Management of translocation carcinomas of the kidney
Introduction
Microphthalmia-associated transcription factor family translocation renal cell carcinoma (MiT-tRCC) is a rare kidney cancer subtype that includes TFE3-rearranged RCC (also known as Xp11 translocation RCC) and TFE-rearranged translocations RCC. It constitutes approximately 1.6–4% of RCC cases in adults and approximately 40% of cases in children (1-3). It is characterized by female predominance and the average age of onset in adult tRCCs is 40 years (2). Due to the morphological overlap with other RCCs, the incidence of tRCCs in adults is probably underestimated to what has been reported thus far (4). There are limited known risk factors; however, prior exposure to cytotoxic chemotherapy is a risk factor for developing tRCC (5).
The characteristic genetic events are chromosomic translocations that affect the short arm of the X chromosome, activating gene fusions involving the MiT family of transcription factors, such as TFE3, TFEB, TFEC, and MiTF; however, new genes, fusion partners, and morphological phenotypes are being discovered, making the diagnosis of tRCC more challenging (6,7). It was initially recognized in the 2004 World Health Organization (WHO) classification of renal tumors as Xp11 translocation RCC before the nomenclature was changed to MITF/TFE translocation renal carcinoma in the 2016 WHO classification (8). Recently, TFE3 (Xp11) rearrangement and TFEB (6p21) alterations were included in the WHO 2022 “molecularly defined renal carcinomas” as a distinct group (9). Although TFE3-rearranged RCC and TFEB-rearranged RCC are grouped into one subtype, they are biologically distinct, exhibiting diverse clinical outcomes and presentations. TFEB-rearranged RCCs are generally rarer than TFE3-rearranged RCCs, with fewer than 100 cases reported in the literature. These tumors are typically characterized by low-grade and biphasic morphology, exhibiting indolent behavior and a favorable prognosis (7).
In this review, we provide an overview of the diagnostic and molecular features of tRCC and discuss the medical management of advanced tRCC, including recent data on the use of immune checkpoint inhibitors (ICIs).
Diagnosis
There are no specific clinical features that distinguish tRCC from other RCC subtypes. Like other RCCs, most tRCCs are asymptomatic at presentation and are typically incidentally discovered on imaging, with no specific radiological features differing from other RCC subtypes. The only identified risk factor for the development of tRCC is prior exposure to cytotoxic chemotherapy. The diagnosis of tRCC is primarily made by a combination of histology, immunohistochemistry (IHC), and genetic testing findings (10-12). There is a resemblance between the wide spectrum of morphologies of tRCC and other more frequent RCC subtypes, such as clear cell (ccRCC) and papillary (pRCC), which can make the diagnosis challenging (4,13). This may also have an impact on the stated incidence rate of tRCC, leading to inaccurate frequency due to the confusion with other RCC subtypes (4). It is important to take tRCC into consideration in all unusual RCC cases, especially the ones occurring in children and young adults (10).
Microscopic examination reveals characteristic features, including clear or eosinophilic cytoplasm and distinct architectural patterns such as papillary or alveolar structures. The most common pattern is the papillary architecture with psammoma bodies, which can aid pathologists in differentiating tRCC from other RCC subtypes (13-15). Figure 1 illustrates TFE3-rearranged tRCC mimicking ccRCC with voluminous clear cytoplasm and psammomatous calcification.
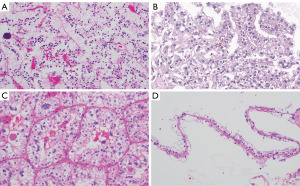
Using IHC staining, these tumors express renal tubular markers such as PAX8, cathepsin K, in addition to strong TFE3 immunostaining (16,17). However, TFE3 immunostaining can be associated with false-positive and false-negative results and therefore the identification of the TFE3 rearrangement by TFE3 break-apart fluorescence in situ hybridization (FISH) assays are the gold standard for the diagnosis of TFE3-rearranged RCCs (18). In selective cases, RNA sequencing or reverse transcription polymerase chain reaction can be used to aid the diagnosis (19).
Molecular and genetic characteristics
The characteristic pathognomonic events in MiT-tRCC are chromosomic translocations that affect the short arm of the X chromosome, and fusions involving the MiT family of transcription factors, such as TFE3, TFEB, TFEC, and MiTF (6,7). The presence of co-occurring genetic alterations is still unclear (11,20). These tumors exhibit a fusion of the TFE3 factor gene with one of several additional genes by translocation, with the most common partners being: PRCC in the t(X;1)(p11.2;q21), ASPSCR1 in the t(X;17)(p11.2;q25), and SFQP in the t(X;1)(p11.2;p34) (21). The diagnosis is generally made with the nuclear expression of TFE3 by IHC and confirmed by FISH given the high false positivity of IHC studies in this setting. RNA sequencing might help identify fusion partners involved in translocations (7).
With the advent of high-throughput sequencing techniques, multiple studies were conducted in recent years to characterize these tumors at the molecular level (22). Although these studies were limited by the small sample size, they have yielded crucial insights and enhanced our comprehension of the molecular attributes associated with these tumors. It is also important to note that very limited number of patients with TFEB-rearranged RCC were included in the molecular studies of tRCC, as such, these data mostly pertain to TFE3-rearranged RCC.
The tumor mutational burden (TMB) has emerged as a biomarker of clinical benefit to immunotherapy in several solid tumors (23,24). Studies examining the TMB in tRCC demonstrated that these tumors are generally gnomically quiet and exhibit a low TMB (20,25). Notably, the TMB in tRCC is significantly lower than that observed in ccRCC and pRCC when compared in the KIRC and KIRP cohorts within The Cancer Genome Atlas (TCGA) cohort.
Furthermore, multiple previous studies revealed that these tumors are characterized by high copy number alterations (11,20,25). A study of 16 patients with tRCC found heterogeneous molecular profiles, with 17q gain and 9p loss as the most frequent copy number variants and the presence of 17q gain was indicative of poor overall survival (OS) outcomes but the presence of 9p loss was not significantly associated with poor OS (11). Another study from the Memorial Sloan Kettering Cancer Center (MSKCC) using MSK-IMPACT sequencing test in 22 patients with tRCC also revealed that these tumors have high copy number alterations, including loss of 9p in 9 of 22 (41%) patients, gain of 17q in 8 of 22 (36%) patients, and gain of 6p in 8 of 22 (36%) patients (20). In terms of clinical outcomes and OS in this cohort, patients with either 9p loss, 17q gain, or a high fraction of the genome exhibiting any copy number alteration (FCNAg) had worse OS compared to patients without these copy number changes.
A large sequencing study by Bakouny et al. reported on a comprehensive integrative analysis of 152 patients with tRCC identified across genomic, clinical trial, and retrospective cohorts (25). Similar findings were identified, including a lower TMB and high copy number alterations in these tumors, in addition to homozygous deletions at chromosome 9p21.3 (19.2% of cases). At the transcriptome level, these tumors displayed unique features, including an antioxidant signature through the activation of the NRF2 pathway.
Molecular analysis from the IMmotion 151 phase III clinical trials in ccRCC comparing atezolizumab plus bevacizumab versus sunitinib using bulk RNA sequencing and targeted mutation sequencing identified seven transcriptionally defined molecular clusters which were associated with unique molecular features and differential responses to ICI and anti-angiogenic therapies (26). A total of 15 patients in cluster 5 (proliferative cluster) were found to have tRCC. This cluster was characterized by a low rate of VHL mutation, lower angiogenesis signatures, and low PD-L1 expression.
At the proteome level, enrichment analysis unveiled the upregulation of various processes in tRCC, including neutrophil degranulation, insulin signaling pathway, glycogen metabolism, membrane trafficking, and lysosomal function. Furthermore, there is a notable upregulation of the mammalian target of rapamycin (mTOR) signaling pathway in tRCC, observed both at the proteome and phosphoproteome levels (27).
Management
There have been significant advances in the treatment landscape of RCC over the last three decades which is driven by a deeper understanding of RCC biology and the discovery of targetable pathways (28). In the localized setting, partial or radical nephrectomy with curative intent remains the standard of care for localized disease, including those cases with positive regional lymph nodes, with favorable outcomes (29). This is the same standard of care for patients with ccRCC and non-ccRCC histologies, including tRCC (29).
In the metastatic setting, the systemic treatment for RCC has evolved from cytokine-based treatments to targeted therapies with vascular endothelial growth factor targeted therapies (VEGF-TT), and mTOR inhibitors (mTORi) to novel immunotherapy agents in the form of ICI targeting the programmed death receptor 1 (PD-1) or its ligand (PD-L1) and CTL-associated protein 4 (CTLA-4) (28,30). These treatments were mostly studied and approved for use in patients with ccRCC (31). Historically, it has been challenging to conduct large studies in patients with metastatic non-ccRCC given the difficulties in enrollment on those studies and the limited understanding of the biology of these rare tumors (32). However, recent data from multiple retrospective studies and prospective clinical trial data of standard ccRCC systemic therapies in non-ccRCC variants, including tRCC have demonstrated some activity of these treatments in particular to ICI combinations. Despite this, they are still insufficient to be accepted as standard of care therapies with high level of evidence (33). Additionally, the prospective data for metastatic tRCC treatment are limited, further complicating the establishment of definitive treatment protocols. Notably, considering the rarity of TFEB-rearranged RCC, most of the data on the activity of systemic therapy pertains to TFE3-rearranged RCC.
VEGF inhibitors activity in translocation RCC
VEGF-tyrosine kinase inhibitors (VEGF-TKIs) are well-established cornerstone therapies in ccRCC and have been investigated in multiple studies in non-ccRCC variants (33,34). Table 1 summarizes the key efficacy data for VEGF-TKI in metastatic tRCC.
Table 1
Reference | Treatment | Number of patients | Line of treatment | ORR (%) | Median PFS (months) | Median OS (months) |
---|---|---|---|---|---|---|
Malouf et al. (35) | Sunitinib | 11 | 1 | 36 | 8.2 | NR |
Bakouny et al. (25) | Sunitinib, pazopanib | 10 | ≥1 | 0 | NR | 10.3 |
Choueiri et al. (36) | Sunitinib, sorafenib, VEGF mAB | 15 | ≥1 | 20 | 7.1 | 14.3 |
Boilève et al. (37) | Sunitinib, pazopanib, sorafenib | 19 | 1 | 10.5 | 3 | NR |
Thouvenin et al. (38) | Cabozantinib | 52 | ≥1 | 17.3 | 6.8 | 18.3 |
Tannir et al. (39) | Sunitinib | 3 | 1 | NR | 6.1 | 16.2 |
VEGF, vascular endothelial growth factor; ORR, objective response rate; PFS, progression-free survival; OS, overall survival; mAB, monoclonal antibody; NR, not reported.
In a study of 23 patients with metastatic tRCC treated with multiple systemic agents at multiple lines of therapy, including sunitinib, sorafenib, cytokines, and mTOR inhibitor; patients who received VEGF-TKIs had better outcomes (35). A total of 11 patients received first-line sunitinib and 9 patients received a cytokine-based regimen with a median progression-free survival (PFS) of 8.2 months in the sunitinib group [95% confidence interval (CI): 2.6–14.7 months] versus 2 months in the cytokine group (95% CI: 0.8–3.3 months) (log-rank P=0.003). The objective response rate (ORR) was 36% in the sunitinib group (3 partial responses and 1 complete response) in comparison to 11% in the cytokine group (1 partial response).
Another retrospective study reported on treatment outcomes in a cohort of 15 patients with metastatic tRCC treated with VEGF inhibitors, including sunitinib (N=10), sorafenib (N=3), VEGF monoclonal antibodies (n=2) received at multiple lines of therapy with an ORR of 20%, median PFS of 7.1 months (95% CI: 1.7–27 months) and median OS of 14.3 months (95% CI: 2.7–not reached) (36).
Boilève et al. reported on a multicenter retrospective study of patients with metastatic tRCC treated in France and the USA, comparing outcomes of ICI and VEGF-TKIs (37). In this study, a total of 19 patients were treated with first-line VEGF-TKI (sunitinib =14, pazopanib =4, and sorafenib =1) with a median PFS of 3 months (range, 1–22 months) and ORR of 10.5%.
A retrospective cohort of patients with metastatic tRCC identified within the International mRCC Database Consortium (IMDC) and Harvard datasets compared outcomes of patients with tRCC treated with VEGF-TKI versus ICIs and included ten patients who received VEGF-TKI at multiple lines (sunitinib =8, pazopanib =2). In this cohort, VEGF-TKI therapy was not associated with any objective responses and resulted in a median OS of 10.3 months (25).
Moving to contemporary VEGF-TKI agents, Thouvenin et al. reported a retrospective series on outcomes of 52 patients with tRCC who were treated with cabozantinib at different lines of therapy (38). The ORR was 17.3%, including two complete responses with a median PFS of 6.8 months (95% CI: 4.6–16.3 months) and median OS of 18.3 months (95% CI: 17.0–30.6 months).
There is limited data in the prospective setting for VEGF-TKI activity in metastatic tRCC. The ESPN study was a phase 2 randomized study of first-line sunitinib in comparison to everolimus in patients with metastatic non-ccRCC which included seven patients with metastatic tRCC (sunitinib =3, everolimus =4) (39). In the sunitinib group, the median PFS was 6.1 months (95% CI: 6.0–8.8 months) and the median OS was 16.2 months [95% CI: 8.8 months–not reached (NR)]. While in the everolimus group, the median PFS was 3.0 months (95% CI: 1.3 months–NR) and the median OS was 8.1 months (95% CI: 5.5–23 months) indicating better outcomes to sunitinib.
ICIs activity in translocation RCC
Novel immunotherapy agents in the form of ICI targeting the PD-1 or PD-L1 and CTLA-4 have revolutionized the management of metastatic RCC (28,40). Currently, per contemporary guidelines the recommended first-line treatment for patients with metastatic ccRCC are ICI combinations, either in the form of dual ICI combination with nivolumab plus ipilimumab or ICI plus VEGF-TKI combinations (31). In the realm of non-ccRCC, where PD-L1 has been shown to be expressed on both tumor cells and tumor-infiltrating mononuclear cells (41), the success of ICI therapies observed in ccRCC has prompted exploration in non-ccRCC, including tRCC, through both retrospective and prospective studies (33). Table 2 provides a concise summary of the key efficacy data for ICI therapies in metastatic tRCC.
Table 2
Reference | Treatment | Number of patients | Line of treatment | ORR (%) | Median PFS (months) | Median OS (months) |
---|---|---|---|---|---|---|
Boilève et al. (37) | Nivolumab, ipilimumab, others | 24 | ≥2 | 16.6 | 2.5 | NR |
Bakouny et al. (25) | Nivolumab, nivolumab + ipilimumab, others | 12 | ≥1 | 25 | NR | 62.4 |
Tykodi et al. (42) | Nivolumab + ipilimumab | 2 | 1 | 0 | NR | NR |
McGregor et al. (43) | Atezolizumab + bevacizumab | 5 | ≥1 | 20 | NR | NR |
Lee et al. (44) | Nivolumab + cabozantinib | 2 | 1 | 50 | NR | NR |
Albiges et al. (45) | Pembrolizumab + lenvatinib | 6 | 1 | 67 | NR | NR |
McGregor et al. (46) | Nivolumab + ipilimumab + cabozantinib | 5 | ≥1 | 0 | NR | NR |
Alhalabi et al. (47) | ICI + TKI, ICI + ICI | 29 | ≥1 | ICI + TKI (36) | ICI + TKI (5.4) | ICI + TKI (30.7) |
ICI + ICI (5.5) | ICI + ICI (2.8) | ICI + ICI (17.8) | ||||
Ged et al. (48) | ICI + TKI, ICI + ICI | 22 | ≥1 | ICI + TKI (54) | ICI + TKI (6.2) | ICI + TKI (15.6) |
ICI + ICI (5.5) | ICI + ICI (1.2) | ICI + ICI (36.7) |
ICI, immune-checkpoint inhibitors; ORR, objective response rate; PFS, progression-free survival; OS, overall survival; NR, not reported; TKI, tyrosine kinase inhibitor.
Boilève et al. retrospectively reviewed 24 patients receiving ICI in the second line or later including nivolumab (n=17), ipilimumab (n=3), and ICI-based combinations (n=4). The ORR to ICI in this cohort was 16.6% with a median PFS of 2.5 months (range, 1–40 months) (37). The outcomes to ICI in this cohort were modest, which may have been limited by the small sample size, and the use of ICI monotherapy in the second line and beyond setting.
A retrospective cohort of patients with metastatic tRCC identified within the IMDC and Harvard datasets included 12 patients who received ICI at multiple lines of therapy (nivolumab =6, nivolumab plus ipilimumab =4, atezolizumab plus bevacizumab =1, other ICI plus ICI combination =1) with ORR of 25% and median OS of 62.4 months (25). The outcomes of ICI in comparison to VEGF-TKIs in this cohort were considerably better.
The CheckMate 920 was a multicohort, phase 3b/4 of nivolumab plus ipilimumab in previously untreated metastatic RCC (42). The non-ccRCC cohort in the study included 52 patients, two of whom had tRCC. The ORR for all non-ccRCC patients in this cohort was 19.6%; however, none of the responses were observed in the tRCC patients.
A phase II single-arm trial evaluating the combination of atezolizumab plus bevacizumab in patients RCC with variant histology or any RCC histology with ≥20% sarcomatoid differentiation, included 60 patients, five of whom had tRCC with ORR of 20% (43).
The IMmotion151 phase 3 trial was a randomized study comparing atezolizumab plus bevacizumab versus sunitinib as first-line therapy in patients with metastatic ccRCC (49). RNA-sequencing performed on tumor biopsies from patients enrolled in this trial identified 15 patients with tRCC (9 patients received atezolizumab plus bevacizumab and 6 patients received sunitinib) (25,26,49). The patients in the sunitinib group had poor outcomes (median PFS of 3.5 months) in comparison to the 9 patients in the atezolizumab plus bevacizumab group (median PFS of 15.8 months).
Lee et al. reported on a two-cohort single-arm prospective study of nivolumab plus cabozantinib in patients with metastatic non-ccRCC (44). Cohort 1 of the study included a total of 40 patients with an ORR of 47.5% and median PFS of 12.5 months. There was a total of two patients with metastatic tRCC in cohort 1, with ORR seen in at least 1 patient (50%).
The KEYNOTE B61 was a single-arm study of pembrolizumab plus lenvatinib in previously untreated metastatic non-ccRCC which is the largest prospective study of ICI combination therapy in metastatic non-ccRCC thus far (45). The study enrolled 158 patients across 48 sites. With a median follow-up of 14.9 months (interquartile range, 11.1–17.4 months), 78 of 158 patients had an ORR (49%) with a median PFS of 18 months (95% CI: 14 months–NR). A total of 6 patients had tRCC with ORR of 67% to the combination of pembrolizumab plus lenvatinib.
The COSMIC-313 was a phase 3 trial comparing the triplet combination of nivolumab plus ipilimumab plus cabozantinib versus nivolumab plus ipilimumab as a first-line treatment in patients with intermediate or poor risk metastatic ccRCC and resulted in significant prolongation of the PFS in favor of the triplet combination of nivolumab plus ipilimumab plus cabozantinib (50). Building on the data of the COSMIC-313, the CaNI trial is a single arm phase 2 study of the triplet combination nivolumab plus ipilimumab plus cabozantinib in patients with metastatic non-ccRCC with the primary endpoint of ORR (46). A total of 39 patients were treated in the study, including 5 with metastatic tRCC. The overall ORR was 18%, and no ORR were seen in the five tRCC patients; however, all patients achieved stable disease as the best response at the time of the initial study report. The study was expanded to enroll an additional 20 patients (NCT0441312).
Alhalabi et al. reported on a multi-institutional cohort of 29 metastatic tRCC patients treated in the USA and France, comparing treatment outcomes of ICI plus ICI combinations to ICI plus VEGF-TKI combinations (47). In this study, a total of 18 and 11 patients received ICI plus ICI and ICI plus VEGF-TKI, respectively. Most patients (59%) received ICI combinations as first-line therapy. ICI plus VEGF-TKI combinations led to higher clinical activity with ORR of 36%, median PFS of 5.4 months and median OS of 30.7 months in comparison to ICI plus ICI combinations which resulted in ORR of 5.5%, median PFS of 2.8 months and median OS of 17.8 months. Another similar retrospective study included 22 metastatic tRCC patients treated with ICI combinations at any line of therapy, of which eight patients were treated with ICI plus ICI and 14 patients were treated with ICI plus VEGF-TKI (48). Similar higher efficacy in favor of ICI plus VEGF-TKI was observed with ORR of 54% and median PFS of 6.2 months in the ICI plus VEGF-TKI cohort in comparison to ORR of 14% and median PFS of 1.2 months in the ICI plus ICI group. However, the median OS was numerically longer in the ICI plus ICI group in comparison to the ICI plus VEGF-TKI group (36.7 versus 15.6 months) but this could have been impacted by the effects of subsequent systemic therapies and the small sample size.
The ongoing randomized phase II AREN172 clinical trial which is being conducted through the US cooperative groups (NCT03595124) is comparing nivolumab versus nivolumab plus axitinib in patients with metastatic tRCC and will provide important prospective randomized data. Unfortunately, the enrollment in this study has been heavily impacted by the limited number of patients with this disease, highlighting the significant limitation of conducting trials in rare diseases.
Based on cross data comparison of multiple small retrospective and prospective studies, the outcomes of ICI based combinations are superior to VEGF-TKI monotherapies with higher ORR and prolonged PFS and OS durations (Tables 1,2). Additionally, no unexpected toxicity signals were reported with the use of ICI and VEGF-TKI combinations that may be relevant to tRCC.
Future directions and novel therapies
The rarity of tRCC comes with its own challenges, which makes the overall management of tRCC difficult. Similar to other rare and orphan diseases, the lack of standardized therapies for advanced stages and of biomarkers leads to the lack of a definite treatment protocol, which should be supported by sufficient research proven data (51). Funding for clinical trials of rare cancers might be difficult to obtain, but even the next steps of finding enough patients to enroll in clinical trials are challenging. Nevertheless, the introduction of innovative high-throughput sequencing technologies, including single-cell sequencing, spatial transcriptomics, and proteomics, holds the promise of unveiling novel biological insights, potential biomarkers of treatment efficacy, and therapeutic targets (22,52). These advanced techniques have the potential to revolutionize our understanding of the molecular landscape, paving the way for the identification of previously undiscovered pathways and potential targets for therapeutic intervention.
Zanzalintinib (XL092) is a novel next-generation TKI presently under investigation in numerous clinical trials for RCC, both as a standalone treatment and in conjunction with various ICIs, such as anti-PD-1, anti-CTLA-4, and anti-LAG3 antibodies. One of these ongoing studies is the STELLAR-02 study, a phase 1b study of zanzalintinib and ICI combinations (NCT05176483) which allows the enrollment of patients with non-ccRCC, including tRCC and will provide further insight of the activity of novel ICI and targeted therapy combinations activity in tRCC (53,54).
Glycoprotein nonmetastatic B (GPNMB) is a transmembrane protein which is known to express at high levels in histiocytes and several malignancies, including malignant melanoma, breast cancer, and gliomas (55,56). It is regarded as a surrogate marker for alterations involving the TSC1/2/mTOR pathway (57). tRCC exhibits significantly higher expression levels compared to ccRCC in pre-clinical studies (58). This finding suggests a potential role for GPNMB as a screening marker or therapeutic target in the context of tRCC. Furthermore, in an effort to uncover new therapeutic targets, a recent study reported on high-throughput drug screening in TFE3-RCC tumor-derived cell line (59). This screening revealed promising candidates, including PI3K/mTOR inhibitor NVP-BGT226, the transcription inhibitor mithramycin A, and GPNMB-targeted antibody-drug conjugate CDX-011 as potential therapeutic targets. These findings further support the potential role of GPNMB in tRCC.
Prostate-specific membrane antigen (PSMA) is a type II membrane protein that is highly expressed in the prostate cancer cells epithelium which has been the imaging and therapeutic radioligand agents (60). However, it is also known to be highly expressed in the neovasculature of ccRCC (61). A recent study examined the vascular expression of PSMA by IHC in multiple RCC subtypes and demonstrated that the highest expression is in ccRCC (88%) followed by tRCC (71%) which may also provide another potential imaging or therapeutic target (62).
Conclusions
The discovery and approval of novel ICI combination therapies have significantly enhanced treatment outcomes for RCC patients, including those with tRCC. The ongoing evolution of combination strategies that synergize between these therapeutic modalities or other drug classes holds promise for further advancements, expanding the array of first-line therapeutic options. Simultaneously, progress in genomic sequencing and molecular characterizations has broadened the scope of disease prognostication, enabling more precise assessments. Nonetheless, there remains a critical need for predictive biomarkers to inform clinical decision-making. Diagnostic tools that integrate biomarker data have the potential to customize treatment plans based on distinct biological features and should be developed alongside new treatments. Collaborative research efforts continue to be vital for establishing standardized therapies for advanced stage tRCC.
Acknowledgments
We acknowledge the patients and their families who contributed to translocation renal cell carcinoma studies.
Funding: None.
Footnote
Peer Review File: Available at https://tcr.amegroups.com/article/view/10.21037/tcr-24-60/prf
Conflicts of Interest: All authors have completed the ICMJE uniform disclosure form (available at https://tcr.amegroups.com/article/view/10.21037/tcr-24-60/coif). The authors have no conflicts of interest to declare.
Ethical Statement: The authors are accountable for all aspects of the work in ensuring that questions related to the accuracy or integrity of any part of the work are appropriately investigated and resolved.
Open Access Statement: This is an Open Access article distributed in accordance with the Creative Commons Attribution-NonCommercial-NoDerivs 4.0 International License (CC BY-NC-ND 4.0), which permits the non-commercial replication and distribution of the article with the strict proviso that no changes or edits are made and the original work is properly cited (including links to both the formal publication through the relevant DOI and the license). See: https://creativecommons.org/licenses/by-nc-nd/4.0/.
References
- van der Beek JN, Hol JA, Coulomb-l'Hermine A, et al. Characteristics and outcome of pediatric renal cell carcinoma patients registered in the International Society of Pediatric Oncology (SIOP) 93-01, 2001 and UK-IMPORT database: A report of the SIOP-Renal Tumor Study Group. Int J Cancer 2021;148:2724-35. [Crossref] [PubMed]
- Sukov WR, Hodge JC, Lohse CM, et al. TFE3 rearrangements in adult renal cell carcinoma: clinical and pathologic features with outcome in a large series of consecutively treated patients. Am J Surg Pathol 2012;36:663-70. [Crossref] [PubMed]
- Gandhi JS, Malik F, Amin MB, et al. MiT family translocation renal cell carcinomas: A 15th anniversary update. Histol Histopathol 2020;35:125-36. [PubMed]
- Akhavein A, Han J, Carter C, et al. Xp11 Translocation Renal Cell Carcinoma: Unusual Variant Masquerading as Upper Tract Urothelial Cell Carcinoma. Urol Case Rep 2014;2:75-7. [Crossref] [PubMed]
- Argani P, Laé M, Ballard ET, et al. Translocation carcinomas of the kidney after chemotherapy in childhood. J Clin Oncol 2006;24:1529-34. [Crossref] [PubMed]
- Hayes M, Peckova K, Martinek P, et al. Molecular-genetic analysis is essential for accurate classification of renal carcinoma resembling Xp11.2 translocation carcinoma. Virchows Arch 2015;466:313-22. [Crossref] [PubMed]
- Argani P. Translocation carcinomas of the kidney. Genes Chromosomes Cancer 2022;61:219-27. [Crossref] [PubMed]
- Moch H, Cubilla AL, Humphrey PA, et al. The 2016 WHO Classification of Tumours of the Urinary System and Male Genital Organs-Part A: Renal, Penile, and Testicular Tumours. Eur Urol 2016;70:93-105. [Crossref] [PubMed]
- Moch H, Amin MB, Berney DM, et al. The 2022 World Health Organization Classification of Tumours of the Urinary System and Male Genital Organs-Part A: Renal, Penile, and Testicular Tumours. Eur Urol 2022;82:458-68. [Crossref] [PubMed]
- Argani P. MiT family translocation renal cell carcinoma. Semin Diagn Pathol 2015;32:103-13. [Crossref] [PubMed]
- Malouf GG, Monzon FA, Couturier J, et al. Genomic heterogeneity of translocation renal cell carcinoma. Clin Cancer Res 2013;19:4673-84. [Crossref] [PubMed]
- Foster RE, Abdulrahman M, Morris MR, et al. Characterization of a 3;6 translocation associated with renal cell carcinoma. Genes Chromosomes Cancer 2007;46:311-7. [Crossref] [PubMed]
- Argani P, Olgac S, Tickoo SK, et al. Xp11 translocation renal cell carcinoma in adults: expanded clinical, pathologic, and genetic spectrum. Am J Surg Pathol 2007;31:1149-60. [Crossref] [PubMed]
- Argani P, Antonescu CR, Illei PB, et al. Primary renal neoplasms with the ASPL-TFE3 gene fusion of alveolar soft part sarcoma: a distinctive tumor entity previously included among renal cell carcinomas of children and adolescents. Am J Pathol 2001;159:179-92. [Crossref] [PubMed]
- Argani P, Antonescu CR, Couturier J, et al. PRCC-TFE3 renal carcinomas: morphologic, immunohistochemical, ultrastructural, and molecular analysis of an entity associated with the t(X;1)(p11.2;q21). Am J Surg Pathol 2002;26:1553-66. [Crossref] [PubMed]
- Argani P, Hicks J, De Marzo AM, et al. Xp11 translocation renal cell carcinoma (RCC): extended immunohistochemical profile emphasizing novel RCC markers. Am J Surg Pathol 2010;34:1295-303. [Crossref] [PubMed]
- Martignoni G, Pea M, Gobbo S, et al. Cathepsin-K immunoreactivity distinguishes MiTF/TFE family renal translocation carcinomas from other renal carcinomas. Mod Pathol 2009;22:1016-22. [Crossref] [PubMed]
- Green WM, Yonescu R, Morsberger L, et al. Utilization of a TFE3 break-apart FISH assay in a renal tumor consultation service. Am J Surg Pathol 2013;37:1150-63. [Crossref] [PubMed]
- Tretiakova MS, Wang W, Wu Y, et al. Gene fusion analysis in renal cell carcinoma by FusionPlex RNA-sequencing and correlations of molecular findings with clinicopathological features. Genes Chromosomes Cancer 2020;59:40-9. [Crossref] [PubMed]
- Marcon J, DiNatale RG, Sanchez A, et al. Comprehensive Genomic Analysis of Translocation Renal Cell Carcinoma Reveals Copy-Number Variations as Drivers of Disease Progression. Clin Cancer Res 2020;26:3629-40. [Crossref] [PubMed]
- Wang XT, Xia QY, Ye SB, et al. RNA sequencing of Xp11 translocation-associated cancers reveals novel gene fusions and distinctive clinicopathologic correlations. Mod Pathol 2018;31:1346-60. [Crossref] [PubMed]
- Reuter JA, Spacek DV, Snyder MP. High-throughput sequencing technologies. Mol Cell 2015;58:586-97. [Crossref] [PubMed]
- Chan TA, Yarchoan M, Jaffee E, et al. Development of tumor mutation burden as an immunotherapy biomarker: utility for the oncology clinic. Ann Oncol 2019;30:44-56. [Crossref] [PubMed]
- Goodman AM, Kato S, Bazhenova L, et al. Tumor Mutational Burden as an Independent Predictor of Response to Immunotherapy in Diverse Cancers. Mol Cancer Ther 2017;16:2598-608. [Crossref] [PubMed]
- Bakouny Z, Sadagopan A, Ravi P, et al. Integrative clinical and molecular characterization of translocation renal cell carcinoma. Cell Rep 2022;38:110190. [Crossref] [PubMed]
- Motzer RJ, Banchereau R, Hamidi H, et al. Molecular Subsets in Renal Cancer Determine Outcome to Checkpoint and Angiogenesis Blockade. Cancer Cell 2020;38:803-817.e4. [Crossref] [PubMed]
- Qu Y, Wu X, Anwaier A, et al. Proteogenomic characterization of MiT family translocation renal cell carcinoma. Nat Commun 2022;13:7494. [Crossref] [PubMed]
- Hsieh JJ, Purdue MP, Signoretti S, et al. Renal cell carcinoma. Nat Rev Dis Primers 2017;3:17009. [Crossref] [PubMed]
- Campbell SC, Clark PE, Chang SS, et al. Renal Mass and Localized Renal Cancer: Evaluation, Management, and Follow-Up: AUA Guideline: Part I. J Urol 2021;206:199-208. [Crossref] [PubMed]
- McKay RR, Bossé D, Choueiri TK. Evolving Systemic Treatment Landscape for Patients With Advanced Renal Cell Carcinoma. J Clin Oncol 2018; Epub ahead of print. [Crossref] [PubMed]
- Motzer RJ, Jonasch E, Agarwal N, et al. Kidney Cancer, Version 3.2022, NCCN Clinical Practice Guidelines in Oncology. J Natl Compr Canc Netw 2022;20:71-90. [Crossref] [PubMed]
- Zhang T, Gong J, Maia MC, et al. Systemic Therapy for Non-Clear Cell Renal Cell Carcinoma. Am Soc Clin Oncol Educ Book 2017;37:337-42. [Crossref] [PubMed]
- Maughan BL. Start of a New Era: Management of Non-Clear Cell Renal Cell Carcinoma in 2022. Curr Oncol Rep 2022;24:1201-8. [Crossref] [PubMed]
- Choueiri TK, Motzer RJ. Systemic Therapy for Metastatic Renal-Cell Carcinoma. N Engl J Med 2017;376:354-66. [Crossref] [PubMed]
- Malouf GG, Camparo P, Oudard S, et al. Targeted agents in metastatic Xp11 translocation/TFE3 gene fusion renal cell carcinoma (RCC): a report from the Juvenile RCC Network. Ann Oncol 2010;21:1834-8. [Crossref] [PubMed]
- Choueiri TK, Lim ZD, Hirsch MS, et al. Vascular endothelial growth factor-targeted therapy for the treatment of adult metastatic Xp11.2 translocation renal cell carcinoma. Cancer 2010;116:5219-25. [Crossref] [PubMed]
- Boilève A, Carlo MI, Barthélémy P, et al. Immune checkpoint inhibitors in MITF family translocation renal cell carcinomas and genetic correlates of exceptional responders. J Immunother Cancer 2018;6:159. [Crossref] [PubMed]
- Thouvenin J, Alhalabi O, Carlo M, et al. Efficacy of Cabozantinib in Metastatic MiT Family Translocation Renal Cell Carcinomas. Oncologist 2022;27:1041-7. [Crossref] [PubMed]
- Tannir NM, Jonasch E, Albiges L, et al. Everolimus Versus Sunitinib Prospective Evaluation in Metastatic Non-Clear Cell Renal Cell Carcinoma (ESPN): A Randomized Multicenter Phase 2 Trial. Eur Urol 2016;69:866-74. [Crossref] [PubMed]
- Kase AM, George DJ, Ramalingam S. Clear Cell Renal Cell Carcinoma: From Biology to Treatment. Cancers (Basel) 2023;15:665. [Crossref] [PubMed]
- Choueiri TK, Fay AP, Gray KP, et al. PD-L1 expression in nonclear-cell renal cell carcinoma. Ann Oncol 2014;25:2178-84. [Crossref] [PubMed]
- Tykodi SS, Gordan LN, Alter RS, et al. Safety and efficacy of nivolumab plus ipilimumab in patients with advanced non-clear cell renal cell carcinoma: results from the phase 3b/4 CheckMate 920 trial. J Immunother Cancer 2022;10:e003844. [Crossref] [PubMed]
- McGregor BA, McKay RR, Braun DA, et al. Results of a Multicenter Phase II Study of Atezolizumab and Bevacizumab for Patients With Metastatic Renal Cell Carcinoma With Variant Histology and/or Sarcomatoid Features. J Clin Oncol 2020;38:63-70. [Crossref] [PubMed]
- Lee CH, Voss MH, Carlo MI, et al. Phase II Trial of Cabozantinib Plus Nivolumab in Patients With Non-Clear-Cell Renal Cell Carcinoma and Genomic Correlates. J Clin Oncol 2022;40:2333-41. [Crossref] [PubMed]
- Albiges L, Gurney H, Atduev V, et al. Pembrolizumab plus lenvatinib as first-line therapy for advanced non-clear-cell renal cell carcinoma (KEYNOTE-B61): a single-arm, multicentre, phase 2 trial. Lancet Oncol 2023;24:881-91. [Crossref] [PubMed]
- McGregor BA, Huang J, Xie W, et al. Phase II study of cabozantinib (Cabo) with nivolumab (Nivo) and ipilimumab (Ipi) in advanced renal cell carcinoma with variant histologies (RCCvh). J Clin Oncol 2023;41:4520. [Crossref]
- Alhalabi O, Thouvenin J, Négrier S, et al. Immune Checkpoint Therapy Combinations in Adult Advanced MiT Family Translocation Renal Cell Carcinomas. Oncologist 2023;28:433-9. [Crossref] [PubMed]
- Ged Y. Insights Into Variant Pathology: Translocation RCC. Presented at: 2023 International Kidney Cancer Symposium; November 9-11, 2023; Nashville, TN. 2023.
- Rini BI, Powles T, Atkins MB, et al. Atezolizumab plus bevacizumab versus sunitinib in patients with previously untreated metastatic renal cell carcinoma (IMmotion151): a multicentre, open-label, phase 3, randomised controlled trial. Lancet 2019;393:2404-15. [Crossref] [PubMed]
- Choueiri TK, Powles T, Albiges L, et al. Cabozantinib plus Nivolumab and Ipilimumab in Renal-Cell Carcinoma. N Engl J Med 2023;388:1767-78. [Crossref] [PubMed]
- Griggs RC, Batshaw M, Dunkle M, et al. Clinical research for rare disease: opportunities, challenges, and solutions. Mol Genet Metab 2009;96:20-6. [Crossref] [PubMed]
- Ged Y, Voss MH. Novel emerging biomarkers to immunotherapy in kidney cancer. Ther Adv Med Oncol 2021;13:17588359211059367. [Crossref] [PubMed]
- Hsu J, Chong C, Serrill J, et al. Preclinical Characterization of XL092, a Novel Receptor Tyrosine Kinase Inhibitor of MET, VEGFR2, AXL, and MER. Mol Cancer Ther 2023;22:179-91. [Crossref] [PubMed]
- Chen YW, Rini BI, Beckermann KE. Emerging Targets in Clear Cell Renal Cell Carcinoma. Cancers (Basel) 2022;14:4843. [Crossref] [PubMed]
- Maric G, Annis MG, Dong Z, et al. GPNMB cooperates with neuropilin-1 to promote mammary tumor growth and engages integrin α5β1 for efficient breast cancer metastasis. Oncogene 2015;34:5494-504. [Crossref] [PubMed]
- Weterman MA, Ajubi N, van Dinter IM, et al. nmb, a novel gene, is expressed in low-metastatic human melanoma cell lines and xenografts. Int J Cancer 1995;60:73-81. [Crossref] [PubMed]
- Wangsiricharoen S, Ingram DR, Morey RR, et al. Glycoprotein Nonmetastatic Melanoma Protein B (GPNMB) Immunohistochemistry Can Be a Useful Ancillary Tool to Identify Perivascular Epithelioid Cell Tumor. Mod Pathol 2024;37:100426. [Crossref] [PubMed]
- Salles DC, Asrani K, Woo J, et al. GPNMB expression identifies TSC1/2/mTOR-associated and MiT family translocation-driven renal neoplasms. J Pathol 2022;257:158-71. [Crossref] [PubMed]
- Lang M, Schmidt LS, Wilson KM, et al. High-throughput and targeted drug screens identify pharmacological candidates against MiT-translocation renal cell carcinoma. J Exp Clin Cancer Res 2023;42:99. [Crossref] [PubMed]
- Ghosh A, Heston WD. Tumor target prostate specific membrane antigen (PSMA) and its regulation in prostate cancer. J Cell Biochem 2004;91:528-39. [Crossref] [PubMed]
- Spatz S, Tolkach Y, Jung K, et al. Comprehensive Evaluation of Prostate Specific Membrane Antigen Expression in the Vasculature of Renal Tumors: Implications for Imaging Studies and Prognostic Role. J Urol 2018;199:370-7. [Crossref] [PubMed]
- Baraban EG, Ged Y, Singla N, et al. Vascular Expression of Prostate-specific Membrane Antigen (PSMA) in MiTF Family Translocation Renal Cell Carcinoma and Related Neoplasms. Appl Immunohistochem Mol Morphol 2023;31:544-9. [Crossref] [PubMed]