment analysis of gene set variation analysis (GSVA)
Pathways related to Notch1 were downloaded from the MSigDB database (20). Enrichment analysis was performed on previously merged datasets using the R software version 4.2.2 and the GSVA package to obtain activity scores of pathways in the samples. Subsequently, the t-test method was applied to identify differential pathways associated with OS. Finally, the Pearson correlation method was used to assess the correlation between the selected pathways and MFAP2.
Cell culture
The human normal osteoblast cell line hFOB1.19 and OS cell lines HOS, U2OS, and MG63 were sourced from Wuhan Pricella Biotechnology Co., Ltd. (Wuhan, China). These cell lines were cultured in specific media: hFOB1.19 in DMEM/F12 (the 1:1 mixture of Dulbecco’s Modified Eagle’s Medium and Ham’s F-12 Nutrient Mixture.), HOS in MEM, U2OS in DMEM, and MG63 in high-glucose DMEM (Gibco, CA, USA). The culture media were enriched with 10% fetal bovine serum (FBS) from Ephraim (Tianjin, China) and 1% double antibody from Hyclone (UT, USA). The cells were maintained in a 5% CO2 incubator (Thermo Fisher Scientific, MA, USA) at 37 ℃.
Cell treatment and groups
After 4 h of starvation in the recommended growth medium containing 1% FBS, U2OS cells were treated with 50 ng/mL of epidermal growth factor (EGF) for 30 min as the EFG group. Subsequently, the cells were transfected with lentiviral vectors for MFAP2 knockdown and subjected to packaging for 48 h as EGF + shRNA targeting MFAP2 (sh-MFAP2) group. The cells in the EGF + sh-NC (shRNA targeting negative control) group were transfected with sh-NC. U2OS cells stably transfected with sh-MFAP2 were treated for 24 h with the Notch1 activator valproic acid (VPA; 3 mM; YZ-1708707, Solarbio, Beijing, China) as the sh-MFAP2 + VPA group.
Animals and diets
Twelve nude mice (BALB/c, 6 weeks old, weighing 18–20 g) were sourced from the Experimental Animal Center of Yangzhou University (Jiangsu, China). The mice were kept under controlled conditions, with a consistent temperature of 23 ℃ and a 12-h light/dark cycle. They had unrestricted access to both food and water. All animal-related procedures and experiments were conducted in strict accordance with institutional ethical standards and guidelines and were approved by the Ethical Committee for Animal Welfare at Yangzhou University (Approval No: 202308009).
Tumor model construction
The mice were initially separated into two cohorts: the sh-NC group and the sh-MFAP2 group. After a one-week acclimation period, 4×106 U2OS cells, transfected with either sh-NC or sh-MFAP2, were introduced into the right axillary region of each mouse via subcutaneous injection (21,22). After 28 days, euthanasia was administered to the mice through the inhalation of 7.5% isoflurane, and the resulting tumors were collected. Detailed records and photographic documentation of the tumors were made, and they were subsequently preserved by fixation in a solution of 4% paraformaldehyde. The measurement of tumor volume was performed every 4 days, utilizing the formula: volume (mm3) = 1/2 × length × width2.
Cell transfection
To achieve MFAP2 knockdown, U2OS cells were subjected to transfection with specific shRNA oligonucleotide duplexes targeting MFAP2 (shMFAP2), while a control sh-NC was also employed, and both were obtained from VectorBuilder Inc. (Guangzhou, China). When cellular confluence reached between 70% to 90%, we proceeded with the gradual viral transduction using either sh-NC or sh-MFAP2. Following an 18-h transfection period, the culture medium was refreshed with a new complete culture medium. After 48 h of transfection, the culture medium was once again replaced, this time with a complete culture medium supplemented with 2.5 µg/mL puromycin, facilitating the selection of stably transfected cell lines. The efficiency of gene knockout was subsequently confirmed through quantitative real-time polymerase chain reaction (qRT-PCR).
qRT-PCR
A qRT-PCR approach was employed to analyze changes in gene expression. First, following the experimental protocol of Amirouche et al. (23), adjustments were made to perform RNA extraction using the Trizol method. Subsequently, cDNA synthesis was performed using the FastKing gDNA Dispelling RT SuperMix kit (TIANGEN, Beijing, China) according to the manufacturer’s instructions. For qRT-PCR reactions, SYBR Green PCR Master Mix (Lifeint, Xiamen, China) was utilized under the following conditions: an initial denaturation step at 95 ℃ for 30 s, followed by 40 cycles consisting of 5 s at 95 ℃ and 34 s at 60 ℃. The relative gene expression was determined using the 2-ΔΔCt method, with GAPDH serving as the internal reference for normalization. Here are the primer sequences: MFAP2 (human) forward primer: 5’-CTG ACC ACG TCC AGT ACA CC-3’, reverse primer: 5’-TCC AGC TCT GCA TTT CCT GG-3’. GAPDH (human) forward primer: 5’-GGA GCG AGA TCC CTC CAA AAT-3’, reverse primer: 5’-GGC TGT TGT CAT ACT TCT CAT GG-3’.
Cell viability assays
Cell viability was assessed through the utilization of the Cell Counting Kit-8 (CCK-8) assay. Initially, 100 µL of cell suspension containing 2,000 cells per well was inoculated into a 96-well plate. This culture plate was then carefully placed within a 37°C incubator enriched with 5% CO2 for pre-cultivation. Once the cells had achieved complete adherence, the transfection procedure was executed by the previously outlined steps. Following the transfection process, 10 µL of CCK-8 reaction solution sourced from Beyotime (Shanghai, China), was meticulously introduced into each well. Subsequently, the culture plate was incubated within a controlled environment for a period of 2 h. Upon completion of the incubation, the absorbance of the samples was quantified at 450 nm utilizing a microplate reader (Wuxi Hiwell Diatek, Nanjing, China).
Migration assays
Wound-healing assay was conducted to evaluate the migratory capacity of OS cells. First, horizontal lines were meticulously drawn at even intervals of approximately 0.5 cm on the underside of a 6-well plate, ensuring that each of the 6 wells was intersected by at least three lines. Subsequently, around 5×105 U2OS cells were carefully introduced into each well, ensuring uniform coverage across the base. On the following day, the pipette tip was positioned as vertically as possible, aligned with a ruler over the previously drawn horizontal lines on the plate’s underside, with a focus on maintaining a perpendicular orientation. Following these preparations, phosphate-buffered saline (PBS) was employed for a triple-round wash of the cell culture, with any dislodged cells being promptly removed. Subsequently, the serum-free culture medium was added to the wells. Sampling was carried out at 0 h, 24 h, and 48 h, during which images of the wound were meticulously captured using a microscope (Olympus, PA, USA). The formula was as follows: migration rate (%) = [1 – (wound area at 24 h or 48 h/wound area at 0 h)] × 100%.
Invasion assays
Matrigel was diluted 1:4 in culture medium to reach a final concentration of 50 mg/L to prepare the Matrigel coating. Then, we added 50 µL of this diluted Matrigel solution to the upper side of the Transwell chamber membrane and allowed it to solidify into a gel by incubating at 37 ℃ for 4 h. For cell seeding and invasion assays, we enzymatically detached the cells, washed them with PBS, and resuspended them in a serum-free culture medium to achieve a cell density of 1×105 cells/mL. Then, we added 200 µL of this cell suspension to the upper chamber of the Transwell. In the lower chamber, we placed a culture medium containing 20% FBS. After 24 h of incubation at 37 ℃ in a CO2 incubator for cell invasion, we removed the Transwell chambers. To assess invasion, we wiped off non-migrated cells from the upper side of the membrane with a cotton swab. We rinsed the chambers with PBS three times to remove any remaining non-migrated cells. Next, we fixed the chambers in ice-cold methanol for 30 min at 4 ℃. After air-drying, we stained the migrated cells with crystal violet for 20 min. The excess stain was washed off by rinsing the chambers with PBS three times. During the Transwell assay, imaging and cell counting were performed on three randomly selected fields of view using a microscope (Olympus).
Western blotting
Cellular protein extraction was meticulously conducted using ice-cold radioimmunoprecipitation assay buffer (Beyotime). To ascertain the protein concentration, the highly regarded bicinchoninic acid (BCA) method from Beyotime was adeptly applied. Protein specimens were effectively separated utilizing precise 10% sodium dodecyl sulfate-polyacrylamide gel electrophoresis, after which they were judiciously transferred onto specialized 0.45 µm polyvinylidene fluoride (PVDF) membranes (Beyotime). A comprehensive blocking process, employing 5% non-fat milk dissolved in TBST (Tris buffered saline with Tween 20), was meticulously carried out for a duration of 1 h at room temperature. Subsequently, these membranes underwent an incubation period of utmost precision, where they were allowed to interact with the corresponding primary antibodies at a constant temperature of 4 ℃ throughout the night. Following this crucial step, a secondary antibody was applied for a duration of 1 h. Finally, a mixture of equal volumes comprising the electrochemiluminescence detection reagents A and B (Applygen, Beijing, China) was expertly concocted. The PVDF membrane, after a meticulous 5-min wash, was delicately swathed with plastic wrap. After this, the membrane was artfully exposed to X-ray film (Servicebio, Wuhan, China) within the confines of a darkroom. The exposed film was subjected to scanning procedures and the resultant data was adeptly archived for subsequent analysis. To express the relative protein content, precise measurements of grayscale values of the protein bands were made, with particular attention given to comparing them against the grayscale values of internal reference protein bands. The specific primary antibodies employed in this study included GAPDH (1:1,000, #2118, CST, MA, USA), β-Catenin (1:1,000, #AF6266, Affinity, ON, CAN), N-Cadherin (1:1,000, #AF5239, Affinity), Vimentin (1:1,000, #AF7013, Affinity), and Notch1 (1:1,000, #3608, CST). For the secondary antibody, a Goat Anti-Rabbit IgG H&L (HRP; 1:2,000, #ab205718, Abcam, CB, UK) was judiciously employed.
Statistical analysis
The data were expressed as the mean ± standard deviation from three independent experiments. Statistical analysis was performed using one-way analysis of variance (ANOVA) followed by Tukey’s post-hoc test to assess differences between groups. GraphPad 8.0 software was utilized for all statistical analyses, and P<0.05 denoted statistical significance.
Results
MFAP2 promotes proliferation, migration, and invasion in OS cells
To investigate the mechanism of MFAP2 in OS, we first evaluated the expression of MFAP2 in human normal osteoblasts hFOB1.19 and OS cell lines HOS, U2OS, and MG63. We observed significant MFAP2 mRNA upregulation in OS cell lines in comparison with normal osteoblast cells (P<0.01). Notably, U2OS cells exhibited significantly higher MFAP2 mRNA expression than HOS and MG63 cells (P<0.01, Figure 1A). Then, we used sh-MFAP2 to knock down its expression in U2OS cells. It was confirmed that MFAP2 silenced at 48 h post-transfection relative to the sh-NC group transfected with an empty virus (P<0.01), demonstrating the successful construction of MFAP2 knockdown in U2OS cells (Figure 1B). Subsequently, we detected the cell viability, migration, and invasion of U2OS cells. Cell migration and invasion are characteristics of tumor malignancy (24). As shown in Figure 1C-1E, our observations revealed that silencing MFAP2 led to a marked reduction in cell viability, as well as decreased migration and invasion abilities in U2OS cells in comparison to the sh-NC group (P<0.01).
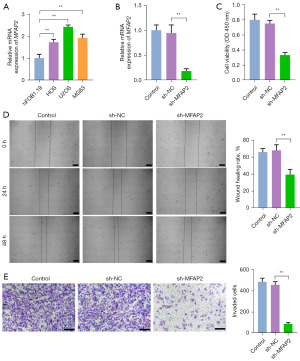
MFAP2 promotes EMT in OS cells
The process of EMT is closely associated with the migration and invasion of tumor cells (24). N-cadherin, vimentin, and β-catenin are biomarkers of EMT (25,26). In contrast to the sh-NC group, the sh-MFAP2 group demonstrated a notable decrease in the protein expression of N-cadherin and vimentin, while there was a marked increase in the protein expression of β-catenin (P<0.01, Figure 2A). To further investigate the effect of MFAP2 on EMT, we induced the U2OS cell lines with EGF, an EMT activator, and then observed cell morphology. As shown in Figure 2B, U2OS cells in the control group exhibited normal morphology, while they transformed into elongated fibroblast-like shapes after EGF treatment. In comparison to the EGF + sh-NC group, inhibition of MFAP2 resulted in EGF-induced U2OS cells exhibiting morphology closer to the normal state.
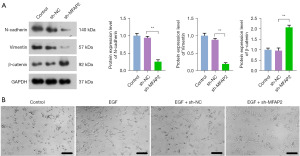
MFAP2 is significantly correlated with Notch1 pathway
Using the sva package in R software version 4.2.2, we merged and removed batch effects from the gene chip data in datasets (GSE28424, GSE42572, and GSE126209). The resulting dataset was designated as Dataset 1, and the integration of data showed promising results (Figure S1). Subsequently, we examined the differential expression of the MFAP2 between the normal and OS groups in Dataset 1. The expression level of MFAP2 was notably higher in the OS group compared to the normal group, as illustrated in Figure 3A (P<0.05). To explore the association between MFAP2 and the Notch1 pathway, we initially retrieved five pathways from the MsigDB database. Following GSVA enrichment analysis and t-test, we identified three pathways with significant differences between the OS and normal groups. These pathways were Reactome_signaling by Notch1, Kyoto Encyclopedia of Genes and Genomes (KEGG)_Notch signaling pathway, and WikiPathways (WP)_Notch signaling. As illustrated in Figure 3B, the activity scores of these three pathways were significantly higher in the OS group than in the normal group (P<0.05). For the selected pathways, we conducted correlation analysis with MFAP2 using the Pearson correlation method. The analysis results demonstrated a significant positive correlation between MFAP2 and the Reactome signaling by the Notch1 pathway as well as the KEGG Notch signaling pathway (Figure 3C, P<0.05).
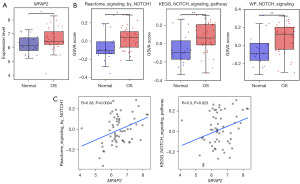
MFAP2 promotes EMT in OS cells by activating the Notch1 pathway
MFAP2 can bind to the Notch1 receptor, thereby initiating signal transduction pathways (27). This activation of the Notch1 signaling pathway can facilitate the onset of EMT, consequently enhancing tumor metastasis and invasion (28). To investigate whether MFAP2 promotes EMT in OS cells through the activation of the Notch1 pathway, we knocked down MFAP2 in OS cells and then treated with VPA (a Notch1 activator). As shown in Figure 4A, in contrast to the sh-NC group, there was a significant reduction in Notch1 protein expression within the sh-MFAP2 group (P<0.05). Nevertheless, the introduction of VPA effectively counteracted the inhibitory impact of MFAP2 knockdown on Notch1 protein expression. These findings affirmed that MFAP2 actively triggered the Notch1 pathway in OS. Subsequently, we proceeded to evaluate the effects on OS cell viability and invasion. The results unveiled that VPA robustly reversed the inhibitory consequences of MFAP2 knockdown on the malignant traits of OS cells. Furthermore, VPA effectively annulled the suppressive influence of MFAP2 knockdown on EMT biomarker levels, leading to a marked increase in the expression of N-cadherin, vimentin, and β-catenin in OS cells (P<0.01, Figure 4B-4D). Together, these results provided sufficient evidence that MFAP2 promoted EMT in OS cells through activation of the Notch1 pathway.
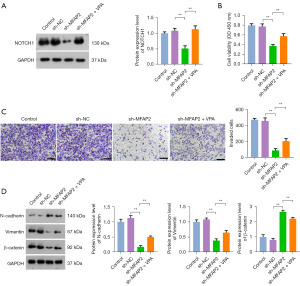
Validation of the promotional effect of MFAP2 on OS progression in xenograft tumor model mice
We also assessed the influence of MFAP2 on tumor growth in the mouse model. Notably, there was a substantial reduction in both tumor volume and weight following MFAP2 knockdown compared to the sh-NC group (P<0.01, Figure 5A,5B). Furthermore, we analyzed the mRNA expression profiles of EMT biomarkers within the OS tumor tissues. Intriguingly, in the sh-MFAP2 group, there was a significant reduction in the expression levels of N-cadherin and vimentin when compared to the sh-NC group (P<0.01, Figure 5C), indicating that inhibiting MFAP2 effectively suppressed the EMT process in OS tumor tissues. Furthermore, western blot analysis results indicated that the Notch1 signaling pathway was markedly inhibited in the sh-MFAP2 group (P<0.01, Figure 5D).
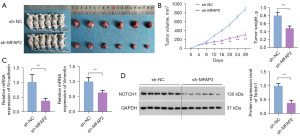
Discussion
The present study demonstrated that MFAP2 induced EMT in OS by activating the Notch1 signaling pathway. First, the significant correlation between MFAP2 and the Notch1 signaling pathway in OS was validated. In addition, our investigation revealed an upregulation of MFAP2 expression in OS. The establishment of stable MFAP2 knockdown led to a noteworthy inhibition in OS cell proliferation, migration, invasion, and the progression of EMT. Furthermore, the activation of Notch1 effectively counteracted the inhibitory effects of MFAP2 knockdown on the malignant behavior of OS cells. Finally, the role of MFAP2 in inducing EMT in OS and its potential relationship with Notch1 activation were verified in a xenograft tumor mice model.
The induction of EMT plays a crucial role in the spread and transfer of malignant cells during the progression of OS (29). The process of tumor EMT encompasses the transformation of epithelial cells into a mesenchymal, fibroblast-like phenotype, resulting in enhanced cellular motility and migratory capacity (30). This transition involves the reduction in the expression of epithelial cell markers such as β-catenin and E-cadherin, accompanied by the concurrent increase in the expression of mesenchymal cell markers, including N-cadherin, vimentin, and fibronectin (4,31). Prior research has provided evidence of MFAP2’s involvement in the regulation of EMT across various cancer types (12). Dong et al. found that there was an elevation in E-cadherin expression while reduction in vimentin, N-cadherin, and β-catenin after suppressing MFAP2 expression in papillary thyroid carcinoma cells (9). As part of this analysis, MFAP2 knockdown reduced cell viability, migration, and invasion capability of U2OS cells and OS tumor size in mice. Importantly, our results demonstrated that MFAP2 knockdown effectively upregulated the levels of β-catenin and downregulated the levels of N-cadherin and vimentin in U2OS cells as well as OS tumor tissues. The differences in β-catenin expression might be associated with the characteristics of the tumor and the status of its internal signaling pathways. Moreover, EGF is an important inducer of EMT (32). In our study, MFAP2 knockdown U2OS cell lines treated with EGF exhibited morphology closer to the normal state, suggesting that MFAP2 knockdown inhibited the activation of EMT. Thus, these results demonstrated that MFAP2 inhibition restressed malignant features, especially EMT, in OS.
The interaction between MFAP2 and Notch1 results in the release of the extracellular domain of Notch1, subsequently activating Notch signaling (15). The impact of MFAP2 on Notch signaling holds significance in cancer (33). In our study, MFAP2 exhibited a significant correlation with the Notch1 signaling pathway in OS. Furthermore, MFAP2 knockdown led to a reduction in Notch1 expression of OS tumor tissues. Taken together, these findings suggested that MFAP2 functioned upstream of the Notch signaling in U2OS cells. Additionally, Notch1 signaling is a key driver for EMT activation (34). Besides, Zhang et al. proved that the Notch1 signaling pathway plays a crucial role in OS (35). He et al. also found that the suppression of Notch1 signaling led to a decrease in cell proliferation and an enhancement in the osteogenic differentiation of bone marrow mesenchymal stem cells (17). Moreover, Gao et al. concluded that inhibition of Notch1 suppressed cell viability, migration, and invasive capability of OS cells, thereby inhibiting the malignant development of OS (19). These findings aligned with our results. We observed that the Notch1 activator reversed the inhibition effects of MFAP2 knockdown on cell viability, invasion, and EMT in U2OS cells. These results supported the role of MFAP2 in promoting the EMT of OS cells by activating the Notch1 pathway.
Despite the significant progress made in this study, further investigations are required to identify potential crosstalk with other signaling pathways involved in EMT regulation. Moreover, current research is primarily focused on cellular and mouse models, and the translation to clinical applications still requires further research for validation and confirmation.
Conclusions
Our research demonstrates that MFAP2 induces EMT in OS cells by activating the Notch1 pathway (Figure 6). Our findings indicated a significant upregulation of MFAP2 in both OS cells and tumor tissues. MFAP2 knockdown inhibited OS tumor growth, malignant characteristics, and EMT progress. Notch1 activation reversed the inhibition of MFAP2 knockdown on OS progression and EMT. The identification of MFAP2 as a key player in EMT and its promotion of Notch1 signaling provides valuable insights into the molecular mechanisms underlying OS progression. Targeting MFAP2 may offer potential therapeutic strategies for inhibiting EMT and impeding OS tumor growth and metastasis. However, we initially demonstrated the promotion of EMT by MFAP2 through the assessment of EMT-related markers (N-cadherin and vimentin) in in vitro experiments. Additional studies will be conducted to further explore in vivo metastasis.
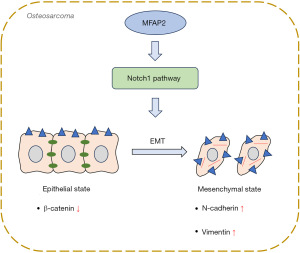
Acknowledgments
Funding: This work was supported by
Footnote
Reporting Checklist: The authors have completed the ARRIVE reporting checklist. Available at https://tcr.amegroups.com/article/view/10.21037/tcr-23-2035/rc
Data Sharing Statement: Available at https://tcr.amegroups.com/article/view/10.21037/tcr-23-2035/dss
Peer Review File: Available at https://tcr.amegroups.com/article/view/10.21037/tcr-23-2035/prf
Conflicts of Interest: All authors have completed the ICMJE uniform disclosure form (available at https://tcr.amegroups.com/article/view/10.21037/tcr-23-2035/coif). The authors have no conflicts of interest to declare.
Ethical Statement: The authors are accountable for all aspects of the work in ensuring that questions related to the accuracy or integrity of any part of the work are appropriately investigated and resolved. The study was conducted in accordance with the Declaration of Helsinki (as revised in 2013). All animal-related procedures and experiments were conducted in strict accordance with institutional ethical standards and guidelines and were approved by the Ethical Committee for Animal Welfare at Yangzhou University (Approval No: 202308009).
Open Access Statement: This is an Open Access article distributed in accordance with the Creative Commons Attribution-NonCommercial-NoDerivs 4.0 International License (CC BY-NC-ND 4.0), which permits the non-commercial replication and distribution of the article with the strict proviso that no changes or edits are made and the original work is properly cited (including links to both the formal publication through the relevant DOI and the license). See: https://creativecommons.org/licenses/by-nc-nd/4.0/.
References
- Zhao X, Wu Q, Gong X, et al. Osteosarcoma: a review of current and future therapeutic approaches. Biomed Eng Online 2021;20:24. [Crossref] [PubMed]
- Jiang Y, Wang J, Sun M, et al. Multi-omics analysis identifies osteosarcoma subtypes with distinct prognosis indicating stratified treatment. Nat Commun 2022;13:7207. [Crossref] [PubMed]
- Sheng G, Gao Y, Yang Y, et al. Osteosarcoma and Metastasis. Front Oncol 2021;11:780264. [Crossref] [PubMed]
- Shao S, Piao L, Wang J, et al. Tspan9 Induces EMT and Promotes Osteosarcoma Metastasis via Activating FAK-Ras-ERK1/2 Pathway. Front Oncol 2022;12:774988. [Crossref] [PubMed]
- Yang J, Antin P, Berx G, et al. Guidelines and definitions for research on epithelial-mesenchymal transition. Nat Rev Mol Cell Biol 2020;21:341-52. [Crossref] [PubMed]
- Yu X, Yustein JT, Xu J. Research models and mesenchymal/epithelial plasticity of osteosarcoma. Cell Biosci 2021;11:94. [Crossref] [PubMed]
- Kim H, Lee S, Shin E, et al. The Emerging Roles of Exosomes as EMT Regulators in Cancer. Cells 2020;9:861. [Crossref] [PubMed]
- Capobianco E, McGaughey V, Seraphin G, et al. Vitamin D inhibits osteosarcoma by reprogramming nonsense-mediated RNA decay and SNAI2-mediated epithelial-to-mesenchymal transition. Front Oncol 2023;13:1188641. [Crossref] [PubMed]
- Dong SY, Chen H, Lin LZ, et al. MFAP2 is a Potential Diagnostic and Prognostic Biomarker That Correlates with the Progression of Papillary Thyroid Cancer. Cancer Manag Res 2020;12:12557-67. [Crossref] [PubMed]
- Yao LW, Wu LL, Zhang LH, et al. MFAP2 is overexpressed in gastric cancer and promotes motility via the MFAP2/integrin α5β1/FAK/ERK pathway. Oncogenesis 2020;9:17. [Crossref] [PubMed]
- Xu W, Wang M, Bai Y, et al. The role of microfibrillar-associated protein 2 in cancer. Front Oncol 2022;12:1002036. [Crossref] [PubMed]
- Wang JK, Wang WJ, Cai HY, et al. MFAP2 promotes epithelial-mesenchymal transition in gastric cancer cells by activating TGF-β/SMAD2/3 signaling pathway. Onco Targets Ther 2018;11:4001-17. [Crossref] [PubMed]
- Chen Z, Lv Y, Cao D, et al. Microfibril-Associated Protein 2 (MFAP2) Potentiates Invasion and Migration of Melanoma by EMT and Wnt/β-Catenin Pathway. Med Sci Monit 2020;26:e923808. [Crossref] [PubMed]
- Combs MD, Knutsen RH, Broekelmann TJ, et al. Microfibril-associated glycoprotein 2 (MAGP2) loss of function has pleiotropic effects in vivo. J Biol Chem 2013;288:28869-80. [Crossref] [PubMed]
- Miyamoto A, Lau R, Hein PW, et al. Microfibrillar proteins MAGP-1 and MAGP-2 induce Notch1 extracellular domain dissociation and receptor activation. J Biol Chem 2006;281:10089-97. [Crossref] [PubMed]
- Zhou B, Lin W, Long Y, et al. Notch signaling pathway: architecture, disease, and therapeutics. Signal Transduct Target Ther 2022;7:95. [Crossref] [PubMed]
- He Y, Zou L. Notch-1 inhibition reduces proliferation and promotes osteogenic differentiation of bone marrow mesenchymal stem cells. Exp Ther Med 2019;18:1884-90. [Crossref] [PubMed]
- Miao K, Lei JH, Valecha MV, et al. NOTCH1 activation compensates BRCA1 deficiency and promotes triple-negative breast cancer formation. Nat Commun 2020;11:3256. [Crossref] [PubMed]
- Gao Y, Bai L, Shang G. Notch-1 promotes the malignant progression of osteosarcoma through the activation of cell division cycle 20. Aging (Albany NY) 2020;13:2668-80. [Crossref] [PubMed]
- Liberzon A, Birger C, Thorvaldsdóttir H, et al. The Molecular Signatures Database (MSigDB) hallmark gene set collection. Cell Syst 2015;1:417-25. [Crossref] [PubMed]
- Liu Y, She W, Li Y, et al. Aa-Z2 triggers ROS-induced apoptosis of osteosarcoma by targeting PDK-1. J Transl Med 2023;21:7. [Crossref] [PubMed]
- Sun X, Zhao X, Xu S, et al. CircSRSF4 Enhances Proliferation, Invasion, and Migration to Promote the Progression of Osteosarcoma via Rac1. Int J Mol Sci 2022;23:6200. [Crossref] [PubMed]
- Amirouche A, Ait-Ali D, Nouri H, et al. TRIzol-based RNA extraction for detection protocol for SARS-CoV-2 of coronavirus disease 2019. New Microbes New Infect 2021;41:100874. [Crossref] [PubMed]
- Liu W, Xin M, Li Q, et al. IL-17A Promotes the Migration, Invasion and the EMT Process of Lung Cancer Accompanied by NLRP3 Activation. Biomed Res Int 2022;2022:7841279. [Crossref] [PubMed]
- Zhu GJ, Song PP, Zhou H, et al. Role of epithelial-mesenchymal transition markers E-cadherin, N-cadherin, β-catenin and ZEB2 in laryngeal squamous cell carcinoma. Oncol Lett 2018;15:3472-81. [Crossref] [PubMed]
- Chaw SY, Abdul Majeed A, Dalley AJ, et al. Epithelial to mesenchymal transition (EMT) biomarkers--E-cadherin, beta-catenin, APC and Vimentin--in oral squamous cell carcinogenesis and transformation. Oral Oncol 2012;48:997-1006. [Crossref] [PubMed]
- Haider C, Hnat J, Wagner R, et al. Transforming Growth Factor-β and Axl Induce CXCL5 and Neutrophil Recruitment in Hepatocellular Carcinoma. Hepatology 2019;69:222-36. [Crossref] [PubMed]
- Qian XQ, Tang SS, Shen YM, et al. Notch1 Affects Chemo-resistance Through Regulating Epithelial-Mesenchymal Transition (EMT) in Epithelial Ovarian cancer cells. Int J Med Sci 2020;17:1215-23. [Crossref] [PubMed]
- Cao Y, Chen E, Wang X, et al. An emerging master inducer and regulator for epithelial-mesenchymal transition and tumor metastasis: extracellular and intracellular ATP and its molecular functions and therapeutic potential. Cancer Cell Int 2023;23:20. [Crossref] [PubMed]
- Dongre A, Weinberg RA. New insights into the mechanisms of epithelial-mesenchymal transition and implications for cancer. Nat Rev Mol Cell Biol 2019;20:69-84. [Crossref] [PubMed]
- Loh CY, Chai JY, Tang TF, et al. The E-Cadherin and N-Cadherin Switch in Epithelial-to-Mesenchymal Transition: Signaling, Therapeutic Implications, and Challenges. Cells 2019;8:1118. [Crossref] [PubMed]
- Liu P, Yang P, Zhang Z, et al. Ezrin/NF-κB Pathway Regulates EGF-induced Epithelial-Mesenchymal Transition (EMT), Metastasis, and Progression of Osteosarcoma. Med Sci Monit 2018;24:2098-108. [Crossref] [PubMed]
- Craft CS, Broekelmann TJ, Mecham RP. Microfibril-associated glycoproteins MAGP-1 and MAGP-2 in disease. Matrix Biol 2018;71-72:100-11. [Crossref] [PubMed]
- Shao S, Zhao X, Zhang X, et al. Notch1 signaling regulates the epithelial-mesenchymal transition and invasion of breast cancer in a Slug-dependent manner. Mol Cancer 2015;14:28. [Crossref] [PubMed]
- Zhang K, Wu S, Wu H, et al. Effect of the Notch1-mediated PI3K-Akt-mTOR pathway in human osteosarcoma. Aging (Albany NY) 2021;13:21090-101. [Crossref] [PubMed]