The LINC00957/miR-17-5p axis regulates the cell cycle and migration in glioblastoma via the cuproptosis-related gene nephronectin
Highlight box
Key findings
• LINC00957/miR-17-5p/nephronectin (NPNT) axis promote the malignant progression of glioblastoma (GBM).
What is known and what is new?
• The competing endogenous RNA (ceRNA) network and cuproptosis can regulate the incidence of GBM.
• NPNT could promote cuproptosis.
• LINC00957 acted as a ceRNA to regulate NPNT via miR-17-5p.
What is the implication, and what should change now?
• Cuproptosis-related ceRNA axis regulates GBM. It is expected that animal model experiments will further verify.
Introduction
Glioblastoma (GBM), an aggressive form of brain cancer, is known for its poor prognosis and high malignancy (1). GBM arises from astrocytes, the supportive cells in the brain, and rapidly grows and invades surrounding brain tissue (2). The types of treatments typically include surgery, radiation, and chemotherapy, mostly with the drug temozolomide (3). However, despite these interventions, the survival rate of patients with GBM remains low, with an average of approximately 15 months (4). The resistance of the disease to conventional therapies is a significant challenge, largely owing to the tumor’s complex genetic mutations, its capacity for adaptation and evolution, and the challenges in administering effective treatments through the blood-brain barrier (5). Therefore, it is imperative to look for novel potential therapeutic targets.
Non-coding RNAs, such as microRNAs (miRNA) and long non-coding RNAs (lncRNA), regulate gene expression and play a crucial role in cellular functions and diseases (6). MiRNAs, which are small non-coding RNA segments, regulate gene expression after transcription, either by degrading messenger RNA (mRNA) or inhibiting its translation (7). In tumors, these segments function as oncogenes or tumor suppressors, influencing cell proliferation, apoptosis, and metastasis (8). In GBM, miRNAs regulate gene expression, affecting tumor growth, invasion, and resistance to therapy, and have been investigated as a potential target for novel therapeutic strategies and biomarkers (9). MiR-17-5p is a single miRNA that can specifically act on the G1/S phase cell cycle boundary. MiR-17-5p regulates the cell cycle, apoptosis, and invasion pathways acting as a tumor suppressor in GBM (10,11). MiR-17-5p is down-regulated in our findings and that this agrees with previous studies (10,11). Thus, miR-17-5p may be a promising therapeutic target and biomarker for GBM and other tumors.
LncRNAs, which are longer RNA molecules, primarily function by modulating gene expression at different stages, including chromatin modification, transcription, and post-transcriptional processing (12). In tumors, lncRNAs can serve as oncogenes or suppressors, influencing cancer initiation, progression, and metastasis (13). In GBM, lncRNAs contribute to tumor growth, drug resistance, and recurrence (14). They are involved in tumor progression and have potential as a target for novel therapeutic strategies (15). The expression of LINC00957 is strongly correlated with the staging and chemotherapy results of certain tumors (16). In recent years, the role of LINC00957 in the prognosis of GBM has been reported in studies, which also illustrates its potential importance (17). Competing endogenous RNAs (ceRNAs) control gene expression by capturing miRNAs, thus influencing their activity (18). Tumors, including GBM, contribute to oncogenesis and progression by disrupting normal miRNA-mediated gene regulation, offering novel insights into cancer biology and potential therapeutic targets.
Regulated cell death (RCD) is a vital biological process involving various mechanisms like apoptosis, necroptosis, and autophagy, which are crucial for maintaining cellular homeostasis and eliminating damaged or harmful cells (19). Cuproptosis is a novel type of RCD, distinct from other types owing to its unique reliance on copper-dependent pathways (20). Unlike apoptosis or necroptosis, which involve protein oligomerization or degradation, cuproptosis is driven by metabolic disruption (21). In tumors, cuproptosis presents a promising therapeutic target. Multiple cancers exhibit altered copper metabolism, and exploring this through cuproptosis could help selectively target cancer cells, especially cells resistant to conventional RCD pathways like apoptosis (22). Owing to its aggressive nature and resistance to standard therapies, GBM can particularly benefit from cuproptosis-based treatments (23). Research is being conducted to explore the sensitivity of GBM to copper-dependent cell death. Targeting cuproptosis in GBM may offer a novel approach to circumvent the typical resistance mechanisms these tumors typically exhibit, which could help develop more effective therapies against this devastating disease (24). The ongoing investigation into cuproptosis in GBM and other cancers opens new frontiers in cancer treatment strategies.
The nephronectin (NPNT) gene encodes NPNT, a component of the extracellular matrix (25). It plays a key role in cell adhesion and signaling, influencing cellular behaviors crucial for tissue development and repair (25). In tumors, NPNT has been implicated in cell cycle and cell migration regulation (26). Its expression can affect tumor growth and metastasis by modulating cell interactions and the surrounding environment (27). The effect of NPNT on the cell cycle can alter the proliferation rate of cancer cells, whereas its role in cell migration is of significance in cancer invasion and metastasis (28). However, the mechanism of action of NPNT in GBM has not been investigated thoroughly, and its relationship with cuproptosis has never been reported.
In this study, we found that in GBM, LINC00957 uses a ceRNA for miR-17-5p, thereby influencing the expression of the cuproptosis-related gene (CRG) NPNT. Based on the assessment of copper ion concentration and reactive oxygen species (ROS) levels, our initial findings indicated that NPNT inhibition enhances cuproptosis. Furthermore, findings from enrichment analysis and phenotypic experiments revealed that the LINC00957/miR-17-5p/NPNT axis significantly regulates the cell cycle and cell migration in GBM. Mechanistic insights from reporter gene experiments suggested that LINC00957 modulates NPNT expression by serving as a ceRNA for miR-17-5p. We present this article in accordance with the MDAR reporting checklist (available at https://tcr.amegroups.com/article/view/10.21037/tcr-24-450/rc).
Methods
Data acquisition
We obtained glioma data and clinical information from The Cancer Genome Atlas (TCGA) database (https://www.cancer.gov/about-nci/organization/ccg/research/structural-genomics/tcga), which included the expression levels of mRNAs, miRNAs, and lncRNAs. GSE63319 was used to retrieve miRNA expression data from normal brain and glioma tissues from the Gene Expression Omnibus (GEO) database (https://www.ncbi.nlm.nih.gov/geo/). The study was conducted in accordance with the Declaration of Helsinki (as revised in 2013).
Differentially expressed gene (DEG) analysis
We used R package limma to analyze the DEGs of normal and tumor cells in the TCGA and GEO databases. CRGs were also identified based on the different levels of cuproptosis in patients with glioma using cluster analysis. Results with log2fold change (FC) ≥1 and adjusted P<0.05 were considered meaningful.
Univariate regression analysis
Univariate Cox regression analysis was performed to evaluate the prognostic value of the mRNAs, miRNAs, and lncRNAs of 170 patients with glioma in the TCGA database.
Gene set enrichment analysis (GSEA)
R package clusterprofiler was used to analyze the GSEA results of the DEGs of lncRNAs, miRNAs, and mRNAs in patients with glioma. The P value <0.05 was significant.
Construction of the ceRNA axes
We used the obtained differentially expressed lncRNAs, miRNAs, and cuproptosis-related mRNAs to construct the ceRNA axis. First, the relationship between lncRNA and miRNA was determined using the miRcode database. Second, the potential target mRNAs were predicted based on the miRNA in TargetScan, miRDB, miRTarbase, and ENCORI databases. Lastly, we filtered the results based on the survival analysis results of lncRNAs, miRNAs, and mRNAs and constructed the final ceRNA network. The software Cytoscape (version 3.9.1) was used to visualize the regulatory relationships. A Sankey diagram was visualized using R package ggalluvial and ggplot2.
Pearson correlation analysis
We performed Pearson correlation analysis using log2[transcripts per million (TPM) +1] values of lncRNAs and mRNAs and log2[reads per million mapped reads (RPM) +1] values of miRNAs. R-squared ≥0.3 and P value <0.05 were considered relevant.
Cell culture
We cultured human glioma U87 (catalog number: HTB-14) and LN229 (catalog number: CRL-2611) cells for the study. The cells were obtained from the American Type Culture Collection (ATCC). U87 cells were cultured in Eagle’s minimum essential medium (EMEM) supplemented with 10% fetal bovine serum (FBS), 100 µg/mL penicillin, and 100 µg/mL streptomycin at 37 ℃ in 5% CO2. LN229 cells were cultured in Dulbecco’s modified Eagle’s medium (DMEM) supplemented with 10% bovine serum albumin (BSA), 100 µg/mL penicillin, and 100 µg/mL streptomycin at 37 ℃ in 5% CO2.
Transfection
A miRNA mimic and a miRNA inhibitor were obtained from Biomics (Nantong, China). Short hairpin RNAs (shRNAs) of NPNT were constructed on the PLKO.1 plasmid. The shRNA sequence was shRNA NPNT (shNPNT) 5'-GCTACTGTCTGAATGGCTACA-3'. The antisense oligonucleotides (ASOs) of lncRNA LINC00957 were purchased from RiboBio (Guangzhou, China). The miRNA mimic, inhibitor, shRNA, and ASOs were transfected into U87 and LN229 cells using Lipofectamine 2000 (Invitrogen, Waltham, MA, USA) according to the manufacturer’s instructions.
RNA extraction and reverse transcription-quantitative polymerase chain reaction (RT-qPCR) assay
We extracted the total RNA and converted it into complementary DNA (cDNA) using reverse transcriptase. We then measured the levels of RNA and miRNA using a qPCR kit. The primers used are listed in Table S1.
Cell Counting Kit-8 (CCK8) assay
We cultured the cells in 96-well plates and used CCK8 reagent to measure the proliferation potential of the cells at 450 nM using a microplate reader.
Cell cycle assay
The U87 cells with shRNA negative control (shNC) or stable NPNT knockdown (1×106) were cultured in 6-well plates. The cells were washed with phosphate-buffered saline (PBS) and fixed overnight with 70% ethanol at 4 ℃. Finally, the cells were stained with propidium iodide (PI) and detected by flow cytometry.
Transwell assay
ASO NC and ASO LINC00957 U87 cells were cultured in serum-free medium. Following this, 1×104 cells were cultured in the upper layer of the chamber (BD Biosciences, Shanghai, China), and serum-containing medium was added to the lower layer. After 48 h, the cells were washed with PBS, fixed with 0.1% crystal violet solution, and observed randomly under a microscope in five fields.
ROS assay
The production of ROS was detected using a ROS assay kit (Beyotime, Shanghai, China) according to the manufacturer’s instructions. shNC and shNPNT U87 and LN229 cells were seeded in 12-well plates. After 24 h, the cells were treated with different concentrations of elesclomol (0, 10, and 20 µM) for 24 h at 37 ℃. Next, the cells were incubated for 20 min with a dichlo-rodihydroflurescein diacetate probe (10 µM) at 37 ℃. ROS fluorescence signals were measured using BD C6 (BD Biosciences).
Measurement of intracellular copper
U87 and LN229 cells were cultured in 6 cm plates for 24 h and treated with a cuproptosis inducers (elesclomol) for 24 h. Following this, the cells were collected and disrupted ultrasonically to detect intracellular copper according to the manufacturer’s instructions (E-BC-K775-M, Elabscience, Houston, TX, USA).
Luciferase reporter assay
We used PGL4.15 to construct plasmids containing wild-type and mutated LINC00957. A Dual-Luciferase Reporter Assay System Kit (E1910; Promega, Madison, WI, USA) was used according to the manufacturer’s instructions.
Statistical analysis
We used univariate regression analysis and Kaplan-Meier (KM) and receiver operating characteristic (ROC) curves to evaluate the patient survival status. We used GraphPad Prism 8 to analyze the statistical significance of the experimental results. Unpaired t-test was used to evaluate the results between the two groups. P values <0.05 were considered statistically significant.
Results
Identification of lncRNAs, miRNAs, and mRNAs associated with GBM progression
Tumor markers are genes that play a vital role in tumor occurrence and development and show significant changes in their expression levels in tumors. In this study, differential mRNA and lncRNA analysis was performed on five pairs of GBM samples and normal brain tissue samples from the TCGA database. Similarly, we performed differential miRNA analysis on four pairs of GBM samples and normal brain tissue samples from the GEO database (GSE63319). A total of 4,694 differentially expressed mRNAs (2,286 upregulated and 2,408 downregulated), 536 lncRNAs (380 upregulated and 156 downregulated), and 50 miRNAs (43 upregulated and 7 downregulated) (Figure 1A,1B and Figure S1) were identified. Subsequently, univariate Cox analysis was performed using 170 GBM tumor samples from the TCGA database, using which we identified 838 oncogenes and 192 tumor suppressor genes (Figure 1C). To further investigate the significance of these genes in GBM tumors, the mRNAs were subjected to GSEA. Interestingly, these DEGs were found to be expressed at high levels in the cell proliferation and cell migration pathways (Figure 2A and Figure S2A-S2C). Results of the gene ontology (GO) enrichment analysis yielded similar results, indicating that these genes were primarily involved in the proliferation and cell migration functions of GBM tumors (Figure 2B and Figure S2D-S2F). Collectively, the genes regulating the malignant progression of GBM tumors showed significant enrichment in pathways related to the cell cycle and cell migration.
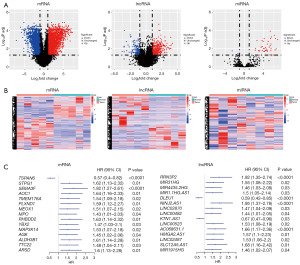
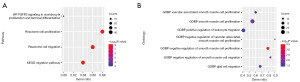
Consensus clustering analysis of CRGs
Cuproptosis is a copper-dependent programmed cell death pathway. However, the function of CRGs in GBM remains unclear. Hence, we performed clustering analysis using the expression matrix of CRGs to categorize 170 patients from the TCGA database into k groups. The optimal classification was achieved when patients were divided into two groups based on ConsensusClusterPlus (Figure 3A and Figure S3A,S3B). Additionally, DEGs were identified in the mRNA data of the two subgroups, with 256 upregulated and 678 downregulated genes (Figure 3B and Figure S3C). Furthermore, GO analysis of these DEGs revealed their significant enrichment in the cell cycle and cell migration pathways (Figure 3C,3D and Figure S3).
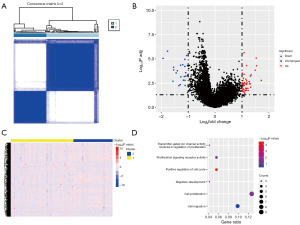
Identifying cuproptosis-related ceRNA axis in GBM tumorigenesis
Based on the above results, we constructed a ceRNA network associated with the cuproptosis-related regulation of malignant progression in GBM, using differentially expressed mRNA, lncRNA, and miRNA, which included 24 lncRNAs, three miRNAs, six mRNAs, and 50 interacting axes (Figure 4A and Figure S4A,S4B). Surprisingly, we identified NPNT, a potential prognostic biomarker for GBM. Literature reports suggest that NPNT can promote cancer cell proliferation, migration, and invasion. However, its mechanism of action in GBM remains unclear. Therefore, we focused on the ceRNA axes associated with NPNT. The results indicated that 15 lncRNAs may be involved in the regulation of NPNT expression (Figure 4B). Among them, AL365361.1, AL591848.3, and LINC00957 exhibited higher expression levels in GBM tumors, suggesting their greater potential functionality. Consequently, we selected these lncRNAs for further screening.
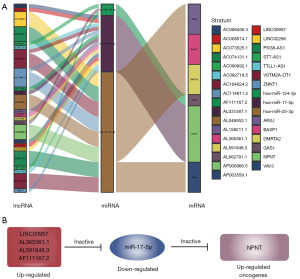
NPNT knockdown enhances the sensitivity of GBM cells to cuproptosis inducers
Based on previous findings, NPNT was observed to be associated with the progression of GBM. However, the potential involvement of NPNT in cuproptosis pathways has not been reported. To better understand the relationship between NPNT and cuproptosis, we treated GBM cells with the cuproptosis inducer elesclomol and measured its half-maximal inhibitory concentration (IC50) value using the CCK8 assay (Figure 5A). Subsequently, we treated NPNT knockdown cells and control cells with varying concentrations (0, 10, and 20 nM) of elesclomol and assessed the changes in the cellular ROS levels using flow cytometry. Lower concentrations of elesclomol significantly reduced the ROS levels in NPNT knockdown cells compared to those in the control group (Figure 5B). To further confirm the association between ROS levels and cuproptosis, we measured the Cu2+ concentrations in both groups of cells, and found that knockdown of NPNT resulted in an increase in intracellular copper ion concentration when GBM cells were induced at low concentrations of elesclomol (Figure 5C). Collectively, the results indicated that NPNT knockdown enhanced the sensitivity of GBM cells to elesclomol and provided further evidence supporting the link between ROS levels and cuproptosis.
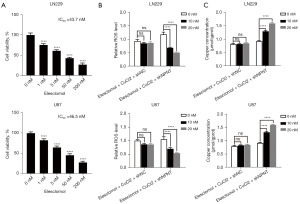
LINC00957 potentially regulates the nucleic acid levels of NPNT
To determine the primary lncRNA regulating NPNT, we conducted an ROC analysis. The area under the curve (AUC) indicated a significant association between the expression of LINC00957 and the survival rate (Figure 6A). To further refine our findings, we performed knockdown experiments using ASOs targeting AL365361.1, AL591848.3, AF111167.2, and LINC00957, and measured the changes in NPNT mRNA levels. LINC00957 knockdown led to a significant alteration in NPNT expression, whereas no statistically significant differences were observed with the knockdown of the other lncRNAs (Figure 6B). Collectively, these findings emphasize the significant role of LINC00957 in regulating NPNT expression.
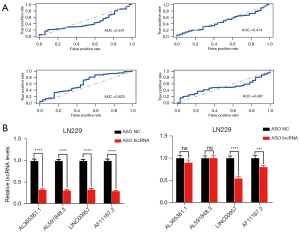
Regulation of GBM malignant progression by LINC00957, miR-17-5p, and NPNT protein
According to the aforementioned predictions, NPNT/miR-17-5p/LINC00957 may be a ceRNA network that regulates the development of GBM, but the specific roles of the network in promoting the malignant progression of GBM remain unclear. Hence, we divided GBM samples into two groups based on the expression levels of LINC00957. We performed differential expression analysis and enrichment analysis (similar analyses were performed for NPNT). Our findings revealed that the target genes regulated by LINC00957 were primarily enriched in cell migration, while NPNT was enriched in cell cycle (Figure 7A and Figure S5A-S5C). To further validate the regulatory roles of NPNT and LINC00957 in GBM malignant progression, we conducted knockdown experiments targeting NPNT and LINC00957 individually. Transwell assays and cell cycle analyses were performed, revealing that the knockdown of NPNT and LINC00957 inhibited GBM cell cycle progression and migration, consistent with the findings of the enrichment analysis (Figure 7B-7D). These results confirmed that NPNT and LINC00957 can indeed regulate the cell cycle and cell migration in GBM and supported the involvement of these molecules in the malignant progression of the disease.
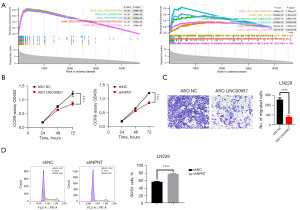
MiR-17-5p as a ceRNA for LINC00957 regulates NPNT protein expression to influence malignant GBM progression
To further investigate the relationship among LINC00957, miR-17-5p, and NPNT, we conducted Pearson correlation analysis. The results showed a high correlation among the expression of these molecules, indicating a significant association between the three genes (Figure 8A). Next, we altered the expression of LINC00957 or miR-17-5p and used RT-PCR to measure the expression levels of the three genes. LNC00957 and miR-17-5p could regulate NPNT expression in GBM, which was consistent with the ceRNA axis hypothesis (Figure 8B). To verify whether LINC00957 acts as the ceRNA of miR-17-5p, we conducted the dual luciferase reporter assay was conducted after introducing complementary mutations in the predicted binding sites between LINC00957 and miR-17-5p. Our findings revealed that the luciferase activity of wild-type LINC00957 was regulated by miR-17-5p, but the mutant form of LINC00957 did not show any changes (Figure 8C,8D). Furthermore, we assessed the DEGs co-regulated by LINC00957 and NPNT in GBM (Figure S6A,S6B). We conducted proliferation in GBM cells with LINC00957 knockdown in both control and shNPNT groups. The findings indicated that LINC00957 knockdown suppressed tumor cell proliferation specifically in the shNPNT group, but not in the control group (Figure 8E). These results suggest that LINC00957 acts as a ceRNA for miR-17-5p, thereby regulating NPNT protein expression and influencing the malignant progression of GBM tumors.
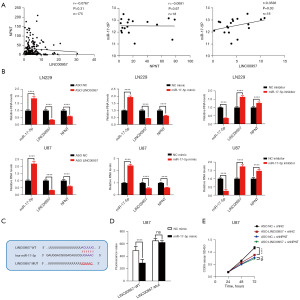
Discussion
GBM is the most aggressive and lethal form of brain cancer, characterized by poor prognosis, high malignancy, and a dire lack of effective treatments. However, despite significant advancements in surgery, radiation, and chemotherapy, the survival rate in GBM remains dismally low, emphasizing the indispensable requirement for novel diagnostic and therapeutic approaches. The complex biology of GBM, including its rapid growth, invasion of adjacent brain tissues, and resistance to conventional therapies, poses significant challenges in treatment. An expanding area of interest in GBM research is the function of non-coding RNAs, particularly lncRNAs and miRNAs, in its pathogenesis. These molecules are crucial regulators in the occurrence and development of GBM, functioning primarily via the ceRNA mechanism. The ceRNA hypothesis suggests that lncRNAs can act as molecular sponges, sequestering miRNAs and preventing them from downregulating their target mRNAs (29). This intricate system of lncRNA-miRNA-mRNA interactions contributes to the regulation of gene expression, thereby impacting key processes in cancer biology like cell proliferation, apoptosis, and metastasis (30). CeRNA function in GBM has been the focus of several studies. A study examined the lncRNA, miRNA, and mRNA-associated ceRNA networks to identify potential prognostic indicators for GBM, highlighting the importance of ceRNA network genes in GBM diagnosis and treatment (31). Collectively, the findings from these studies contribute to the understanding of the regulatory mechanisms and functions of ceRNA networks in the pathogenesis of GBM (31). In this study, we developed a ceRNA regulatory network specific to GBM. We confirmed that LINC00957, acting as a ceRNA for miR-17-5p, shows significance in regulating the malignant progression of GBM. This study substantially expands our understanding of the ceRNA regulatory mechanisms in GBM.
Cuproptosis is a specific cell death pathway mediated via copper accumulation within cells. Cuproptosis is distinct from apoptosis or necrosis in that it entails the direct interaction of copper with lipoylated elements of the tricarboxylic acid (TCA) cycle (32). This interaction leads to the accumulation of lipoylated proteins, which consequently disrupts iron-sulfur cluster proteins that are essential for mitochondrial function (33). This disruption leads to the loss of mitochondrial respiration, leading to cell death. In cancer, where cells often exhibit altered copper metabolism and mitochondrial function, the exploitation of cuproptosis presents a unique therapeutic avenue (34). By selectively inducing cuproptosis, it is possible to target tumor cells, offering a potential strategy for cancer treatment, particularly in case of tumors that have developed resistance to traditional treatments (35). The effect of CRGs on GBM has been a subject of interest in recent research. For example, a systematic analysis and bioinformatics studies highlighted the link between CRG expression and immune infiltration in GBM and its potential implications for prognosis (36). In another study, a cuproptosis network was developed to determine the interactions and prognostic implications of CRGs in GBM, providing insights into the role in the disease (37). Furthermore, the activation of cuproptosis was associated with prognosis in GBM, with the expression of CRGs being linked to tumor immune infiltration and the immune microenvironment (38). These results enhance our understanding of the unique impact of cuproptosis on GBM and its potential influence on disease prognosis and therapeutic strategies. Nevertheless, the precise role of cuproptosis in the evolution and advancement of GBM warrants additional research, given the existing ambiguities in this area. In this context, GBM samples were classified according to the expression levels of 13 CRGs, which facilitated the segregation of patients into two different groups characterized by varying degrees of cuproptosis. Subsequent differential analysis helped identify specific genes associated with cuproptosis in GBM. These insights offer promising targets for developing targeted therapies for cuproptosis in GBM treatment.
The NPNT gene encodes NPNT, which plays a crucial role in tumor cell apoptosis and migration (39). NPNT reduces the migration and invasive potential of cancer cells, such as ovarian and cervical cancer cells, by suppressing specific pathways and decreasing protein expression in cell motility (40). Additionally, various studies have demonstrated the ability of certain compounds to influence cancer cell proliferation, migration, and apoptosis by targeting signaling pathways, including the PI3K/AKT pathway, which is central to the control of cell physiology (41,42). Therefore, NPNT and its associated signaling pathways play a crucial in regulating tumor cell behavior, making them potential targets for therapeutic intervention. However, its mechanism of action in GBM has not been clarified, and a relationship with cuproptosis has never been reported. In the ceRNA network, NPNT may form an integral part of a complex regulatory mechanism in cancer biology (43). In the case of GBM, understanding how ceRNA interactions regulate NPNT can help gain insights into tumor progression and potential therapeutic targets. For example, if a particular lncRNA is found to regulate NPNT expression through a ceRNA mechanism, targeting the lncRNA could be a strategy to control NPNT levels and, consequently, impact GBM progression. The abovementioned studies established a co-regulatory network of NPNT and non-coding RNA in GBM, providing a theoretical basis for targeted NPNT diagnosis and treatment.
Conclusions
Herein, we discovered that LINC00957 serves as a ceRNA for miR-17-5p in GBM, thereby influencing the expression of the CRG NPNT. We first assessed copper ion levels and ROS, determining that NPNT inhibition enhances cuproptosis. Further, enrichment analyses and phenotypic tests revealed that the LINC00957/miR-17-5p/NPNT axis impacts the GBM cell cycle and migration. From a mechanistic perspective, the findings of our reporter gene assays indicate that LINC00957 modulates NPNT expression by acting as a ceRNA for miR-17-5p. Our findings reveal a ceRNA network linked to cuproptosis in GBM, offering novel insights for GBM diagnosis and therapy.
Acknowledgments
Funding: None.
Footnote
Reporting Checklist: The authors have completed the MDAR reporting checklist. Available at https://tcr.amegroups.com/article/view/10.21037/tcr-24-450/rc
Data Sharing Statement: Available at https://tcr.amegroups.com/article/view/10.21037/tcr-24-450/dss
Peer Review File: Available at https://tcr.amegroups.com/article/view/10.21037/tcr-24-450/prf
Conflicts of Interest: All authors have completed the ICMJE uniform disclosure form (available at https://tcr.amegroups.com/article/view/10.21037/tcr-24-450/coif). The authors have no conflicts of interest to declare.
Ethical Statement: The authors are accountable for all aspects of the work in ensuring that questions related to the accuracy or integrity of any part of the work are appropriately investigated and resolved. The study was conducted in accordance with the Declaration of Helsinki (as revised in 2013).
Open Access Statement: This is an Open Access article distributed in accordance with the Creative Commons Attribution-NonCommercial-NoDerivs 4.0 International License (CC BY-NC-ND 4.0), which permits the non-commercial replication and distribution of the article with the strict proviso that no changes or edits are made and the original work is properly cited (including links to both the formal publication through the relevant DOI and the license). See: https://creativecommons.org/licenses/by-nc-nd/4.0/.
References
- Janjua TI, Rewatkar P, Ahmed-Cox A, et al. Frontiers in the treatment of glioblastoma: Past, present and emerging. Adv Drug Deliv Rev 2021;171:108-38. [Crossref] [PubMed]
- Brandao M, Simon T, Critchley G, et al. Astrocytes, the rising stars of the glioblastoma microenvironment. Glia 2019;67:779-90. [Crossref] [PubMed]
- Zhang H, Wang R, Yu Y, et al. Glioblastoma Treatment Modalities besides Surgery. J Cancer 2019;10:4793-806. [Crossref] [PubMed]
- Marenco-Hillembrand L, Wijesekera O, Suarez-Meade P, et al. Trends in glioblastoma: outcomes over time and type of intervention: a systematic evidence based analysis. J Neurooncol 2020;147:297-307. [Crossref] [PubMed]
- Noch EK, Ramakrishna R, Magge R. Challenges in the Treatment of Glioblastoma: Multisystem Mechanisms of Therapeutic Resistance. World Neurosurg 2018;116:505-17. [Crossref] [PubMed]
- Bhatti GK, Khullar N, Sidhu IS, et al. Emerging role of non-coding RNA in health and disease. Metab Brain Dis 2021;36:1119-34. [Crossref] [PubMed]
- Soni DK, Biswas R. Role of Non-Coding RNAs in Post-Transcriptional Regulation of Lung Diseases. Front Genet 2021;12:767348. [Crossref] [PubMed]
- Kashyap D, Tuli HS, Garg VK, et al. Oncogenic and Tumor-Suppressive Roles of MicroRNAs with Special Reference to Apoptosis: Molecular Mechanisms and Therapeutic Potential. Mol Diagn Ther 2018;22:179-201. [Crossref] [PubMed]
- Bi J, Chowdhry S, Wu S, et al. Altered cellular metabolism in gliomas - an emerging landscape of actionable co-dependency targets. Nat Rev Cancer 2020;20:57-70. [Crossref] [PubMed]
- Wang Z, Chen X, Liang Q, et al. Inhibiting of circ-TLK1 inhibits the progression of glioma through down-regulating PANX1 via targeting miR-17-5p. J Mol Histol 2021;52:1007-20. [Crossref] [PubMed]
- Zeng A, Yin J, Wang Z, et al. miR-17-5p-CXCL14 axis related transcriptome profile and clinical outcome in diffuse gliomas. Oncoimmunology 2018;7:e1510277. [Crossref] [PubMed]
- Zhang X, Wang W, Zhu W, et al. Mechanisms and Functions of Long Non-Coding RNAs at Multiple Regulatory Levels. Int J Mol Sci 2019;20:5573. [Crossref] [PubMed]
- Liu SJ, Dang HX, Lim DA, et al. Long noncoding RNAs in cancer metastasis. Nat Rev Cancer 2021;21:446-60. [Crossref] [PubMed]
- Movahedpour A, Khatami SH, Khorsand M, et al. Exosomal noncoding RNAs: key players in glioblastoma drug resistance. Mol Cell Biochem 2021;476:4081-92. [Crossref] [PubMed]
- Li X, Xu M, Ding L, et al. MiR-27a: A Novel Biomarker and Potential Therapeutic Target in Tumors. J Cancer 2019;10:2836-48. [Crossref] [PubMed]
- Zhang LH, Li LH, Zhang PF, et al. LINC00957 Acted as Prognostic Marker Was Associated With Fluorouracil Resistance in Human Colorectal Cancer. Front Oncol 2019;9:776. [Crossref] [PubMed]
- Qin Y, Zhang X, Chen Y, et al. Prognostic Analysis of a Hypoxia-Associated lncRNA Signature in Glioblastoma and its Pan-Cancer Landscape. J Neurol Surg A Cent Eur Neurosurg 2024;85:378-88. [Crossref] [PubMed]
- Cheng J, Meng J, Zhu L, et al. Exosomal noncoding RNAs in Glioma: biological functions and potential clinical applications. Mol Cancer 2020;19:66. [Crossref] [PubMed]
- Koren E, Fuchs Y. Modes of Regulated Cell Death in Cancer. Cancer Discov 2021;11:245-65. [Crossref] [PubMed]
- Tsui KH, Hsiao JH, Lin LT, et al. The Cross-Communication of Cuproptosis and Regulated Cell Death in Human Pathophysiology. Int J Biol Sci 2024;20:218-30. [Crossref] [PubMed]
- Chen L, Min J, Wang F. Copper homeostasis and cuproptosis in health and disease. Signal Transduct Target Ther 2022;7:378. [Crossref] [PubMed]
- Tong X, Tang R, Xiao M, et al. Targeting cell death pathways for cancer therapy: recent developments in necroptosis, pyroptosis, ferroptosis, and cuproptosis research. J Hematol Oncol 2022;15:174. [Crossref] [PubMed]
- Qi YL, Wang HR, Chen LL, et al. Recent advances in small-molecule fluorescent probes for studying ferroptosis. Chem Soc Rev 2022;51:7752-78. [Crossref] [PubMed]
- Xie J, Yang Y, Gao Y, et al. Cuproptosis: mechanisms and links with cancers. Mol Cancer 2023;22:46. [Crossref] [PubMed]
- Teo AE, Garg S, Johnson TI, et al. Physiological and Pathological Roles in Human Adrenal of the Glomeruli-Defining Matrix Protein NPNT (Nephronectin). Hypertension 2017;69:1207-16. [Crossref] [PubMed]
- Qian G, Liao Q, Li G, et al. miR-378 associated with proliferation, migration and apoptosis properties in A549 cells and targeted NPNT in COPD. PeerJ 2022;10:e14062. [Crossref] [PubMed]
- Sun Y, Kuek V, Qiu H, et al. The emerging role of NPNT in tissue injury repair and bone homeostasis. J Cell Physiol 2018;233:1887-94. [Crossref] [PubMed]
- Huang L, Guan S, Feng L, et al. Integrated analysis identified NPNT as a potential key regulator in tumor metastasis of hepatocellular carcinoma. Gene 2022;825:146436. [Crossref] [PubMed]
- Thomson DW, Dinger ME. Endogenous microRNA sponges: evidence and controversy. Nat Rev Genet 2016;17:272-83. [Crossref] [PubMed]
- Tang XJ, Wang W, Hann SS. Interactions among lncRNAs, miRNAs and mRNA in colorectal cancer. Biochimie 2019;163:58-72. [Crossref] [PubMed]
- Zhu X, Jiang L, Yang H, et al. Analyzing the lncRNA, miRNA, and mRNA-associated ceRNA networks to reveal potential prognostic biomarkers for glioblastoma multiforme. Cancer Cell Int 2020;20:393. [Crossref] [PubMed]
- Wang J, Luo LZ, Liang DM, et al. Progress in the research of cuproptosis and possible targets for cancer therapy. World J Clin Oncol 2023;14:324-34. [Crossref] [PubMed]
- Li Y, Du Y, Zhou Y, et al. Iron and copper: critical executioners of ferroptosis, cuproptosis and other forms of cell death. Cell Commun Signal 2023;21:327. [Crossref] [PubMed]
- Zhang X, Tao T, Qiu Y, et al. Copper-mediated novel cell death pathway in tumor cells and implications for innovative cancer therapies. Biomed Pharmacother 2023;168:115730. [Crossref] [PubMed]
- Wang W, Mo W, Hang Z, et al. Cuproptosis: Harnessing Transition Metal for Cancer Therapy. ACS Nano 2023;17:19581-99. [Crossref] [PubMed]
- Bao JH, Lu WC, Duan H, et al. Identification of a novel cuproptosis-related gene signature and integrative analyses in patients with lower-grade gliomas. Front Immunol 2022;13:933973. [Crossref] [PubMed]
- Chen S, Zhang S, Yuan Y, et al. Prognostic value of cuproptosis-related genes signature and its impact on the reshaped immune microenvironment of glioma. Front Pharmacol 2022;13:1016520. [Crossref] [PubMed]
- Lu H, Zhou L, Zhang B, et al. Cuproptosis key gene FDX1 is a prognostic biomarker and associated with immune infiltration in glioma. Front Med (Lausanne) 2022;9:939776. [Crossref] [PubMed]
- Wilson CL, Hung CF, Schnapp LM. Integrin α8 and Its Ligand Nephronectin in Health and Disease. In: Gullberg D, Eble JA. editors. Integrins in Health and Disease: Key Effectors of Cell-Matrix and Cell-Cell Interactions. Cham: Springer International Publishing; 2023:185-216.
- Chhichholiya Y, Ruthuparna M, Velagaleti H, et al. Brain metastasis in breast cancer: focus on genes and signaling pathways involved, blood-brain barrier and treatment strategies. Clin Transl Oncol 2023;25:1218-41. [Crossref] [PubMed]
- He Y, Sun MM, Zhang GG, et al. Targeting PI3K/Akt signal transduction for cancer therapy. Signal Transduct Target Ther 2021;6:425. [Crossref] [PubMed]
- Rascio F, Spadaccino F, Rocchetti MT, et al. The Pathogenic Role of PI3K/AKT Pathway in Cancer Onset and Drug Resistance: An Updated Review. Cancers (Basel) 2021;13:3949. [Crossref] [PubMed]
- Chen L, Wei K, Li J, et al. Integrated Analysis of LncRNA-Mediated ceRNA Network in Calcific Aortic Valve Disease. Cells 2022;11:2204. [Crossref] [PubMed]