Low-dose apatinib optimizes the vascular normalization and enhances the antitumor effect of PD-1 inhibitor in gastric cancer
Highlight box
Key findings
• Low-dose apatinib has been shown to enhance the antitumor effects of programmed cell death protein 1 (PD-1) inhibitor in gastric cancer (GC) by normalizing tumor-associated blood vessels, alleviating intratumor hypoxia, and altering the immunosuppressive microenvironment (IM).
What is known and what is new?
• As the fifth most commonly diagnosed cancer, GC has also been recognized as the third leading cause of cancer-related deaths worldwide. Immune checkpoint inhibitors (ICIs) have been used in patients with GC, which are regarded as emerging cancer drugs with great clinical potential.
• Low-dose apatinib (50 mg/kg) optimizes vascular normalization and enhances the antitumor effect of PD-1 inhibitor in GC.
What is the implication, and what should change now?
• Treatment with low-dose apatinib hinders the IM characterized by regulatory T cells, myeloid-derived suppressor cells and Tyro3, Axl and MerTK, which recruit more effector T cells to promote the therapeutic effect of immune checkpoint PD-1 inhibitor in GC.
Introduction
As the fifth most commonly diagnosed cancer, gastric cancer (GC) has also been recognized as the third leading cause of cancer-related deaths worldwide (1-3). In China, there is the similar situation that GC is commonly diagnosed, being one of the most common causes of death (4). Due to the lack of a comprehensive nationwide screening program, a large proportion of GC patients in China are diagnosed at an advanced stage (4). There is an extremely low 5-year survival rate of GC patients with stage III tumors, ranging from 18% to 50% (5). Currently, there are limited treatment options for locally advanced and metastatic GC patients, especially for patients resistant to first- or second-line chemotherapy. There are rarely targeted therapies that can provide substantial benefit to patient survival, with limited options such as trastuzumab and ramucirumab, and the survival after chemotherapy resistance is very disappointing (6,7).
Recently, it has been reported that immune checkpoint inhibitors (ICIs) have been used in GC patients, which are regarded as emerging cancer drugs with great clinical potential. Immunotherapies targeting immune checkpoint molecules such as programmed cell death protein 1 ligand 1 (PD-L1) and programmed cell death protein 1 (PD-1) have been proven to be effective in the treatment of multiple malignant tumors, such as melanoma, non-small cell lung cancer and colorectal cancer, as well as the tumors with high levels of microsatellite instability (MSI-H) and DNA deficient mismatch repair (dMMR) (8-10). The PD-1-targeting inhibitor has been approved for use as a third-line treatment for advanced GC with high expression levels of PD-L1 (11). However, only about 11–20% of patients with unselected tumors exhibited a high response to this treatment. At the same time, another PD-1-targeting inhibitor, pembrolizumab, also failed to improve its survival rate in a phase III clinical trial targeting PD-L1-overexpressed advanced GC (12). Consequently, it is urgent to explore new strategies to improve the therapeutic effect of ICI in GC patients.
Apatinib is a tyrosine kinase inhibitor targeting vascular endothelial growth factor receptor 2 (VEGFR-2), and has been approved for the treatment of advanced GC in China (13). Besides, it has now been widely used clinically as an oral drug, showing potential when being combinedly used with ICI in patients with advanced GC. Apatinib exhibited highly selective competitive ability for the adenosine-triphosphate (ATP) binding site of VEGFR-2 in cells to block downstream signal transduction and inhibit neovascularization in tumor tissues (14). Studies have shown that the rational application of angiogenesis inhibitors can effectively promote the normalization of blood vessels in tumors, thereby improving the abnormal vascular perfusion, hypoxia, and low pondus hydrogenii (pH) in tumors, increasing the infiltration of immunocyte in tumors, and enhancing the anti-tumor activity of immunotherapy drugs (15-17).
Although the combined use of PD-1 inhibitor with apatinib has shown improved therapeutic outcomes in GC, the mechanism of action has not been fully elucidated. Consequently, the purpose of this research is to explore the impact of apatinib on the anti-tumor effect of PD-1 inhibitor in GC and clarify the underlying mechanisms by constructing a mouse model of GC to compare the effects of different doses of apatinib as well as the combined use of apatinib with PD-1 inhibitor on the experimental mice with GC. We present this article in accordance with the ARRIVE reporting checklist (available at https://tcr.amegroups.com/article/view/10.21037/tcr-23-2328/rc).
Methods
Tumor cell lines and experimental mice
The mouse forestomach carcinoma cell (MFC) was purchased from the Cell Bank of the Chinese Academy of Sciences and cultured in Dulbecco’s Modified Eagle Medium (DMEM) medium containing 10% serum and 1% penicillin-streptomycin. Thirty 6-week-old female 615 mice (30–35 g) were purchased from the Chinese Academy of Medical Sciences and raised in a specific pathogen free (SPF) environment with a temperature of 24±2 ℃ and a humidity of 55%±5%, alternating light and dark cycle, and free access to food and drink. The mice underwent adaptive feeding for a week. A protocol was prepared before the study without registration. All animal experiments involved in this study were approved by the Institutional Animal Care and Use Committee of Zhejiang Center of Laboratory Animals (Ethics batch No. ZJCLA-IACUC-20020079), in compliance with institutional guidelines for the care and use of animals.
GC mouse model establishment
Appropriate point for needle injection was chosen in the right armpit of the mice subcutaneously. The whole procedures of tumor model are listed as follow: (I) injecting 1×107 MFC cells resuspended in 1 mL phosphate buffer solution (PBS) to the mice to form tumor subcutaneously; (II) dividing all mice into 5 groups randomly (as shown in Table 1, n=6) after 6 days; (III) treating the mice in each group for 14 days and all injection were performed intraperitoneally; (IV) monitoring and recording tumor size and bodyweight of mice every 2 days; (V) after 20 days, the blood of the mice was collected, the mice were euthanized with 3 times the normal dose of anesthesia, and the tumors were collected (anti-PD1, Merck Co., Ltd., Darmstadt, Germany; apatinib, Hengrui Medicine Co., Ltd., Lianyungang, China).
Table 1
Group name | Treatment |
---|---|
NS group | 200 mL normal saline daily |
A group | Low-dose apatinib group: apatinib (dissolved in 0.9% sodium chloride solution) at a dose of 50 mg/kg daily |
B group | High-dose apatinib group: apatinib (dissolved in 0.9% sodium chloride solution) at a dose of 200 mg/kg daily |
C group | PD-1 inhibitor monotherapy group: PD-1 inhibitor (dissolved in 0.9% sodium chloride solution) at a dose of 2 mg/kg every 3 days |
D group | PD-1 inhibitor combined with the low-dose apatinib group: apatinib at a dose of 50 mg/kg daily for 14 days, PD-1 inhibitor at a dose of 2 mg/kg every 3 days |
NS, normal saline; PD-1, programmed cell death protein 1.
Histologic analysis
Collect mouse tumor tissue and make paraffin sections. Hematoxylin and eosin (HE) staining or immunofluorescent staining were then performed. Vascular formation analysis was conducted using CD31/alpha-smooth muscle actin (α-SMA) and CD31/collagen IV (CD31, ab28364; Col IV, ab19808; α-SMA, ab7817; Abcam, Cambridge, UK). The cell nuclei were stained by 4’,6-diamidino-2-phenylindole (DAPI). Also, the immunohistochemistry staining of the tumor tissue sections was executed according to the producer’s procedures for hypoxia inducible factor 1 alpha (HIF1α) (ab179483, Abcam) and CD8 (ab14-0808-82, eBioscience, California, USA). Confocal laser-scanning microscopy was photographed on a laser scanning confocal microscope (Nikon A1, Nikon, Tokyo, Japan).
Flow cytometry
The collected tumor tissues were enzymatically digested at room temperature for 45 minutes to obtain single cell suspensions. Erythrocyte lysis (Sigma, Missouri, USA) was used to lyse erythrocytes. The single-cell suspensions were blocked with CD16/32 (Abcam, ab223200), CD8 (Abcam, ab223200), CD11b (Abcam, ab217344), F4/80 (Abcam, ab6640), or negative controls. All the data were analyzed using FlowJo software.
Cytokine assay
After euthanasia of the mice, all the tumor tissues and blood samples were collected and transferred to a refrigerator at −80 ℃ for further cytokine analysis. The evaluation of cytokine was performed based on enzyme-linked immuno sorbent assay (ELISA) analysis. The quantitative measurement of intratumoral tumor necrosis factor α (TNFα) was detected using mouse TNFα ELISA kit (Abcam, ab208348). All the samples were tested in duplicate.
Statistical analysis
GraphPad PRISM 6.0 was used for the entire image processing. The statistical program IBM SPSS 25.0 was used for comparative analysis. The one-way analysis of variance (ANOVA) and t-test analysis were performed in order to make comparisons among different treatment groups, and P<0.05 indicated statistically remarkable difference.
Results
Low-dose apatinib suppressed tumor growth in mice with GC
The bearing GC model was successfully constructed (Figure 1A). No statistical difference was revealed in the body weight of all the mice in each group, and the tumor inhibition rate of mice in group A was 23.34%±0.09% (Table 2). The tumor tissues from subcutaneous xenograft models can be seen in Figure 1B. Results demonstrated that mice in group A treated with low-dose apatinib showed smaller tumor volumes and grew slowly compared with those in the control group (Figure 1B,1C).
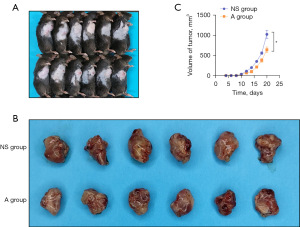
Table 2
Group | N | Body weight (g), mean ± SD | Tumor weight (mg), mean ± SD | Tumor inhibition rate (%), mean ± SD |
---|---|---|---|---|
A | 5 | 24.08±0.33 | 906.70±24.99 | 23.34±0.09 |
NS | 5 | 23.98±1.00 | 1,203.00±86.51 | – |
Group A: low-dose apatinib 50 mg/kg; Group NS: normal saline. SD, standard deviation.
Low-dose apatinib alleviated hypoxia and promoted intratumor vascular normalization
Anti-VEGF/R2 treatment has been shown to modulate abnormal tumor vasculature, thereby alleviating intratumor hypoxia (18). Herein, we focused our attention on analysis of vascular normalization and the hypoxic microenvironment in tumors. Perivascular cell coverage was sensibly higher in the low-dose apatinib group compared with the control and the high-dose apatinib groups, as revealed by CD31/α-SMA dual staining results (Figure 2A,2B), whereas the tumor microvascular density (MVD) on the basis of CD31+ vessel numbers per field showed no difference (Figure 2C). Afterwards, we observed increased collagen IV as basement membrane (BM) in the low-dose apatinib group (Figure 2D). Moreover, low-dose apatinib was found to alleviate hypoxia. In addition, the expression of HIF1α in the low-dose apatinib group were shown to be significantly decreased when compared with that in the high-dose apatinib group (Figure 2E,2F).
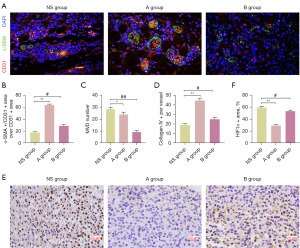
Low-dose apatinib hindered the immunosuppressive microenvironment (IM)
Intratumoral vasculature has been revealed by previous studies to have strong associations with the filtration of immune-cells in tumors (15,19). Thence, we appraised the effects of low-dose apatinib on the immunosuppressive activity of anti-tumor immunity response related T-cells in tumor microenvironment (TME). Results displayed that the fraction of tumor-associated macrophages (TAMs) obviously decreased after treatment with low-dose apatinib (Figure 3A,3B). Besides, we also examined CD163 cells in tumors and found a significantly decreased proportion of M2-like macrophage (CD163) in the low-dose apatinib group (Figure 3C). In order to further assess the CD8+ cells in tumors, immunohistochemistry staining was carried out. The fractions of CD8+ cells were revealed to be the highest in the low-dose apatinib group (Figure 3D). All these results display that the normalization of the vascular system in tumors may affect the decrease of immunosuppressive cells after treatment with low-dose apatinib.
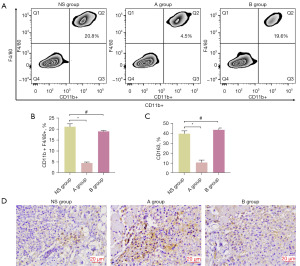
PD-1 inhibitor combined with low-dose apatinib led to optimized antitumor effects
The presence of an immunosuppressive environment and abnormal angiogenesis are well-recognized barriers to effective cancer immunotherapy (20). Based on the previous results, we hypothesized that the combined use of low-dose apatinib and PD-1 inhibitor may have significant antitumor effect. As expected, the combined use of low-dose apatibib plus PD-1 inhibitor decreased the tumor size and tumor weight compared with other groups. Although the monotherapy of apatinib or PD-1 inhibitor also showed obvious antitumor effect, their combined use exhibited the strongest effect in decreasing tumor growth (Figure 4A,4B). HE staining results showed a higher proportion of necrotic tumor tissues in the low-dose apatinib plus PD-1 inhibitor group than that in other groups (Figure 4C). Besides, the expression of NG2 and HIF1α in tumors were obviously decreased when using the combined treatment of low-dose apatinib and PD-1 inhibitor, indicating a more normalized vascular density and alleviated hypoxia in TME (Figure 4C).
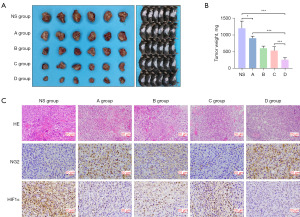
Expression of immune cells and molecules after the combined use of PD-1 inhibitor and low-dose apatinib
To find out whether low-dose apatinib plus PD-1 inhibitor could alleviate immunosuppressive TME to further enhance antitumor effect, the intratumoral immune components were accessed. Consistent with the results from the monotherapy of apatinib, the combined use of low-dose apatinib and PD-1 inhibitor brought about a better immunosupportive tumor environment. More CD8+ T cells (Figure 5A) but fewer TAMs (Figure 5B) were observed in the tumor environment. PD-1 inhibitor monotherapy appeared to increase the proportion of CD8+ T cells, and this effect was further enhanced with the combined use of PD-1 inhibitor with apatinib. However, no statistical difference was found in the levels of TNFα and interferon-γ (IFN-γ) after treatment using PD-1 inhibitor with or without apatinib (Figure 5C,5D), indicating that the obvious antitumor effects by the use of this combined treatment may be attributed to the transformation of immune components in TME.
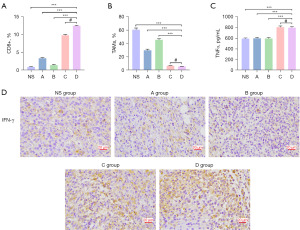
Discussion
GC is one of the malignant tumors seriously endangering human health, and most GC patients in China are only discovered in the late stage (4). Although combined chemotherapy remains the standard treatment for advanced GC, its efficacy has not been significantly improved for several years. At present, ICI targeting PD-1 have been approved for advanced GC treatment, offering new immunotherapy options for GC patients (11). However, compared with the efficacy of PD-1 inhibitor in lung cancer, ICI have unsatisfactory efficacy in GC, and have not yet been approved for first- and second-line treatment of GC (12,21).
In recent years, researches have reported that the development of GC is accompanied by the formation of a large number of new blood vessels (22-24). Different from normal blood vessels, intratumoral neovascularization exhibits structural and functional abnormalities, forming a tumor IM characterized by interstitial hypertension, hypoxia, and low pH, thus affecting the efficacy of immunotherapy. Abnormally generated angiogenesis in tumors can promote the generation of an IM, which enables tumor cells to evade host immune surveillance, and inhibit the antitumor activity of immunotherapeutic drugs (19,25). Compared with normal tissues, intratumoral blood vessels are abnormal in morphology and function, resulting in a hypoxic microenvironment, low pH, and high tissue hydraulic pressure within the tumor (26). Tumor cells adapt to such an environment rapidly and thus achieve a higher survival advantage over immune cells. In addition, abnormal angiogenesis in tumors also inhibits the filtration of circulating immune cells into the tumor, thereby limiting the antitumor activity of immune cells (27). Finally, a variety of cells in the tumor produce a variety of immunosuppressive cytokines, such as C-C motif ligand 2 (CCL2), angiopoietin-2 (ANG2), C-C motif chemokine ligand 28 (CCL28), C-X-C motif ligand 8 (CXCL8), C-X-C chemokine ligand 12 (CXCL12), vascular endothelial-derived growth factor (VEGF), etc., in the environment with little oxygen and low pH. These cytokines are released into the blood circulation to recruit a variety of immunosuppressive cells into the tumor, including Tregs, TAM and myeloid-derived suppressor cells (MDSCs), etc. (28,29). Therefore, promoting the intratumoral vascular normalization is essential to improve the therapeutic effects of ICI in malignant tumors by improving the intratumoral IM.
Immunotherapy based on anti-PD-1 treatment exhibited outstanding clinical benefits in certain end-stage GC patients, but many patients do not benefit fromanti-PD-1 single-agent therapy. Resistance to PD-1 inhibitor resistance may occur at various stages of the antitumor immune response, such as reduced production of new immunogenic antigens, disabled antigen presenting cells (APCs), failed tumor-related antigen process, deficiencies in initiating T cells specific immune response, and the formation of immunosuppressive TME (30). Based on these mechanisms, strategies aimed at enhancing specific aspects of the antitumor immune response through binding interactions may help alleviate anti-PD-1 resistance. The VEGF signaling pathway has demonstrated potential to promote angiogenesis and mediate tumor-related immune response by inducing amplification of immunosuppressive cell subgroups while influencing lymphocyte trafficking (31). Preclinical studies have reported that anti-VEGF/VEGFR-2 alters the invasion of a series of tumor-type lymphocytes or macrophages, and its combined use with immunotherapy enhances the antitumor effect (32-34). Despite the above-mentioned findings, there is currently a lack of data on the combined treatment of GC with both antiangiogenesis and anti-PD-1 therapy. Therefore, the present study aims to clarify whether low-dose apatinib could exert functions on changing the immunosuppressive environment and strengthen the antitumor efficacy of PD-1 inhibitor in a GC animal model.
As shown in this study, compared to other intervention groups, there was a significantly less subcutaneous xenograft tumor accumulation in the group of mice subjected to the combined therapy of PD-1 inhibitor and apatinib, with the PD-1 inhibitor showing an obviously enhanced efficacy. This effect may be attributed to the outstanding ability of normalizing intratumor vessels. The vascular normalization reflected in the increased perivascular cell coverage and decreased intratumor MVD resulting in the alleviation of intratumor hypoxia and less delivery of oxygen and nutrients to tumor via blood vessels. Besides, apatinib also demonstrated the potential in changing the IM. After treatment with low-dose apatinib, the proportion of TAM, M2-like macrophages, MDSC, which were recognized as immunosuppressive cells, reduced, while the proportion of CD8+ T cells, which are involved in the specific antitumor immune response of T cells, raised. The vascular normalization and changes in the IM by apatinib significantly enhanced the efficacy of the PD-1 inhibitor. Mice receiving the combined therapy of PD-1 inhibitor and low-dose apatinib exhibited the smallest tumor size. Furthermore, this combined therapy raised the proportion of CD8+ T cells compared to either the monotherapy of apatinib or the PD-1 inhibitor.
Although this research has identified that low-dose apatinib strengthens the anti-tumor effect of PD-1 inhibitor in GC by normalizing tumor-related blood vessels, alleviating intratumor hypoxia and changing the IM, certain research limitations still exist. Firstly, although there was no change in the tumor size in both the low- and high-dose apatinib groups, a higher perivascular cell coverage was observed in the low-dose apatinib group, indicating vascular normalization and alleviated hypoxia in TME. Due to ethical considerations regarding animal ethics, we did not set the group with treatment of PD-1 inhibitor plus high-dose apatinib, as high doses of apatinib may pose more safety concerns and have less impact on changing hypoxia in tumor. Therefore, further investigation into the combined use of PD-1 inhibitor with high-dose apatinib still remains of interest. Secondly, this research is limited by the absence of clinical samples. The current research is confined to animal and cell levels, and the obtained research data may not fully represent practical clinical scenarios. Therefore, future research focusing on this topic should incorporate clinical samples to enhance the credibility of the current research results and promote the clinical transformation of the combination therapy.
Conclusions
Low-dose apatinib exhibited great potential in reducing GC size by normalizing tumor-related vessels, alleviating intratumor hypoxia and transforming the IM. Hence, low-dose apatinib can enhance the efficacy of PD-1 inhibitor. The results indicate that the combined use of PD-1 inhibitor and low-dose apatinib is highly effective in treating mice with GC. This combination therapy could benefit more patients with GC.
Acknowledgments
Funding: This work was supported by grants from
Footnote
Reporting Checklist: The authors have completed the ARRIVE reporting checklist. Available at https://tcr.amegroups.com/article/view/10.21037/tcr-23-2328/rc
Data Sharing Statement: Available at https://tcr.amegroups.com/article/view/10.21037/tcr-23-2328/dss
Peer Review File: Available at https://tcr.amegroups.com/article/view/10.21037/tcr-23-2328/prf
Conflicts of Interest: All authors have completed the ICMJE uniform disclosure form (available at https://tcr.amegroups.com/article/view/10.21037/tcr-23-2328/coif). The authors have no conflicts of interest to declare.
Ethical Statement: The authors are accountable for all aspects of the work in ensuring that questions related to the accuracy or integrity of any part of the work are appropriately investigated and resolved. Approval was granted by the Institutional Animal Care and Use Committee of Zhejiang Center of Laboratory Animals (No. ZJCLA-IACUC-20020079), in compliance with institutional guidelines for the care and use of animals.
Open Access Statement: This is an Open Access article distributed in accordance with the Creative Commons Attribution-NonCommercial-NoDerivs 4.0 International License (CC BY-NC-ND 4.0), which permits the non-commercial replication and distribution of the article with the strict proviso that no changes or edits are made and the original work is properly cited (including links to both the formal publication through the relevant DOI and the license). See: https://creativecommons.org/licenses/by-nc-nd/4.0/.
References
- Siegel RL, Miller KD, Fuchs HE, et al. Cancer Statistics, 2021. CA Cancer J Clin 2021;71:7-33. [Crossref] [PubMed]
- Guan WL, He Y, Xu RH. Gastric cancer treatment: recent progress and future perspectives. J Hematol Oncol 2023;16:57. [Crossref] [PubMed]
- Bray F, Ferlay J, Soerjomataram I, et al. Global cancer statistics 2018: GLOBOCAN estimates of incidence and mortality worldwide for 36 cancers in 185 countries. CA Cancer J Clin 2018;68:394-424. [Crossref] [PubMed]
- Gao K, Wu J. National trend of gastric cancer mortality in China (2003-2015): a population-based study. Cancer Commun (Lond) 2019;39:24. [Crossref] [PubMed]
- Sexton RE, Al Hallak MN, Diab M, et al. Gastric cancer: a comprehensive review of current and future treatment strategies. Cancer Metastasis Rev 2020;39:1179-203. [Crossref] [PubMed]
- Oh DY, Bang YJ. HER2-targeted therapies - a role beyond breast cancer. Nat Rev Clin Oncol 2020;17:33-48. [Crossref] [PubMed]
- Takei S, Kawazoe A, Shitara K. The New Era of Immunotherapy in Gastric Cancer. Cancers (Basel) 2022;14:1054. [Crossref] [PubMed]
- Shiravand Y, Khodadadi F, Kashani SMA, et al. Immune Checkpoint Inhibitors in Cancer Therapy. Curr Oncol 2022;29:3044-60. [Crossref] [PubMed]
- Li Q, Han J, Yang Y, et al. PD-1/PD-L1 checkpoint inhibitors in advanced hepatocellular carcinoma immunotherapy. Front Immunol 2022;13:1070961. [Crossref] [PubMed]
- Shi T, Zhang Y, Wang Y, et al. DKK1 Promotes Tumor Immune Evasion and Impedes Anti-PD-1 Treatment by Inducing Immunosuppressive Macrophages in Gastric Cancer. Cancer Immunol Res 2022;10:1506-24. [Crossref] [PubMed]
- Zhang Z, Cheng S, Qi C, et al. Response to the rechallenge of combination immunotherapy in a patient with late-stage gastric cancer: case report. Ann Palliat Med 2022;11:818-26. [Crossref] [PubMed]
- Shitara K, Özgüroğlu M, Bang YJ, et al. Pembrolizumab versus paclitaxel for previously treated, advanced gastric or gastro-oesophageal junction cancer (KEYNOTE-061): a randomised, open-label, controlled, phase 3 trial. Lancet 2018;392:123-33. [Crossref] [PubMed]
- Hironaka S. Anti-angiogenic therapies for gastric cancer. Asia Pac J Clin Oncol 2019;15:208-17. [Crossref] [PubMed]
- Gong T, Luo Y, Wang Y, et al. Multiple Pulmonary Metastases of Recurrent Giant Cell Tumor of Bone with Expression of VEGFR-2 Successfully Controlled by Denosumab and Apatinib: A Case Report and Literature Review. Cancer Manag Res 2021;13:4447-54. [Crossref] [PubMed]
- Shi Y, Li Y, Wu B, et al. Normalization of tumor vasculature: A potential strategy to increase the efficiency of immune checkpoint blockades in cancers. Int Immunopharmacol 2022;110:108968. [Crossref] [PubMed]
- Takakura N. Immune therapy and tumor angiogenesis. Rinsho Ketsueki 2020;61:1440-5. [PubMed]
- Dudley AC, Griffioen AW. Pathological angiogenesis: mechanisms and therapeutic strategies. Angiogenesis 2023;26:313-47. [Crossref] [PubMed]
- Roy T, James BD, Allen JB. Anti-VEGF-R2 Aptamer and RGD Peptide Synergize in a Bifunctional Hydrogel for Enhanced Angiogenic Potential. Macromol Biosci 2021;21:e2000337. [Crossref] [PubMed]
- Sun Y, Chen W, Torphy RJ, et al. Blockade of the CD93 pathway normalizes tumor vasculature to facilitate drug delivery and immunotherapy. Sci Transl Med 2021;13:eabc8922. [Crossref] [PubMed]
- Chen C, Wang Z, Ding Y, et al. Tumor microenvironment-mediated immune evasion in hepatocellular carcinoma. Front Immunol 2023;14:1133308. [Crossref] [PubMed]
- Zhao S, Ren S, Jiang T, et al. Low-Dose Apatinib Optimizes Tumor Microenvironment and Potentiates Antitumor Effect of PD-1/PD-L1 Blockade in Lung Cancer. Cancer Immunol Res 2019;7:630-43. [Crossref] [PubMed]
- Joshi SS, Badgwell BD. Current treatment and recent progress in gastric cancer. CA Cancer J Clin 2021;71:264-79. [Crossref] [PubMed]
- Biagioni A, Peri S, Versienti G, et al. Gastric Cancer Vascularization and the Contribution of Reactive Oxygen Species. Biomolecules 2023;13:886. [Crossref] [PubMed]
- Huang HW, Chen CY, Huang YH, et al. CMAHP promotes metastasis by reducing ubiquitination of Snail and inducing angiogenesis via GM-CSF overexpression in gastric cancer. Oncogene 2022;41:159-72. [Crossref] [PubMed]
- Zhang L, Xu J, Zhou S, et al. Endothelial DGKG promotes tumor angiogenesis and immune evasion in hepatocellular carcinoma. J Hepatol 2024;80:82-98. [Crossref] [PubMed]
- Li S, Zhang Q, Hong Y. Tumor Vessel Normalization: A Window to Enhancing Cancer Immunotherapy. Technol Cancer Res Treat 2020;19:1533033820980116. [Crossref] [PubMed]
- Pan Y, Yu Y, Wang X, et al. Tumor-Associated Macrophages in Tumor Immunity. Front Immunol 2020;11:583084. Erratum in: Front Immunol 2021;12:775758. [Crossref] [PubMed]
- Tsuchiya H, Shiota G. Immune evasion by cancer stem cells. Regen Ther 2021;17:20-33. [Crossref] [PubMed]
- Rahma OE, Hodi FS. The Intersection between Tumor Angiogenesis and Immune Suppression. Clin Cancer Res 2019;25:5449-57. [Crossref] [PubMed]
- Lahiri A, Maji A, Potdar PD, et al. Lung cancer immunotherapy: progress, pitfalls, and promises. Mol Cancer 2023;22:40. [Crossref] [PubMed]
- Patel SA, Nilsson MB, Le X, et al. Molecular Mechanisms and Future Implications of VEGF/VEGFR in Cancer Therapy. Clin Cancer Res 2023;29:30-9. [Crossref] [PubMed]
- Tamura R, Fujioka M, Morimoto Y, et al. A VEGF receptor vaccine demonstrates preliminary efficacy in neurofibromatosis type 2. Nat Commun 2019;10:5758. Erratum in: Nat Commun 2020;11:2028. [Crossref] [PubMed]
- Tamura R, Morimoto Y, Kosugi K, et al. Clinical and histopathological analyses of VEGF receptors peptide vaccine in patients with primary glioblastoma - a case series. BMC Cancer 2020;20:196. Erratum in: BMC Cancer 2020;20:301. [Crossref] [PubMed]
- Molgora M, Esaulova E, Vermi W, et al. TREM2 Modulation Remodels the Tumor Myeloid Landscape Enhancing Anti-PD-1 Immunotherapy. Cell 2020;182:886-900.e17. [Crossref] [PubMed]