Predicting response to bacillus Calmette-Guerin in high-risk non-muscle invasive bladder cancer
Introduction
Bladder cancer is the 9th most common malignancy with 573,000 new cases and 213,000 deaths reported worldwide in 2020 (1,2). Cancer of the bladder is often categorized as either non-muscle invasive bladder cancer (NMIBC) or muscle invasive bladder cancer (MIBC). NMIBC encompasses 70% of newly detected cases and includes stage Ta (tumor confined to the epithelium; non-invasive), T1 [invasive to lamina propria (LP)] and Tis [carcinoma in situ (CIS)], and in general is associated with favorable prognosis (3,4). MIBC implies cancer invasion of the muscularis propria, perivesical fat or surrounding organs or structures (T2–T4) and has a high risk of developing metastatic disease. Bladder cancer accounts for considerable expenditure of health care dollars and is often considered the costliest cancer to treat. This is primarily due to high cancer recurrence rates, need for frequent and lifelong surveillance, and cost of surgical and medical treatments (5). This cost burden is largely attributed to NMIBC, which is inherently prone to recurrence secondary to bladder epithelial field cancerization (6,7). What is particularly concerning is the potential for NMIBC, in certain subpopulations, to not only recur but progress to MIBC, significantly decreasing 5-year survival rates from 90% to a range of 37–57% (8,9).
NMIBC can be further sub-divided into categories, based on predicted risk of cancer recurrence and progression. These categories include low, intermediate, and high risk NMIBC. High risk NMIBC has the greatest likelihood of cancer recurrence and progression and clinical care guidelines strongly recommend administration of intravesical bacillus Calmette-Guerin (BCG) following a complete visual trans urethral resection of bladder cancer (TURBT) to reduce bladder cancer recurrence and progression (3,10). The idea that BCG may play a role in treating cancer dates back to 1929 when Pearl observed lower cancer rates in autopsies of tuberculosis patients (11). In general, BCG primarily exerts its activity on bladder epithelial field cancerization as well as minimal residual tumor cells (12-14). BCG is known to bind to fibronectin in the extracellular matrix which facilitates the infiltration to tumoral cells. This, in turn, activates antigen-presenting cells (APCs), leading to the enhancement of immunogenicity in tumor cells (15). Interleukin (IL)-1, IL-6, IL-18, IL-10, IL-12, tumor necrosis factor-α (TNF-α), and interferon (IFN) are secreted following the first installation of BCG, with a more pronounced burst of cytokine secretion after additional installations (16,17). BCG is associated with a complex interplay between the innate and adaptive immune systems that often leads to a shift in the bladder microenvironment from T-helper 2 (Th2) to T-helper 1 (Th1) responses (18,19) (Figure 1).
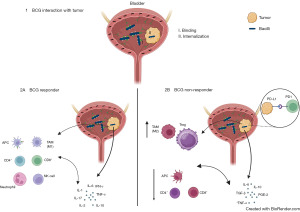
Despite considerable clinical evidence of the efficacy of intravesical BCG reducing cancer, the recurrence and progression rates after 1-year in NMIBC diagnosed patients, will reach up to 61% and 17% respectively in the high-risk group (20). There is an imperative clinical need to better identify patients who will not respond to BCG, especially progression to MIBC, and develop alternative therapeutic opportunities. This article focuses on our current knowledge of identifying patients who are more or less likely to respond to BCG.
EORTC and CUETO tables predicting outcomes in NMIBC
A scoring system has been proposed to predict recurrence and progression of NMIBC and separate the patients into three prognostic risk groups (low, intermediate, high). Sylvester et al. performed a meta-analysis on seven phase 3 clinical trials conducted by the European Organization for Research and Treatment of Cancer (EORTC) (20,21). The EORTC scoring system, assigns weights to prognostic factors including the number of tumors, tumor size, history of previous bladder cancer recurrence, stage (Ta/T1), concomitant CIS, and tumor grade [based on World Health Organization (WHO) 1973] (Table 1). These weighted scores can be utilized to predict the likelihood of recurrence and progression after either 1 or 5 years from the initial tumor occurrence, in an individual patient (20,21). Although the EORTC risk scores provide clinical value, there are several limitations to the scoring model and the most pronounced is most patients did not receive adjuvant intravesical BCG following TURBT. The European Association of Urology (EAU) prognostic factor risk groups for NMIBC were recently updated and incorporated both the WHO 2004/2016 and WHO 1973 classification systems for tumor grade and included a more contemporary group of treated NMIBC patients (22). The multivariate analysis from the study identified tumor stage, tumor grade, concomitant CIS, number of tumors, tumor size and age to form four risk groups for which the probability of progression at 5 years varied from <1% to >40%. Importantly, the study identified a fourth risk group, the new “very high-risk group” for NMIBC progression and emphasized these patients are less likely to respond to intravesical therapy, although BCG was not used in these patients. However, a recent study by Lobo et al, suggests the EAU risk groups, including the very high-risk group, overestimate NMIBC progression and possibly this was expected given lack of BCG treatment in the patients used to derive the EAU risk groups. This notion was also supported by Contieri et al., who assessed the EAU NMIBC 2021 scoring model and concluded the very-high-risk group, who had received BCG, had a significantly lower risk of progression, therefore refinement in clinical predictive tools is needed (23,24).
Table 1
Risk factors | EORTC | CUETO |
---|---|---|
Gender | – | Male, female† |
Age (years) | – | <60, 60–70, >70 |
Number of tumors | 1, 2–7, ≥8 | ≤3 or >3 |
Recurrent tumor | ≤1 recurrence per year, >1 recurrence per year | Yes or no |
T category | Ta, T1 | Ta, T1‡ |
CIS | Yes or no | Yes or no |
Grade (WHO 1973) | G1, G2, G3 | G1, G2, G3 |
Tumor size (cm) | <3, ≥3 | – |
†, gender is relevant on predicting recurrence but not progression; ‡, T stage is relevant of predicting progression but not recurrence. NMIBC, non-muscle invasive bladder cancer; EORTC, European Organization for Research and Treatment of Cancer; CUETO, Club Urologico Espanol de Tratamiento Oncologico; CIS, carcinoma in situ; WHO, World Health Organization.
The Club Urologico Espanol de Tratamiento Oncologico (CUETO) proposed a similar scoring system to predict recurrences and progression in patients with NMIBC. The scoring system was based on four CUETO conducted clinical trials, employing various schedules of adjuvant intravesical BCG following TURBT. The factors most predictive of tumor recurrence included gender (females with worse prognosis), age (older individuals with worse prognosis), tumor frequency (primary or recurrent), number of tumors, concomitant CIS, and tumor grade (Table 1). On the other hand, for tumor progression, gender did not confer a greater risk, while the T category (Ta/T1) emerged as a significant prognostic factor (25).
As mentioned, the EORTC and CUETO scoring systems are valuable tools in clinical practice for counseling patients, however, they have limitations, most pronounced is less than optimal receipt of what is considered the most effective schedule of intravesical BCG. The current standard of care for BCG includes a 6-week induction course followed by maintenance therapy, based on the Southwest Oncology Group (SWOG-8507) protocol. This maintenance therapy is administered weekly for 3 weeks at 3, 6, 12, 18, 24, 30, and 36 months from the initiation of the induction therapy (26). Given that the study populations included in the risk tables did not receive current guideline directed BCG therapy, the models likely overestimate recurrence and progression rates (27). Moreover, both models include prior recurrence of NMIBC as a prognostic factor. This may have limitations as a primary, solitary high grade T1 tumor receives a lower score based on tumor status when compared to a recurrent, multifocal, low grade (G1) Ta tumor for likelihood of progression despite clear evidence that a high grade T1 cancer would be the more concerning disease state. There are ongoing efforts to integrate EORTC and CUETO models with molecular markers, necessitating further investigation for widespread acceptance (28).
A recent study by Ferro et al. focused on predictive factors to identify BCG-unresponsive patients after resection for T1 high-grade NMIBC (29). The study concluded that multifocality, lymphovascular invasion (LVI), and T1 high grade status on re-staging TURBT were independent predictive factors for BCG response. Of note, there was a considerable decline of 5-year overall survival for BCG-nonresponsive comparing to responsive group (82.9% vs. 92.4%, P<0.0001).
T1 substaging and risk of disease progression
Non-muscle invasive tumors that involve the LP staged as T1 cancers, have the greatest risk of cancer recurrence and progression, following TURBT and adjuvant BCG. However, prognosis is very heterogeneous for T1 cancers, and many studies have focused efforts on increasing understanding of outcomes. The LP comprises loose connective tissue with vessels and a thin muscularis mucosa (MM). Diagnosing MM is not always straightforward, as it is not a continuous layer throughout the bladder tissue and is absent in some bladders. Additionally, in cases of bladder outlet obstruction, these muscular bundles may undergo compensatory hypertrophy, posing technical challenges in differentiating them from the muscularis propria (30,31). Based on MM identification, LP invasion was categorized using two mostly similar anatomical systems in prior studies: (I) two category system: T1a; tumor above MM and T1b; tumor invaded MM or (II) three category system T1a; tumor above MM, T1b; tumor invaded MM, and T1c; tumor invasion beyond MM. The assessment of MM as a tool to gauge the depth of invasion and its impact on recurrence and progression has been the focus of several studies (32). A multicenter study reported that T1b tumors were associated with more progression to MIBC when compared to T1a tumors (23% vs. 14%, P=0.01), with 5-year follow-up (33). Orsola et al. conducted a prospective assessment of 89 patients with T1a and 111 patients with T1b bladder cancer. The T1b group exhibited a markedly higher progression rate than the T1a group [23.6% vs. 5.6%, hazard ratio (HR) =0.21, P=0.0004] (34).
While it is recommended by some urologists and pathologists to consider T1 substaging reporting, in their treatment strategy, this is not always feasible or agreed upon by all providers. First, as mentioned above, MM is a non-continuous layer and may not exist in all pathological samples. The presence of MM in pathologic reports varies from 32–100%. Second, there is a lack of consistency in pathologist agreement on degree of T1 invasion (interobserver variability) (32).
An alternative method of defining degree of LP invasion in T1 bladder cancer focuses on measuring quantitative depth of T1 invasion (metric system), independent of the presence or involvement of MM. T1 microinvasive (T1m) is characterized by a depth of invasion less than 0.5 mm, while T1 extensive (T1e) has a greater depth of invasion, exceeding 0.5 mm in a high-power field at ×400 magnification (35). In a study conducted by Fransen van de Putte et al. the prognostication of T1 substaging systems was compared (T1m/e vs. T1a/b). The study concluded that T1e had a higher risk of progression to MIBC than T1m (HR =3.8 and P<0.001) and the T1a/T1b substaging system did not differentiate patients more likely to progress (36). This study suggests measuring depth of LP invasion (i.e., T1m/e system) is more prognostic and likely more practical to implement in clinical practice.
Another pathologic predictor of treatment outcome following BCG in high risk NMIBC is tumor budding (TB). TB is defined as a single cancer cell or fewer than five cancer cells scattered in the stroma (37). TB in various cancers, including colon, breast, lung squamous cell carcinoma, and oral cancers, has been associated with aggressive features and lower survival rates (38-41). A study evaluated the prognostic role of TB in NMIBC and suggested a median bud count ≥0.9 was statistically related to worse recurrence-free survival (RFS) (P=0.005) and progression-free survival (PFS) (P=0.017). The study concluded TB may serve as an alternative system for T1 substaging however this requires further validation (42).
Artificial intelligence (AI) and its application on predicting NMIBC outcomes
AI is a burgeoning technology drawing attention for its potential impact on cancer diagnosis and prognostication. Various patient inputs including imaging findings, pathologic findings, clinical features, and genomic sequencing data are fed into AI systems. These datasets are leveraged for machine learning (ML), enabling AI to acquire the proficiency to perform specific tasks (43). As computers grasp the input-output mapping, they acquire the capability to predict outcomes for new, unseen inputs. Supervised ML involves external supervisors, such as radiologists or pathologists, who oversee and rectify data analysis during feature design and model training. In contrast, deep learning (DL) is a methodology within the ML domain that utilizes artificial neural networks to recognize recurring patterns in intricate datasets (44). DL autonomously integrates feature design and model training without human intervention, showcasing its effectiveness in survival predictions across various cancers like colorectal cancer, hepatocellular carcinoma, and melanoma (45-47). There is growing interest in applications of AI in predicting NMIBC outcomes and response to BCG.
Jansen et al. conducted a review of 328 TURBT specimens from 232 individuals spanning the years 2000 to 2016 (48). These specimens were assessed based on the WHO 2004 classification (low grade, high grade) by three expert pathologists and a DL system in two stages. The methodology correctly classified low-grade and high-grade tumors at rates of 76% and 71%, respectively. Tokuyama et al. also developed an AI-based predictive model for NMIBC (49). In their investigation, 125 patients diagnosed with NMIBC were monitored for at least 2 years after induction BCG. Digital scans of the initial TURBT slides were used, and a pathologist manually identified regions of interest (ROI). The study concentrated on nuclear atypia, evaluating factors such as nuclear size, contour length, major axis length, roundness, solidity, and eccentricity of the nucleus. ML techniques, specifically support vector machine (SVM) and Random Forest (RF), were employed and compared. The SVM-based model accurately predicted a 90% recurrence rate over 2 years, while the RF-based model achieved an 86.7% accuracy. As mentioned earlier, the study focused exclusively on characterizing the nucleus, omitting features such as lymphovascular invasion, TB, or growth patterns. Consequently, the authors proposed utilizing their AI model as an additive tool for risk stratification in future studies.
The enthusiasm for AI and its possible role in prognostication is growing in the field of medicine. A multi-institutional clinical trial (NCT05825950) has been initiated with the objective of developing and validating an AI model for predicting responsiveness to BCG therapy in BCG naïve high-risk NMIBC. This study and others promise to deliver new opportunities for important medical knowledge and possibly improving personalized care.
CD8+ T-cells and neutrophil-lymphocyte ratio (NLR)
The study of lymphocyte infiltration in bladder cancer has been investigated, yielding inconclusive findings. Rouanne et al. reported that a higher density of tumor-infiltrating lymphocytes (TILs) was associated with depth of tumor invasion, but not correlated with survival outcomes (50). On the other hand, in a review of pathology samples from 167 pT1 patients who underwent TURBT, Hülsen et al. found that higher levels of CD3+ cells (and not CD8+) had favorable outcomes but infiltration of both CD3+ and CD8+ T-cells in the tumor were associated with higher tumor grade (G3) and worse clinical outcomes (51). This finding may indicate that the presence of CD8+ T-cells in NMIBC may not always exert an anti-tumoral effect (i.e., T-cell exhaustion), and, in fact, it could be a response to the invasiveness of the bladder tumor.
Recently, the preoperative NLR has gained attention for its potential prognostic value in NMIBC. In a retrospective study evaluating preoperative NLR in 122 newly diagnosed NMIBC cases, an NLR >2.41 was associated with worse 3-year PFS (52). Similar findings of poorer outcomes with an NLR >3 was reported by D’Andrea, with a HR for 5-year RFS and PFS of 1.9 and 2.4, respectively (53). It is hypothesized that a lower number of lymphocytes may diminish their role in the antigenic response to tumoral antigens. On the other hand, an excess of neutrophils may be associated with the production of cytokines and vascular endothelial growth factor (VEGF), acting as a pro-oncogenic element in this setting (54). While the NLR parameter may seem appealing to clinicians due to its cost-effectiveness and ease of performance, it requires systematic validation and incorporation into clinical models. The increase in NLR is not specific to bladder cancer and is also observed in other malignancies such as non-small cell lung cancer, pancreatic cancer, and gastric cancer, all of which are associated with poorer clinical outcomes (55-57). Additionally, it is important to note that smoking can influence the NLR. Smoking induces a systemic inflammatory response and increases the NLR, while smoking cessation is associated with a reduction in this value (58,59).
Genomics of papillary (Ta/T1) high risk NMIBC
Novel insights into molecular carcinogenesis and advancements in genomic characterization have propelled molecular and genomic profiling to the forefront of cancer research. In general, T1 bladder cancers are characterized by mutations in well-known oncogenes and tumor suppressors, genomic instability and a high tumor mutational burden (TMB). However, response to BCG and tumor progression vary considerably amongst T1 cancers, posing intricate challenges for prediction solely based on histopathological features. Consequently, there is an imperative to uncover genetic patterns distinguishing low and high-risk disease progression in these tumors beyond clinical and pathologic factors. Despite ongoing efforts to decipher drivers of progression and recurrence through genetic events, success has been somewhat limited. Intriguingly, similarities in mutational profiles and mutation loads between T1 NMIBC and MIBC contribute to unfavorable clinical outcomes (60,61).
Pietzak et al. molecularly profiled a large cohort of NMIBC tumors using next-generation sequencing (NGS), revealing numerous potentially actionable genetic alterations. High-grade tumors often exhibit alterations in DNA repair genes, aligning with heightened mutational burden. Moreover, mutations in ARID1A, a chromatin modifier gene, and a copy number of gains of CCNE1, a cell cycle regulator, were associated with increased recurrence risk post-BCG treatment (62). Similarly, another study found that ARID1A mutations and CCNE1 amplifications were associated with a poorer response to BCG therapy (63). Microsatellite instability (MSI), particularly the MSI factor MutL homologue 1 (MLH1), emerged as an independent predictor of PFS in a retrospective study of BCG-treated T1 patients (64). Another prospective study of T1 bladder tumors highlighted APOBEC-A mutational signature, ERCC2 mutations and TMB as strongly linked to favorable outcomes (65). APOBEC-associated mutations, APOBEC RNA expression, and TMB were linked to one of the two Ta tumor subtypes identified by Hurst et al. Since TMB leads to a higher presence of neo-antigens, patients with these characteristics may benefit more from BCG therapy (66). Other than single gene events, other studies focused on genomic alterations and clusters of genomic modifications and correlation with prognosis and response to BCG treatment. Specific gene amplifications such as PVRL4, SOX4, E2F3, and PPARG were associated with distinct clinical outcomes in the T1 bladder tumors. Additionally, clusters (MutCN1–3) based on mutations and copy number events correlated with clinical outcomes, where MutCN2 showcased predominantly favorable clinical outcomes (60,65).
Transcriptome profiling
Recent studies have classified T1 bladder cancers into distinct clusters based on transcriptomic profiling, revealing different clinical outcomes and biological characteristics following BCG treatment. These subtypes showed varying responses to BCG, with some exhibiting high recurrence rates, while others demonstrated better response and fewer recurrences (67). However, none of the described subtypes were associated with PFS, likely due to small sample size, and the subtypes require further validation. In a larger cohort study of BCG-naïve T1 tumors managed in a contemporary manner, molecular signatures identified distinct subtypes (BRS1, 2 and 3) predicting response to BCG therapy based on whole transcriptomic sequencing. An aggressive subtype (BRS3), characterized by epithelial-mesenchymal transition (EMT), demonstrated a higher rate of post-BCG progression compared to the other subtypes (BRS1/2), BRS3 was also associated with immune-suppressive gene overexpression, potentially linked to BCG treatment failure. Notably, BRS3 was an independent predictor of PFS in a multivariate model that incorporated clinical and pathologic factors, thus helping better identify high-risk patients who may not respond to BCG (68). In a study by Kim et al., which focused on pT1 patients, a 24-gene signature related to immune response and inflammation was discovered to predict the response to BCG therapy (69). Another study examined the molecular profiles of high-grade T1 patients who had undergone more than 6 months of induction BCG therapy, dividing them into good outcomes (no progression to MIBC or death) and bad outcomes (progression to MIBC or death). The study concluded that while no single gene could statistically predict the response to BCG, gene sets could identify patients with poor responses, such as the downregulation of IFN-alpha and IFN-gamma (IFN-γ) response pathways in tumors with bad outcomes. However, this study was limited by a small number of patients who received BCG (70). Similarly, an updated NMIBC dataset from UROMOL showed no significant difference in BCG failure-free survival between the high-grade T1 subtypes (2a or 2b; n=54) (71). In another cohort (n=156) analyzed by Strandgaard et al, the samples were categorized according to the UROMOL2021 classification system. They found that classes 2a and 2b tumors did not predict response to BCG therapy, consistent with findings from the smaller UROMOL BCG-treated cohort. In clinically BCG-unresponsive patients, elevated expression of immune inhibitory markers such as LAG3, PD-1, KLRG1, HAVCR2, and CTLA-4 was observed compared to BCG-responsive patients. This suggests that CD8 T-cell exhaustion might contribute to BCG resistance (72).
The potential of these genomic predictors to guide treatment decisions for T1 cancers is substantial, aiding in distinguishing between cases amenable to bladder-sparing approaches and those requiring more aggressive interventions like radical cystectomy. As precision oncology evolves, integrating genomic data into prognostic methodologies will be pivotal for effectively managing NMIBC, specifically T1 bladder cancer.
Urinary markers
Early identification of high risk NMIBC patients not responding to intravesical BCG is a major challenge for urologists and holds vital importance to optimize oncological outcomes. Despite exploring various urinary biomarkers for early detection of cancer recurrence, none are routinely used in clinical and not advocated by most international guidelines. However, the continued validation of recently developed molecular tests and development of novel markers holds promise that earlier detection methods will become available in the future.
mRNA urine-based tests like the Cxbladder studied by Kavalieris et al. integrate gene expression, clinical data, and patient information to effectively rule out recurrent urothelial carcinoma (73). Similarly, the Xpert Monitor mRNA urine test, detecting markers that include ABL1, ANXA10, UPK1B, CRH, and IGF2, demonstrated superior sensitivity over urine cytology, especially in high-grade tumors, but need for improved specificity (74).
Urinary fluorescent in situ hybridization (FISH; UroVysion), a molecular cytogenetic test used to detect four specific chromosomal abnormalities, was originally designed for bladder cancer detection, and remains an available tool for predicting recurrence in patients undergoing BCG therapy. A positive FISH result following BCG treatment indicates a higher risk of recurrence and progression. Liem et al. linked recurrence to a positive UroVysion test conducted 3 months post-TURBT, showing 77% accuracy and 59% sensitivity (75). Another large prospective study affirmed the predictive capability of two consecutive UroVysion tests over urine cytology, exhibiting 70% accuracy and 50% sensitivity (76). UroVysion FISH serves as a supportive tool for physicians making treatment decisions, aligning with guidelines from the American Urological Association (AUA) and the Society of Urologic Oncology (SUO) (3).
Another urine-based DNA genome test called EpiCheck, utilizing 15 DNA methylation biomarkers, demonstrated high accuracy (88%) and sensitivity (97%) in high-grade tumors (77). A compiled list of recently studied urinary based assays used for surveillance of bladder cancer is given in Table 2. Performance characteristics of urine cytology based on Paris consensus classification is also included in Table 2.
Table 2
Urinary assays | Biomarkers | Sensitivity (%) | Specificity (%) | Reference |
---|---|---|---|---|
Cxbladder (mRNA based) | IGFBP5, HOXA13, MDK, CDC2, CXCR2 | 91 | 90 | (73) |
XpertBC (mRNA based) | ABL1, ANXA10, UPK1B, IGF2, CRH | 46.2 | 77 | (74) |
UroVysion (FISH)† | Cytogenetic test | 59 | 84 | (75) |
EpiCheck (DNA methylation) | 15 proprietary DNA methylated genes | 68.2 | 88 | (77) |
Urine cytology‡ | – | 67 | 85 | (78) |
CyPRIT (cytokine assay) | IL-2, IL-6, IL-8, IL-18, IL-1ra, TRAIL, IFN-γ, IL-12, and TNF-α | 85.5 | – | (79) |
†, the test was conducted 3 months post TURBT; ‡, TPS for reporting urine cytology. NMIBC, non-muscle invasive bladder cancer; FISH, fluorescent in situ hybridization; IL, interleukin; IFN-γ, interferon-gamma; TNF, tumor necrosis factor; TURBT, trans urethral resection of bladder cancer; TPS, The Paris System.
Urinary cytokines have emerged as promising predictors of identifying NMIBC patients more or less likely to respond to BCG treatment. These molecules play crucial roles in orchestrating downstream immune cell recruitment, shaping the immune landscape, and directly affecting tumor cell destruction during the post-BCG immune response. BCG treatment can alter the urinary cytokine environment from a Th2 profile towards a Th1 profile. This shift, marked by increased levels of cytokines like IL-2, IL-12, and IFN-γ, is believed to be essential for a positive clinical response to BCG (80). Analysis of cytokine profiles can gauge the effectiveness of the BCG-induced cytotoxic response and can be detected in urine within 1–4 weeks from the treatment’s initiation (16). Among these cytokines, IL-2 has been extensively studied. As a fundamental Th-1 cytokine, IL-2 is released by activated CD4+ T cells and stimulates the proliferation of cytotoxic CD8+ lymphocytes, activates macrophages, and triggers delayed hypersensitivity reactions. Various research groups have noted higher IL-2 levels in the urine of BCG responders compared to non-responders, and notably, IL-2 levels tend to peak earlier than IL-10 levels (Th2 cytokine), in responders (81,82). Further studies have confirmed the predictive roles of IL-10 and TNF-α in the initial response to BCG, influencing subsequent RFS and PFS (83). Two hours after the initial BCG instillation, higher levels of IL-8 were found to be associated with better outcomes in BCG therapy following TURBT (84).
Researchers have explored ratios between Th1/Th2 markers to navigate the intricate interactions among cytokines. For instance, the ratio between IL-6 and IL-10 demonstrated 83% sensitivity and 76% specificity in predicting recurrence post-BCG, a finding later confirmed in a larger cohort of T1 patients (85,86). Given the complexity of the immune response to BCG, it is unlikely that any single cytokine or biomarker alone can definitively predict a positive or negative treatment response. Kamat et al. developed the CyPRIT panel, comprising nine inducible cytokines (IL-2, IL-6, IL-8, IL-18, IL-1ra, TRAIL, IFN-γ, IL-12, and TNF-α), showing 85.5% accuracy in predicting disease recurrence after BCG (79). Moreover, a multiplex immunoassay targeting 10 urinary proteins exhibited 90% accuracy in predicting disease recurrence in a small cohort of high-grade patients (87), suggesting potential use of urinary biomarkers in selecting T1 patients for responsiveness to BCG. However, utilizing urinary cytokine levels does present potential pitfalls. Since urinary cytokine production might reflect local inflammatory responses, excluding urinary tract infections before analyzing these levels is essential. Additionally, systemic processes might influence urinary cytokine levels, making it unclear whether these changes accurately reflect the magnitude of the local immune response in patients receiving systemic immunomodulators. Importantly, many urinary biomarkers assess the BCG response late in the treatment process, after the patient has already completed therapy. These markers are only detectable in urine during treatment, limiting their ability to offer predictive insights before starting treatment. Interpretation of cytokine levels should be cautious, considering variations due to technical aspects such as assay selection, use of different cut-off values, study design, and patient selection.
Tissue based markers
In recent decades, various tissue-based markers related to different cell growth and differentiation pathways associated with NMIBC have been investigated. Traditionally, markers like p53, pRB, p21, p27, bcl-2 and Ki-67 have been associated with BCG response (88-90). However, studies on these markers have common limitations, including inconsistent measurement methods, subjective interpretations, arbitrary thresholds, small sample sizes, variations in patient selection, and inadequate validation. Some research indicates that molecules linked to antigen presentation might increase either in tumor cells or associated immune cells. For instance, heat shock protein 90 (HSP90), believed to aid antigen presentation and recruit various effector cells, has shown an association with BCG response in a study where low HSP90 expression (<40%) predicted BCG failure (91). Another study found that a greater rise in major histocompatibility complex I expression post-BCG treatment predicted longer RFS (92). However, it is crucial to note that the expression of antigen presentation molecules can be influenced by levels of IFN, which increase during BCG therapy stimulation. Whether these molecules directly contribute to tumor antigen presentation remains uncertain.
In 2017, Cormio et al. conducted a study comparing human epidermal growth factor receptor 2 (HER2) expression between BCG-treated and non-BCG-treated stage T1 bladder cancer patients. They found that HER2 overexpression independently predicted RFS and PFS in both univariate and multivariate analyses (93). In another study by the same researchers, HER2 overexpression, in combination with MSI markers (MLH1 and MSH2), was shown to predict outcomes (64).
With ever increasing interest in immunotherapies for treating bladder cancer the prognostic and predictive roles of the immune microenvironment is being explored. Two independent studies focused on the role of macrophages and found that higher numbers of tumor infiltrating CD68+ tumor-associated macrophages (TAMs) predicted higher recurrence rates after BCG, and hypothesized that these cells are part of the inflammatory circuit that may promote tumor progression (94,95). Similarly, high counts of Treg and TAM were associated with shorter RFS, in another study (96). Moreover, Dowell et al. proposed that an increased number of IL-17+ mast cells could enhance, the effect of BCG immunotherapy (97).
Finally, the expression of CD4 and CD8 molecules has been linked to the response to BCG treatment in NMIBC patients. Notably, in a pilot study involving 40 patients, higher CD4 levels were significantly associated with longer PFS following BCG treatment (98). Another recent study suggested that a positive response to BCG might be related to low serological levels of CD4 and CD8, along with a high density of these lymphocytic populations in neoplastic tissue, especially the non-exhausted CD8 subtype. Conversely, BCG treatment failure seemed correlated with the presence of exhausted CD8 cells (99). However, these promising findings require further investigation due to the limited number of patients involved. CD8+ T-cell exhaustion, characterized by the expression of coinhibitory molecules, is a key feature of BCG resistance. Moreover, BCG activates inhibitory checkpoints such as the NKG2A receptor on natural killer (NK) and CD8+ T cells, as well as PD-1 on CD8+ T cells. It is well known that BCG induces IFN-γ response, which subsequently result in the expression of HLA-E that can bind to the NKG2A and PD-1, ultimately results in acquired resistance to BCG. The higher expression of PD-L1 was observed in patients who are unresponsive to BCG treatment compared to the responders (100). Single cell RNA sequencing found IFN-γ to be highly expressed in recurrent tumors, where it was predominantly produced by NK cells (80,101-103). Furthermore, research by Esteso et al. demonstrated that BCG stimulates the expansion of γδ T-cells alongside CD56high CD16+ NK cells. This T-cell population is known for its high IFN-γ secretion signature (104,105). These findings offer new avenues for developing emerging immunotherapies.
Summary
Despite various advances in treating high risk NMIBC, cancer recurrence within the bladder continues to be a problem. Possibly, even more concerning is the risk of cancer progression for a subset of high-risk patients. Strategies to better identify individuals who will not respond to BCG is of a great need.
Several studies have focused on clinical and pathologic factors to better identify patients at greatest risk of bladder cancer recurrence and progression following BCG. Implementing AI models to clinical and pathologic data may lead to improved identification of patients with a high likelihood of not responding to BCG. Also, as the field of genomics and transcriptomics evolves, augmented by spatial biology, there will be opportunities to increase the predictive ability of clinical models by studying both the tumor and the microenvironment.
Acknowledgments
Funding: None.
Footnote
Peer Review File: Available at https://tcr.amegroups.com/article/view/10.21037/tcr-24-180/prf
Conflicts of Interest: All authors have completed the ICMJE uniform disclosure form (available at https://tcr.amegroups.com/article/view/10.21037/tcr-24-180/coif). The authors have no conflicts of interest to declare.
Ethical Statement: The authors are accountable for all aspects of the work in ensuring that questions related to the accuracy or integrity of any part of the work are appropriately investigated and resolved.
Open Access Statement: This is an Open Access article distributed in accordance with the Creative Commons Attribution-NonCommercial-NoDerivs 4.0 International License (CC BY-NC-ND 4.0), which permits the non-commercial replication and distribution of the article with the strict proviso that no changes or edits are made and the original work is properly cited (including links to both the formal publication through the relevant DOI and the license). See: https://creativecommons.org/licenses/by-nc-nd/4.0/.
References
- Zhang Y, Rumgay H, Li M, et al. The global landscape of bladder cancer incidence and mortality in 2020 and projections to 2040. J Glob Health 2023;13:04109. [Crossref] [PubMed]
- Wéber A, Vignat J, Shah R, et al. Global burden of bladder cancer mortality in 2020 and 2040 according to GLOBOCAN estimates. World J Urol 2024;42:237. [Crossref] [PubMed]
- Chang SS, Boorjian SA, Chou R, et al. Diagnosis and Treatment of Non-Muscle Invasive Bladder Cancer: AUA/SUO Guideline. J Urol 2016;196:1021-9. [Crossref] [PubMed]
- Babjuk M, Burger M, Zigeuner R, et al. EAU guidelines on non-muscle-invasive urothelial carcinoma of the bladder: update 2013. Eur Urol 2013;64:639-53. [Crossref] [PubMed]
- Riley GF, Potosky AL, Lubitz JD, et al. Medicare payments from diagnosis to death for elderly cancer patients by stage at diagnosis. Med Care 1995;33:828-41. [Crossref] [PubMed]
- Marzouka N, Lindgren D, Eriksson P, et al. Recurring urothelial carcinomas are clonal but incompatible with a direct relationship. Urologic Oncology: Seminars and Original Investigations 2020;38:899-900. [Crossref]
- Hafner C, Knuechel R, Zanardo L, et al. Evidence for oligoclonality and tumor spread by intraluminal seeding in multifocal urothelial carcinomas of the upper and lower urinary tract. Oncogene 2001;20:4910-5. [Crossref] [PubMed]
- Breau RH, Karnes RJ, Farmer SA, et al. Progression to detrusor muscle invasion during urothelial carcinoma surveillance is associated with poor prognosis. BJU Int 2014;113:900-6. [Crossref] [PubMed]
- Vlaming M, Kiemeney LALM, van der Heijden AG. Survival after radical cystectomy: Progressive versus De novo muscle invasive bladder cancer. Cancer Treat Res Commun 2020;25:100264. [Crossref] [PubMed]
- Babjuk M, Burger M, Capoun O, et al. European Association of Urology Guidelines on Non-muscle-invasive Bladder Cancer (Ta, T1, and Carcinoma in Situ). Eur Urol 2022;81:75-94. [Crossref] [PubMed]
- Pearl R. Cancer and tuberculosis. American Journal of Epidemiology 1929;9:97-159. [Crossref]
- Bondaruk J, Jaksik R, Wang Z, et al. The origin of bladder cancer from mucosal field effects. iScience 2022;25:104551. [Crossref] [PubMed]
- Strandgaard T, Nordentoft I, Birkenkamp-Demtröder K, et al. Field Cancerization Is Associated with Tumor Development, T-cell Exhaustion, and Clinical Outcomes in Bladder Cancer. Eur Urol 2024;85:82-92. [Crossref] [PubMed]
- Jones TD, Wang M, Eble JN, et al. Molecular evidence supporting field effect in urothelial carcinogenesis. Clin Cancer Res 2005;11:6512-9. [Crossref] [PubMed]
- Lattime EC, Gomella LG, McCue PA. Murine bladder carcinoma cells present antigen to BCG-specific CD4+ T-cells. Cancer Res 1992;52:4286-90. [PubMed]
- Jackson AM, Alexandroff AB, Kelly RW, et al. Changes in urinary cytokines and soluble intercellular adhesion molecule-1 (ICAM-1) in bladder cancer patients after bacillus Calmette-Guérin (BCG) immunotherapy. Clin Exp Immunol 1995;99:369-75. [Crossref] [PubMed]
- De Boer EC, De Jong WH, Steerenberg PA, et al. Induction of urinary interleukin-1 (IL-1), IL-2, IL-6, and tumour necrosis factor during intravesical immunotherapy with bacillus Calmette-Guérin in superficial bladder cancer. Cancer Immunol Immunother 1992;34:306-12. [Crossref] [PubMed]
- Pichler R, Gruenbacher G, Culig Z, et al. Intratumoral Th2 predisposition combines with an increased Th1 functional phenotype in clinical response to intravesical BCG in bladder cancer. Cancer Immunol Immunother 2017;66:427-40. [Crossref] [PubMed]
- Nunez-Nateras R, Castle EP, Protheroe CA, et al. Predicting response to bacillus Calmette-Guérin (BCG) in patients with carcinoma in situ of the bladder. Urol Oncol 2014;32:45.e23-45.e4.5E30.
- Sylvester RJ, van der Meijden AP, Oosterlinck W, et al. Predicting recurrence and progression in individual patients with stage Ta T1 bladder cancer using EORTC risk tables: a combined analysis of 2596 patients from seven EORTC trials. Eur Urol 2006;49:466-5; discussion 475-7. [Crossref] [PubMed]
- van Rhijn BW, Burger M, Lotan Y, et al. Recurrence and progression of disease in non-muscle-invasive bladder cancer: from epidemiology to treatment strategy. Eur Urol 2009;56:430-42. [Crossref] [PubMed]
- Sylvester RJ, Rodríguez O, Hernández V, et al. European Association of Urology (EAU) Prognostic Factor Risk Groups for Non-muscle-invasive Bladder Cancer (NMIBC) Incorporating the WHO 2004/2016 and WHO 1973 Classification Systems for Grade: An Update from the EAU NMIBC Guidelines Panel. Eur Urol 2021;79:480-8. [Crossref] [PubMed]
- Lobo N, Hensley PJ, Bree KK, et al. Updated European Association of Urology (EAU) Prognostic Factor Risk Groups Overestimate the Risk of Progression in Patients with Non-muscle-invasive Bladder Cancer Treated with Bacillus Calmette-Guérin. Eur Urol Oncol 2022;5:84-91. [Crossref] [PubMed]
- Contieri R, Hurle R, Paciotti M, et al. Accuracy of the European Association of Urology (EAU) NMIBC 2021 scoring model in predicting progression in a large cohort of HG T1 NMIBC patients treated with BCG. Minerva Urol Nephrol 2023;75:180-7. [Crossref] [PubMed]
- Fernandez-Gomez J, Madero R, Solsona E, et al. Predicting nonmuscle invasive bladder cancer recurrence and progression in patients treated with bacillus Calmette-Guerin: the CUETO scoring model. J Urol 2009;182:2195-203. [Crossref] [PubMed]
- Lamm DL, Blumenstein BA, Crissman JD, et al. Maintenance bacillus Calmette-Guerin immunotherapy for recurrent TA, T1 and carcinoma in situ transitional cell carcinoma of the bladder: a randomized Southwest Oncology Group Study. J Urol 2000;163:1124-9. [Crossref] [PubMed]
- Xylinas E, Kent M, Kluth L, et al. Accuracy of the EORTC risk tables and of the CUETO scoring model to predict outcomes in non-muscle-invasive urothelial carcinoma of the bladder. Br J Cancer 2013;109:1460-6. [Crossref] [PubMed]
- van Rhijn BW. Combining molecular and pathologic data to prognosticate non-muscle-invasive bladder cancer. Urol Oncol 2012;30:518-23. [Crossref] [PubMed]
- Ferro M, Barone B, Crocetto F, et al. Predictive clinico-pathological factors to identify BCG, unresponsive patients, after re-resection for T1 high grade non-muscle invasive bladder cancer. Urol Oncol 2022;40:490.e13-20. [Crossref] [PubMed]
- Lopez-Beltran A, Cheng L. Stage T1 bladder cancer: diagnostic criteria and pitfalls. Pathology 2021;53:67-85. [Crossref] [PubMed]
- Bostwick DG, Ramnani D, Cheng L. Diagnosis and grading of bladder cancer and associated lesions. Urol Clin North Am 1999;26:493-507. [Crossref] [PubMed]
- Fransen van de Putte EE, Behrendt MA, Pigot GL, et al. Prognostic significance of substage and WHO classification systems in T1 urothelial carcinoma of the bladder. Curr Opin Urol 2015;25:427-35. [Crossref] [PubMed]
- Rouprêt M, Seisen T, Compérat E, et al. Prognostic interest in discriminating muscularis mucosa invasion (T1a vs T1b) in nonmuscle invasive bladder carcinoma: French national multicenter study with central pathology review. J Urol 2013;189:2069-76. [Crossref] [PubMed]
- Orsola A, Werner L, de Torres I, et al. Reexamining treatment of high-grade T1 bladder cancer according to depth of lamina propria invasion: a prospective trial of 200 patients. Br J Cancer 2015;112:468-74. [Crossref] [PubMed]
- van der Aa MN, van Leenders GJ, Steyerberg EW, et al. A new system for substaging pT1 papillary bladder cancer: a prognostic evaluation. Hum Pathol 2005;36:981-6. [Crossref] [PubMed]
- Fransen van de Putte EE, Otto W, Hartmann A, et al. Metric substage according to micro and extensive lamina propria invasion improves prognostics in T1 bladder cancer. Urol Oncol 2018;36:361.e7-361.e13. [Crossref] [PubMed]
- Fukumoto K, Kikuchi E, Mikami S, et al. Tumor budding, a novel prognostic indicator for predicting stage progression in T1 bladder cancers. Cancer Sci 2016;107:1338-44. [Crossref] [PubMed]
- Koelzer VH, Zlobec I, Lugli A. Tumor budding in colorectal cancer--ready for diagnostic practice? Hum Pathol 2016;47:4-19. [Crossref] [PubMed]
- Salhia B, Trippel M, Pfaltz K, et al. High tumor budding stratifies breast cancer with metastatic properties. Breast Cancer Res Treat 2015;150:363-71. [Crossref] [PubMed]
- Kadota K, Nitadori J, Woo KM, et al. Comprehensive pathological analyses in lung squamous cell carcinoma: single cell invasion, nuclear diameter, and tumor budding are independent prognostic factors for worse outcomes. J Thorac Oncol 2014;9:1126-39. [Crossref] [PubMed]
- Feitosa SG, Oliveira RV, Bezerra TMM, et al. Tumor Budding and Poor Prognosis in Oral Cancer: A Systematic Review and Meta-Analysis. Asian Pac J Cancer Prev 2023;24:2565-73. [Crossref] [PubMed]
- Eckstein M, Kimmel C, Bruendl J, et al. Tumor budding correlates with tumor invasiveness and predicts worse survival in pT1 non-muscle-invasive bladder cancer. Sci Rep 2021;11:17981. [Crossref] [PubMed]
- Borhani S, Borhani R, Kajdacsy-Balla A. Artificial intelligence: A promising frontier in bladder cancer diagnosis and outcome prediction. Crit Rev Oncol Hematol 2022;171:103601. [Crossref] [PubMed]
- Echle A, Rindtorff NT, Brinker TJ, et al. Deep learning in cancer pathology: a new generation of clinical biomarkers. Br J Cancer 2021;124:686-96. [Crossref] [PubMed]
- Skrede OJ, De Raedt S, Kleppe A, et al. Deep learning for prediction of colorectal cancer outcome: a discovery and validation study. Lancet 2020;395:350-60. [Crossref] [PubMed]
- Saillard C, Schmauch B, Laifa O, et al. Predicting Survival After Hepatocellular Carcinoma Resection Using Deep Learning on Histological Slides. Hepatology 2020;72:2000-13. [Crossref] [PubMed]
- Kulkarni PM, Robinson EJ, Sarin Pradhan J, et al. Deep Learning Based on Standard H&E Images of Primary Melanoma Tumors Identifies Patients at Risk for Visceral Recurrence and Death. Clin Cancer Res 2020;26:1126-34. [Crossref] [PubMed]
- Jansen I, Lucas M, Bosschieter J, et al. Automated Detection and Grading of Non-Muscle-Invasive Urothelial Cell Carcinoma of the Bladder. Am J Pathol 2020;190:1483-90. [Crossref] [PubMed]
- Tokuyama N, Saito A, Muraoka R, et al. Prediction of non-muscle invasive bladder cancer recurrence using machine learning of quantitative nuclear features. Mod Pathol 2022;35:533-8. [Crossref] [PubMed]
- Rouanne M, Betari R, Radulescu C, et al. Stromal lymphocyte infiltration is associated with tumour invasion depth but is not prognostic in high-grade T1 bladder cancer. Eur J Cancer 2019;108:111-9. [Crossref] [PubMed]
- Hülsen S, Lippolis E, Ferrazzi F, et al. High Stroma T-Cell Infiltration is Associated with Better Survival in Stage pT1 Bladder Cancer. Int J Mol Sci 2020;21:8407. [Crossref] [PubMed]
- Mano R, Baniel J, Shoshany O, et al. Neutrophil-to-lymphocyte ratio predicts progression and recurrence of non-muscle-invasive bladder cancer. Urol Oncol 2015;33:67.e1-7. [Crossref] [PubMed]
- D'Andrea D, Moschini M, Gust K, et al. Prognostic Role of Neutrophil-to-Lymphocyte Ratio in Primary Non-muscle-invasive Bladder Cancer. Clin Genitourin Cancer 2017;15:e755-64. [Crossref] [PubMed]
- Ślusarczyk A, Zapała P, Zapała Ł, et al. Prediction of BCG responses in non-muscle-invasive bladder cancer in the era of novel immunotherapeutics. Int Urol Nephrol 2019;51:1089-99. [Crossref] [PubMed]
- Cedrés S, Torrejon D, Martínez A, et al. Neutrophil to lymphocyte ratio (NLR) as an indicator of poor prognosis in stage IV non-small cell lung cancer. Clin Transl Oncol 2012;14:864-9. [Crossref] [PubMed]
- Yang JJ, Hu ZG, Shi WX, et al. Prognostic significance of neutrophil to lymphocyte ratio in pancreatic cancer: a meta-analysis. World J Gastroenterol 2015;21:2807-15. [Crossref] [PubMed]
- Miyamoto R, Inagawa S, Sano N, et al. The neutrophil-to-lymphocyte ratio (NLR) predicts short-term and long-term outcomes in gastric cancer patients. Eur J Surg Oncol 2018;44:607-12. [Crossref] [PubMed]
- Demir CC, Demir ME. The impact of smoking on inflammation indices: A cross-sectional study. Medicine Science 2023;12:347-51. [Crossref]
- Komiyama M, Ozaki Y, Miyazaki Y, et al. Neutrophil/lymphocyte ratio is correlated with levels of inflammatory markers and is significantly reduced by smoking cessation. J Int Med Res 2021;49:3000605211019223. [Crossref] [PubMed]
- Nassar AH, Umeton R, Kim J, et al. Mutational Analysis of 472 Urothelial Carcinoma Across Grades and Anatomic Sites. Clin Cancer Res 2019;25:2458-70. [Crossref] [PubMed]
- Meeks JJ, Carneiro BA, Pai SG, et al. Genomic characterization of high-risk non-muscle invasive bladder cancer. Oncotarget 2016;7:75176-84. [Crossref] [PubMed]
- Pietzak EJ, Bagrodia A, Cha EK, et al. Next-generation Sequencing of Nonmuscle Invasive Bladder Cancer Reveals Potential Biomarkers and Rational Therapeutic Targets. Eur Urol 2017;72:952-9. [Crossref] [PubMed]
- Bacon JVW, Müller DC, Ritch E, et al. Somatic Features of Response and Relapse in Non-muscle-invasive Bladder Cancer Treated with Bacillus Calmette-Guérin Immunotherapy. Eur Urol Oncol 2022;5:677-86. [Crossref] [PubMed]
- Sanguedolce F, Cormio A, Massenio P, et al. Altered expression of HER-2 and the mismatch repair genes MLH1 and MSH2 predicts the outcome of T1 high-grade bladder cancer. J Cancer Res Clin Oncol 2018;144:637-44. [Crossref] [PubMed]
- Bellmunt J, Kim J, Reardon B, et al. Genomic Predictors of Good Outcome, Recurrence, or Progression in High-Grade T1 Non-Muscle-Invasive Bladder Cancer. Cancer Res 2020;80:4476-86. [Crossref] [PubMed]
- Hurst CD, Alder O, Platt FM, et al. Genomic Subtypes of Non-invasive Bladder Cancer with Distinct Metabolic Profile and Female Gender Bias in KDM6A Mutation Frequency. Cancer Cell 2017;32:701-715.e7. [Crossref] [PubMed]
- Robertson AG, Groeneveld CS, Jordan B, et al. Identification of Differential Tumor Subtypes of T1 Bladder Cancer. Eur Urol 2020;78:533-7. [Crossref] [PubMed]
- de Jong FC, Laajala TD, Hoedemaeker RF, et al. Non-muscle-invasive bladder cancer molecular subtypes predict differential response to intravesical Bacillus Calmette-Guérin. Sci Transl Med 2023;15:eabn4118. [Crossref] [PubMed]
- Kim YJ, Ha YS, Kim SK, et al. Gene signatures for the prediction of response to Bacillus Calmette-Guerin immunotherapy in primary pT1 bladder cancers. Clin Cancer Res 2010;16:2131-7. [Crossref] [PubMed]
- Goel A, Ward DG, Noyvert B, et al. Combined exome and transcriptome sequencing of non-muscle-invasive bladder cancer: associations between genomic changes, expression subtypes, and clinical outcomes. Genome Med 2022;14:59. [Crossref] [PubMed]
- Lindskrog SV, Prip F, Lamy P, et al. An integrated multi-omics analysis identifies prognostic molecular subtypes of non-muscle-invasive bladder cancer. Nat Commun 2021;12:2301. [Crossref] [PubMed]
- Strandgaard T, Lindskrog SV, Nordentoft I, et al. Elevated T-cell Exhaustion and Urinary Tumor DNA Levels Are Associated with Bacillus Calmette-Guérin Failure in Patients with Non-muscle-invasive Bladder Cancer. Eur Urol 2022;82:646-56. [Crossref] [PubMed]
- Kavalieris L, O'Sullivan P, Frampton C, et al. Performance Characteristics of a Multigene Urine Biomarker Test for Monitoring for Recurrent Urothelial Carcinoma in a Multicenter Study. J Urol 2017;197:1419-26. [Crossref] [PubMed]
- Elsawy AA, Awadalla A, Elsayed A, et al. Prospective Validation of Clinical Usefulness of a Novel mRNA-based Urine Test (Xpert® Bladder Cancer Monitor) for surveillance in Non Muscle Invasive Bladder Cancer. Urol Oncol 2021;39:77.e9-77.e16. [Crossref] [PubMed]
- Liem EIML, Baard J, Cauberg ECC, et al. Fluorescence in situ hybridization as prognostic predictor of tumor recurrence during treatment with Bacillus Calmette-Guérin therapy for intermediate- and high-risk non-muscle-invasive bladder cancer. Med Oncol 2017;34:172. [Crossref] [PubMed]
- Kojima T, Nishiyama H, Ozono S, et al. Clinical evaluation of two consecutive UroVysion fluorescence in situ hybridization tests to detect intravesical recurrence of bladder cancer: a prospective blinded comparative study in Japan. Int J Clin Oncol 2018;23:1140-7. [Crossref] [PubMed]
- Witjes JA, Morote J, Cornel EB, et al. Performance of the Bladder EpiCheck™ Methylation Test for Patients Under Surveillance for Non-muscle-invasive Bladder Cancer: Results of a Multicenter, Prospective, Blinded Clinical Trial. Eur Urol Oncol 2018;1:307-13. [Crossref] [PubMed]
- Nikas IP, Seide S, Proctor T, et al. The Paris System for Reporting Urinary Cytology: A Meta-Analysis. J Pers Med 2022;12:170. [Crossref] [PubMed]
- Kamat AM, Briggman J, Urbauer DL, et al. Cytokine Panel for Response to Intravesical Therapy (CyPRIT): Nomogram of Changes in Urinary Cytokine Levels Predicts Patient Response to Bacillus Calmette-Guérin. Eur Urol 2016;69:197-200. Erratum in: Eur Urol 2016;70:e26. [Crossref] [PubMed]
- Luo Y, Chen X, O'Donnell MA. Role of Th1 and Th2 cytokines in BCG-induced IFN-gamma production: cytokine promotion and simulation of BCG effect. Cytokine 2003;21:17-26. [Crossref] [PubMed]
- Saint F, Kurth N, Maille P, et al. Urinary IL-2 assay for monitoring intravesical bacillus Calmette-Guérin response of superficial bladder cancer during induction course and maintenance therapy. Int J Cancer 2003;107:434-40. [Crossref] [PubMed]
- Nadler R, Luo Y, Zhao W, et al. Interleukin 10 induced augmentation of delayed-type hypersensitivity (DTH) enhances Mycobacterium bovis bacillus Calmette-Guérin (BCG) mediated antitumour activity. Clin Exp Immunol 2003;131:206-16. [Crossref] [PubMed]
- Elsawy AA, Abol-Enein H, Laymon M, et al. Predictive value of immunological markers after bacille Calmette-Guérin induction in bladder cancer. BJU Int 2022;130:444-53. [Crossref] [PubMed]
- Sagnak L, Ersoy H, Ozok U, et al. Predictive value of urinary interleukin-8 cutoff point for recurrences after transurethral resection plus induction bacillus Calmette-Guérin treatment in non-muscle-invasive bladder tumors. Clin Genitourin Cancer 2009;7:E16-23. [Crossref] [PubMed]
- Cai T, Mazzoli S, Meacci F, et al. Interleukin-6/10 ratio as a prognostic marker of recurrence in patients with intermediate risk urothelial bladder carcinoma. J Urol 2007;178:1906-11; discussion 1911-2. [Crossref] [PubMed]
- Cai T, Nesi G, Mazzoli S, et al. Prediction of response to bacillus Calmette-Guérin treatment in non-muscle invasive bladder cancer patients through interleukin-6 and interleukin-10 ratio. Exp Ther Med 2012;4:459-64. [Crossref] [PubMed]
- Murakami K, Kamat AM, Dai Y, et al. Application of a multiplex urinalysis test for the prediction of intravesical BCG treatment response: A pilot study. Cancer Biomark 2022;33:151-7. [Crossref] [PubMed]
- Shariat SF, Ashfaq R, Sagalowsky AI, et al. Predictive value of cell cycle biomarkers in nonmuscle invasive bladder transitional cell carcinoma. J Urol 2007;177:481-7; discussion 487. [Crossref] [PubMed]
- Esuvaranathan K, Chiong E, Thamboo TP, et al. Predictive value of p53 and pRb expression in superficial bladder cancer patients treated with BCG and interferon-alpha. Cancer 2007;109:1097-105. [Crossref] [PubMed]
- Asakura T, Takano Y, Iki M, et al. Prognostic value of Ki-67 for recurrence and progression of superficial bladder cancer. J Urol 1997;158:385-8. [Crossref] [PubMed]
- Lebret T, Watson RW, Molinié V, et al. HSP90 expression: a new predictive factor for BCG response in stage Ta-T1 grade 3 bladder tumours. Eur Urol 2007;51:161-6; discussion 166-7. [Crossref] [PubMed]
- Videira PA, Calais FM, Correia M, et al. Efficacy of bacille Calmette-Guérin immunotherapy predicted by expression of antigen-presenting molecules and chemokines. Urology 2009;74:944-50. [Crossref] [PubMed]
- Cormio L, Sanguedolce F, Cormio A, et al. Human epidermal growth factor receptor 2 expression is more important than Bacillus Calmette Guerin treatment in predicting the outcome of T1G3 bladder cancer. Oncotarget 2017;8:25433-41. [Crossref] [PubMed]
- Ayari C, LaRue H, Hovington H, et al. Bladder tumor infiltrating mature dendritic cells and macrophages as predictors of response to bacillus Calmette-Guérin immunotherapy. Eur Urol 2009;55:1386-95. [Crossref] [PubMed]
- Takayama H, Nishimura K, Tsujimura A, et al. Increased infiltration of tumor associated macrophages is associated with poor prognosis of bladder carcinoma in situ after intravesical bacillus Calmette-Guerin instillation. J Urol 2009;181:1894-900. [Crossref] [PubMed]
- Miyake M, Tatsumi Y, Gotoh D, et al. Regulatory T Cells and Tumor-Associated Macrophages in the Tumor Microenvironment in Non-Muscle Invasive Bladder Cancer Treated with Intravesical Bacille Calmette-Guérin: A Long-Term Follow-Up Study of a Japanese Cohort. Int J Mol Sci 2017;18:2186. [Crossref] [PubMed]
- Dowell AC, Cobby E, Wen K, et al. Interleukin-17-positive mast cells influence outcomes from BCG for patients with CIS: Data from a comprehensive characterisation of the immune microenvironment of urothelial bladder cancer. PLoS One 2017;12:e0184841. [Crossref] [PubMed]
- Pichler R, Fritz J, Zavadil C, et al. Tumor-infiltrating immune cell subpopulations influence the oncologic outcome after intravesical Bacillus Calmette-Guérin therapy in bladder cancer. Oncotarget 2016;7:39916-30. [Crossref] [PubMed]
- Lim CJ, Nguyen PHD, Wasser M, et al. Immunological Hallmarks for Clinical Response to BCG in Bladder Cancer. Front Immunol 2021;11:615091. [Crossref] [PubMed]
- Kates M, Matoso A, Choi W, et al. Adaptive Immune Resistance to Intravesical BCG in Non-Muscle Invasive Bladder Cancer: Implications for Prospective BCG-Unresponsive Trials. Clin Cancer Res 2020;26:882-91. [Crossref] [PubMed]
- Salomé B, Sfakianos JP, Ranti D, et al. NKG2A and HLA-E define an alternative immune checkpoint axis in bladder cancer. Cancer Cell 2022;40:1027-1043.e9. [Crossref] [PubMed]
- Cheng X, Zhao Z, Ventura E, et al. The PD-1/PD-L pathway is up-regulated during IL-12-induced suppression of EAE mediated by IFN-gamma. J Neuroimmunol 2007;185:75-86. [Crossref] [PubMed]
- Larsson L, Frisén J, Lundeberg J. Spatially resolved transcriptomics adds a new dimension to genomics. Nat Methods 2021;18:15-8. [Crossref] [PubMed]
- García-Cuesta EM, Esteso G, Ashiru O, et al. Characterization of a human anti-tumoral NK cell population expanded after BCG treatment of leukocytes. Oncoimmunology 2017;6:e1293212. [Crossref] [PubMed]
- Esteso G, Felgueres MJ, García-Jiménez ÁF, et al. BCG-activation of leukocytes is sufficient for the generation of donor-independent innate anti-tumor NK and γδ T-cells that can be further expanded in vitro. Oncoimmunology 2022;12:2160094. [Crossref] [PubMed]