Causal relationship between circulating immune cells and gastric cancer: a bidirectional Mendelian randomization analysis using UK Biobank and FinnGen datasets
Highlight box
Key findings
• The absolute numbers of CD4−CD8− T cells and IgD−CD27− B cells were positively correlated with the development of gastric cancer.
• The percentage of IgD+CD24− B cells in lymphocytes was negatively associated with the development of gastric cancer.
• Gastric cancer was not causally associated with any of the immune cell phenotypes in the reverse Mendelian randomization (MR) analysis.
What is known and what is new?
• The immune system plays a crucial role in cancer immune surveillance, but the relationship between circulating immune cells and gastric cancer remains largely unknown.
• This study used bidirectional two-sample MR to demonstrate that certain immune cell traits, including CD4−CD8− T cells, IgD−CD27− B cells, and IgD+CD24− B cells, are causally related to gastric cancer risk.
What is the implication, and what should change now?
• The findings provide insight into the relationship between immune cells and gastric cancer pathogenesis, which may aid in the development of new immunotherapy targets for gastric cancer.
• Further research is required to confirm these causal relationships and investigate the underlying mechanisms between the identified immune cell traits and gastric cancer.
Introduction
Globally, gastric cancer is the fifth most common type of cancer, with over a million cases diagnosed each year (1). There is a poor prognosis for gastric cancer, and it is the third leading cause of cancer-related deaths (1,2). Currently, surgery and chemotherapy are the most commonly used treatments for gastric cancer (3-6). Immunotherapy is an emerging therapy that has been shown to have a number of advantages, including durable responses, long-term survival benefits, and a lower level of toxicity (7-10). Understanding the relationship between the immune system and gastric cancer may assist in the development of new immunotherapy targets.
The immune system of the host plays an important role in the immune surveillance of cancer and is capable of killing cancer cells through both innate and adapted immune responses (11). Despite this, there are contradictory reports regarding the role of immune cells in cancer pathogenesis (12-15). In gastric cancer, tumor-infiltrating dendritic cells and natural killer cells are generally associated with improved prognosis and enhanced anti-tumor immunity (16). In contrast, effector regulatory T cells and regulatory B cells can promote immune escape, leading to poorer outcomes (7). The prognostic implications of cytotoxic T cells (CD8+) are particularly complex (17-19); while some studies link high CD8+ T cell infiltration to better survival rates, others associate it with worse prognoses. These discrepancies likely arise from the intricate interplay between different immune cell subpopulations, the tumor microenvironment, and the stage of cancer progression. Thus, elucidating the causal relationships between immune cells and gastric cancer is crucial. However, traditional observational studies are often confounded by factors such as reverse causality, making it challenging to establish definitive causal links.
Mendelian randomization (MR) is an emerging genetic epidemiological method based on Mendel’s genetic inheritance laws (20). MR uses genetic variations as instrumental variants (IVs), which are randomly assigned at conception prior to disease onset (21). Therefore, MR can be used to identify causal relationships independent of confounding factors and avoid reverse causality (22-24). In recent years, MR analysis has increasingly been utilized to identify possible risk factors for gastric cancer (25-27). Several MR studies have also examined the causal relationship between inflammatory biomarkers and gastric cancer, including interleukin-6 (28), interleukin-10 (29), and C-reactive protein (30).
While previous studies have primarily focused on the impact of immune cells on cancer development, emerging evidence suggests that cancer itself can influence immune cell populations and function. This bidirectional relationship between cancer and the immune system is known as cancer immunoediting (31). During this process, the tumor can shape the immune response, potentially leading to changes in circulating immune cell populations (31,32). Therefore, investigating the bidirectional relationship between the immune cells and gastric cancer is crucial for understanding the complex interplay between them.
However, to our knowledge, no MR study has examined the possible causal link between circulating immune cells and gastric cancer risk. Therefore, in this study, we conducted a bidirectional two-sample MR analysis using large-scale genome-wide association study (GWAS) data to determine the causal relationship between immune cell traits and gastric cancer. We present this article in accordance with the STROBE-MR reporting checklist (available at https://tcr.amegroups.com/article/view/10.21037/tcr-24-480/rc).
Methods
Study design
This is a bidirectional two-sample MR study. In the forward MR analyses, immune cell traits were considered as exposures and gastric cancer was considered as outcome, whereas in the reverse MR analyses, gastric cancer was considered as exposure and immune cell traits as outcome. MR uses single nucleotide polymorphisms (SNPs) as IVs, and the valid IVs should fulfil the following three assumptions (33): first, IVs should be robustly associated with exposure (the relevance assumption); second, IVs should be independent of any confounding factors (the independence assumption); third, IVs affect outcome only through the risk factor, but not via other pathways (the exclusion limitation assumption). Figure 1 illustrates our study design.
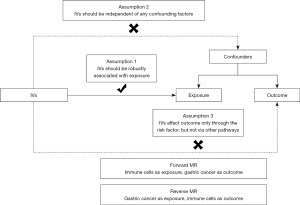
Data sources
GWAS-summary statistics for each immune trait are available through the GWAS Catalog (accession numbers GCST90001391 to GCST90001700) (34). A total of 310 immune cell phenotypes were analyzed, including absolute cell counts (n=118) and relative cell counts (n=192). The GWAS on immune traits was performed using data from 3,757 European individuals. Peripheral blood samples were collected from the donors in heparin tubes. Circulating immune cells were tested using flow cytometry, where the blood samples were stained with specific antibodies and analyzed with BD FACSCanto II flow cytometers (34). The data were processed using BD FACSDiva software, and cell populations were manually gated to ensure consistency (34). The GWAS included 20,143,392 SNPs, and the associations were examined after adjusting for covariates such as sex, age, and age2 (35). GWAS-summary statistics regarding gastric cancer are available through the GWAS Catalog (accession number GCST90018849) (36). The statistics included 24,188,662 SNPs from 476,116 European individuals across two large-scale biobanks: the UK Biobank and FinnGen. In the UK Biobank cohort, gastric cancer cases were identified through linkage with national cancer registries (37). In the FinnGen cohort, gastric cancer cases were ascertained using a combination of nationwide health registries, including the Finnish Cancer Registry, the Care Register for Health Care, and the Cause of Death Register (38). Identification of gastric cancer was facilitated by corresponding International Classification of Diseases, 9th Revision (ICD-9), and 10th Revision (ICD-10) codes. Ethical approval for this project was not required as the data analyzed were publicly available, and each source of data had already obtained ethical approval from their respective institutions. The study was conducted in accordance with the Declaration of Helsinki (as revised in 2013).
Selection of IVs
We employed a systematic approach to select IVs for each immune trait and gastric cancer. For immune traits, we extracted significant SNPs at a threshold of P<1×10−5 (39,40), while for gastric cancer GWAS data, we used a more stringent threshold of P<5×10−8. To ensure independence among selected SNPs, we performed linkage disequilibrium (LD)-based clumping using PLINK. For immune traits, we applied an r2 threshold of 0.1 within a 500 kb window (39), whereas for gastric cancer, we used a stricter r2 threshold of 0.0001 within a 1,000 kb window. LD calculations were based on the 1,000 Genomes Project reference panel (41). To assess instrument strength, we calculated the F statistic for each SNP and included only those with F>10 to mitigate weak instrument bias (42). Furthermore, we utilized Phenoscanner V2 to exclude SNPs directly associated with potential confounders or outcomes (43,44), ensuring the validity of our selected IVs. This comprehensive selection process aimed to identify robust and independent IVs for our MR analysis.
Statistical analysis
To examine causal associations between different immune cell phenotypes and gastric cancer, we conducted five analyses: inverse variance weighting (IVW), MR Egger, weighted median, simple mode, and weighted mode, among which the IVW method (random effects) was the primary analysis (45). These methods make different assumptions about pleiotropy and instrument validity: IVW assumes all instruments are valid or that pleiotropic effects are balanced (46); MR Egger allows for directional pleiotropy and provides a test for its presence (47); weighted median is robust when up to 50% of the weight comes from invalid instruments (48); simple mode assumes that the most common estimate is the true causal effect (49); weighted mode provides a more robust estimate when there is heterogeneity among the SNPs (50). We used multiple methods to assess the robustness of our findings across different assumptions. Since there are multiple tests in this study, we used the false discovery rate (FDR) correction to avoid the likelihood of type 1 errors, and a PFDR<0.2 was considered as significant (39,51). The Cochran’s Q statistic was used to assess the heterogeneity among selected IVs, and a P value <0.05 indicates the existence of heterogeneity (45). The MR-Egger intercept test was employed to evaluate horizontal pleiotropy. If the MR-Egger intercept was significant (P<0.05), it suggested that association results may be influenced by horizontal pleiotropic effects of other traits (52). Forest plots and scatter plots were generated to visualize our results. Moreover, the leave-one-out sensitivity test was performed to test for the influence of a single SNP on the overall causal estimates. The statistical analyses were conducted with R version 4.3.1 (http://www.Rproject.org).
Results
Exploration of the causal effect of immune cell phenotypes on gastric cancer
We first used the IVW method to conduct MR analysis. The results showed that 27 immune cell phenotypes were associated with gastric cancer (P<0.05) (Figure 2). After FDR adjustment, three immune characteristics showed a causal association with gastric cancer (PFDR<0.2), namely: the number of CD4−CD8− T cells, the number of IgD−CD27− B cells and the percentage of IgD+CD24− B cells in lymphocytes.
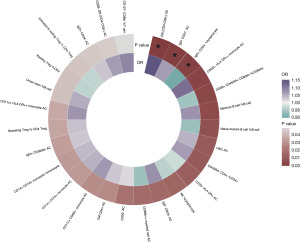
The results showed that the absolute numbers of IgD−CD27− B cells and CD4−CD8− T cells were positively related to the development of gastric cancer, with odd ratios (ORs) of 1.15 [95% confidence interval (CI), 1.07–1.24; P<0.001; PFDR=0.041] and 1.07 (95% CI, 1.03–1.11; P=0.001; PFDR=0.187). However, IgD+CD24− B cells in lymphocytes (OR =0.90; 95% CI: 0.84–0.96; P=0.002; PFDR=0.187) were negatively associated with gastric cancer development (Figure 3).
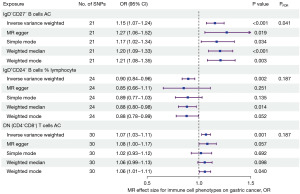
In addition, we performed MR analysis using four other methods: the MR Egger, weighted median, simple mode, and weighted mode (Figure 3). Four methods yielded similar results regarding the causal relationship between CD4−CD8− T cells and gastric cancer. In regard to the number of IgD−CD24− B cells, only the weighted mode method provided comparable results to the IVW method. In terms of the percentage of IgD+CD27+ B cells in lymphocytes, only the weighted median method yielded comparable results to the IVW method. Regardless, the causal relationships suggested by the various MR methods are consistent with those suggested by the IVW method. Figure 4A-4C illustrate the scatter plots for the MR analysis of these immune cell phenotypes.
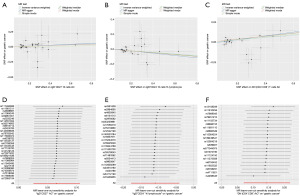
While conducting MR analysis on the above three immune cell phenotypes, no significant heterogeneity (Cochran’s Q statistic, P>0.05) was observed (Table 1). Based on the MR-Egger-intercept analysis, there was no significant horizontal pleiotropy (P>0.05) for each immune cell phenotype (Table 1), suggesting that the SNPs did not influence the outcome via factors unrelated to the exposure factors. Moreover, the leave-one-out sensitivity test showed that the overall causal estimates were not affected by individual SNPs (Figure 4D-4F).
Table 1
Exposure | P value (Q-Egger) | P value (Q-IVW) | Egger-intercept | P value (Egger-intercept) |
---|---|---|---|---|
IgD−CD27− B cells AC | 0.562 | 0.516 | −0.003 | 0.712 |
IgD+CD24− B cells %lymphocyte | 0.401 | 0.353 | 0.008 | 0.704 |
DN (CD4−CD8−) T cells AC | 0.255 | 0.271 | −0.017 | 0.282 |
AC, absolute count; DN, double negative; IVW, inverse variance weighted.
For the other immune cell phenotypes, https://cdn.amegroups.cn/static/public/tcr-24-480-1.xlsx provides the results of MR analysis, and https://cdn.amegroups.cn/static/public/tcr-24-480-2.xlsx shows the results of heterogeneity and horizontal pleiotropy.
Exploration of the causal effect of gastric cancer on immune cell phenotypes
The IVW method was first used to conduct MR analysis. Following FDR adjustment, gastric cancer showed no causal association with any of the immune cell phenotypes (PFDR>0.2). Gastric cancer did not have a causal effect on the number of CD4−CD8− T cells, the number of IgD−CD27− B cells, or the percentage of IgD+CD24− B cells in lymphocytes, which was also demonstrated by the four other methods (MR Egger, weighted median, simple mode, and weighted mode) (Figure 5).
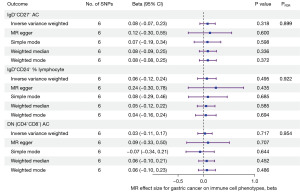
The https://cdn.amegroups.cn/static/public/tcr-24-480-3.xlsx presents MR results, and the https://cdn.amegroups.cn/static/public/tcr-24-480-4.xlsx presents heterogeneity and horizontal pleiotropy results for all immune cell phenotypes.
Discussion
In the present study, we used MR to investigate the causal relationship between 310 immune cell traits and gastric cancer. Our study demonstrated that the absolute number of CD4−CD8− T cells and the absolute number of IgD−CD27− B cells were positively related to the development of gastric cancer, whereas the percentage of IgD+CD24− B cells in lymphocytes were negatively associated with gastric cancer development. Moreover, we found that gastric cancer did not have a causal effect on these immune cell phenotypes.
According to our study, peripheral blood CD4−CD8− T cell counts are positively associated with the development of gastric cancer. In the human body, the majority of adaptive immune cells contain either CD4 or CD8 as coreceptors. However, there is a small subpopulation of T lymphocytes that lack both CD4 and CD8 coreceptors which is called double-negative T cells (53). This subpopulation accounts for approximately 3–5% of peripheral blood T lymphocytes (54). It has been demonstrated that double-negative T cells are crucial to orchestrating immune responses. Numerous studies have examined the role of double-negative T cells in the development and progression of tumors. Lu et al. demonstrated that human double-negative T cells reduced pancreatic cancer cell proliferation and infiltration in co-culture (55). Double-negative T cells also showed enhanced cytotoxicity against lung cancer-derived cells in vitro (56). In some studies, activating double-negative T cells is suggested to be an alternative therapy for the treatment of leukemia (57,58). However, several studies reported inconsistent findings. Prins et al. found that decreasing the number of double-negative T cells improved host survival in murine glioma and melanoma models, indicating the immunosuppressive properties of double-negative T cells (59). Furthermore, double-negative T cells were shown to contribute to tumor metastasis. An increase in double-negative T cells was observed in the lymph nodes of melanoma patients with disease progression as compared to those without progression (60,61). In view of these contradictory conclusions, it seems that double-negative T cells may be either pro-tumor or anti-tumor depending on the specific microenvironment and type of cancer (53). To date, little is known about the role of double-negative T cells in the development and progression of gastric cancer. Our results indicate a positive correlation between the number of double-negative T cells and the development of gastric cancer. However, further research will be required to confirm this finding.
Furthermore, we found that IgD−CD27− B cell counts were positively associated with a higher risk of gastric cancer. IgD−CD27− B cells are a rare subset of B cells known as double-negative B cells. They constitute approximately 5% of all peripheral B cells (62). Although IgD−CD27− B cells are relatively rare in normal peripheral blood, they play an important role in a number of diseases, including systemic lupus erythematosus (63), coronavirus disease 2019 (COVID-19) (64), and malaria (65). Their functions include defending the host against pathogens as well as initiating autoimmune responses (66). IgD−CD27− B cells have been reported to play an immunosuppressive role in the tumor microenvironment in some types of cancer. Centuori et al. demonstrate that infiltrating IgD−CD27− B cells are enriched within the microenvironment of non-small cell lung cancer tumors, and that these cells are associated with tumor differentiation (67). Similarly, nasopharyngeal carcinoma is also enriched with IgD−CD27− B cells, and the presence of IgD−CD27− B cells is related to poorer clinical outcomes (68). These findings suggest that IgD−CD27− B cells may be a potential immunotherapeutic target for these cancers. As of yet, no study has examined the role that double-negative B cells play in the development and progression of gastric cancer. According to our study, double-negative B-cell count was positively related to gastric cancer risk. These results are consistent with previous findings regarding other types of cancer, however more research is needed to illustrate the role and underlying mechanisms of IgD−CD27− B cells in cancer development.
We also found that a higher percentage of IgD+CD24− B cells was associated with a lower risk of gastric cancer. The causality of this association is yet to be established by previous studies, but a number of studies have provided indirect evidence (69). Yang et al. examined the proportion of CD24-high-expressing B cells in the peripheral blood of patients with gastric cancer, and found that the proportion of these cells was lower in patients with non-progressive gastric cancer than in those with progression (69). Further research found that these cells are capable of suppressing the proliferation of autologous CD4+ T cells and inhibiting the production of interferon-gamma by CD4+ T cells (70). Thus, CD24 expression on B cells may contribute to immune suppression in gastric cancer. IgD is an antibody that is expressed on the surface of antigen-naive B cells (62). The main function of IgD is to control the survival, differentiation, and further utilization of the low-affinity autoreactive B cells that recognize structurally distinct antigens (71,72). The expression of IgD may counteract the restrictive pressure placed on the naive B cell receptor, thereby precipitating an adaptive immune response (73). Based on these findings, IgD+CD24− B cells may be capable of exerting anticancer immunity.
In the present study, we used large-scale GWAS data, examined the association between as many as 310 immune cell traits and gastric cancer and provided genetic evidence for the causal relationship between circulating immune cells and gastric cancer. However, there are several limitations in this study. First, the study was based on a European database, and the results may not be generalizable to other ethnic groups. Second, while we observed a causal relationship between certain immune cells and gastric cancer, the underlying mechanisms are still largely unknown, so our findings should be interpreted with caution.
Conclusions
Our study demonstrated that circulating CD4−CD8− T cells and IgD−CD27− B cells were positively correlated with the development of gastric cancer, while the percentage of IgD+CD24− B cells in lymphocytes were negatively correlated. These findings provide insight into the relationship between immune cells and the pathogenesis of gastric cancer. Moreover, our findings may also serve as a basis for the development of immunotherapies for gastric cancer. Further research, however, is required to confirm these causal relationships, as well as investigating the underlying mechanisms.
Acknowledgments
Funding: This work was supported by
Footnote
Reporting Checklist: The authors have completed the STROBE-MR reporting checklist Available at https://tcr.amegroups.com/article/view/10.21037/tcr-24-480/rc
Peer Review File: Available at https://tcr.amegroups.com/article/view/10.21037/tcr-24-480/prf
Conflicts of Interest: All authors have completed the ICMJE uniform disclosure form (available at https://tcr.amegroups.com/article/view/10.21037/tcr-24-480/coif). The authors have no conflicts of interest to declare.
Ethical Statement: The authors are accountable for all aspects of the work in ensuring that questions related to the accuracy or integrity of any part of the work are appropriately investigated and resolved. The study was conducted in accordance with the Declaration of Helsinki (as revised in 2013).
Open Access Statement: This is an Open Access article distributed in accordance with the Creative Commons Attribution-NonCommercial-NoDerivs 4.0 International License (CC BY-NC-ND 4.0), which permits the non-commercial replication and distribution of the article with the strict proviso that no changes or edits are made and the original work is properly cited (including links to both the formal publication through the relevant DOI and the license). See: https://creativecommons.org/licenses/by-nc-nd/4.0/.
References
- Sung H, Ferlay J, Siegel RL, et al. Global Cancer Statistics 2020: GLOBOCAN Estimates of Incidence and Mortality Worldwide for 36 Cancers in 185 Countries. CA Cancer J Clin 2021;71:209-49. [Crossref] [PubMed]
- Global Burden of Disease 2019 Cancer Collaboration; Kocarnik JM, Compton K, et al. Cancer Incidence, Mortality, Years of Life Lost, Years Lived With Disability, and Disability-Adjusted Life Years for 29 Cancer Groups From 2010 to 2019: A Systematic Analysis for the Global Burden of Disease Study 2019. JAMA Oncol 2022;8:420-44.
- Delgado-Ramos GM, Fitzsimons J, Dhanarajan A. A narrative review of the evolving landscape of the management of metastatic gastric cancer: the role of targeted therapies. J Gastrointest Oncol 2023;14:2600-16. [Crossref] [PubMed]
- Pinheiro RN, Mucci S, Zanatto RM, et al. Influence of the centralizing gastric cancer surgery on the health-related quality of life in Brazil. J Gastrointest Oncol 2023;14:1235-49. [Crossref] [PubMed]
- Nevo Y, Ferri L. Current management of gastric adenocarcinoma: a narrative review. J Gastrointest Oncol 2023;14:1933-48. [Crossref] [PubMed]
- Zhang Y, Xu X, Hu C, et al. Trastuzumab in combination with chemotherapy for HER2-positive metastatic gastric cancer patients underwent conversion therapy. Transl Cancer Res 2022;11:2145-56. [Crossref] [PubMed]
- Zhao Y, Bai Y, Shen M, et al. Therapeutic strategies for gastric cancer targeting immune cells: Future directions. Front Immunol 2022;13:992762. [Crossref] [PubMed]
- Li S, Gao J, Xu Q, et al. A Signature-Based Classification of Gastric Cancer That Stratifies Tumor Immunity and Predicts Responses to PD-1 Inhibitors. Front Immunol 2021;12:693314. [Crossref] [PubMed]
- Tan S, Li D, Zhu X. Cancer immunotherapy: Pros, cons and beyond. Biomed Pharmacother 2020;124:109821. [Crossref] [PubMed]
- Liu Z, Yang M, Shu H, et al. A novel prognostic and therapeutic target biomarker based on complement-related gene signature in gastric cancer. Transl Cancer Res 2023;12:3565-80. [Crossref] [PubMed]
- Chen DS, Mellman I. Oncology meets immunology: the cancer-immunity cycle. Immunity 2013;39:1-10. [Crossref] [PubMed]
- Sukri A, Hanafiah A, Kosai NR. The Roles of Immune Cells in Gastric Cancer: Anti-Cancer or Pro-Cancer? Cancers (Basel) 2022;14:3922. [Crossref] [PubMed]
- Wang W, Wang Y, Cao Z. Changes of proportions of circulating lymphocyte subsets in cancer patients after chemotherapy. Transl Cancer Res 2021;10:4169-79. [Crossref] [PubMed]
- Yin Y, Wang B, Yang M, et al. Gastric cancer prognosis: unveiling autophagy-related signatures and immune infiltrates. Transl Cancer Res 2024;13:1479-92. [Crossref] [PubMed]
- Chen S, Ben X, Guo L, et al. Identification of lncRNAs based on different patterns of immune infiltration in gastric cancer. J Gastrointest Oncol 2022;13:102-16. [Crossref] [PubMed]
- Hargadon KM. Tumor-altered dendritic cell function: implications for anti-tumor immunity. Front Immunol 2013;4:192. [Crossref] [PubMed]
- Lee HE, Chae SW, Lee YJ, et al. Prognostic implications of type and density of tumour-infiltrating lymphocytes in gastric cancer. Br J Cancer 2008;99:1704-11. [Crossref] [PubMed]
- Thompson ED, Zahurak M, Murphy A, et al. Patterns of PD-L1 expression and CD8 T cell infiltration in gastric adenocarcinomas and associated immune stroma. Gut 2017;66:794-801. [Crossref] [PubMed]
- Tan MP, Pedersen J, Zhan Y, et al. CD8+ T cells are associated with severe gastritis in Helicobacter pylori-infected mice in the absence of CD4+ T cells. Infect Immun 2008;76:1289-97. [Crossref] [PubMed]
- Davies NM, Holmes MV, Davey Smith G. Reading Mendelian randomisation studies: a guide, glossary, and checklist for clinicians. BMJ 2018;362:k601. [Crossref] [PubMed]
- Lawlor DA, Harbord RM, Sterne JA, et al. Mendelian randomization: using genes as instruments for making causal inferences in epidemiology. Stat Med 2008;27:1133-63. [Crossref] [PubMed]
- Davey Smith G, Hemani G. Mendelian randomization: genetic anchors for causal inference in epidemiological studies. Hum Mol Genet 2014;23:R89-98. [Crossref] [PubMed]
- Ran S, Yao J, Liu B. The association between sarcopenia and cirrhosis: a Mendelian randomization analysis. Hepatobiliary Surg Nutr 2023;12:291-3. [Crossref] [PubMed]
- Gao L, Di X, Gao L, et al. The Frailty Index and colon cancer: a 2-sample Mendelian-randomization study. J Gastrointest Oncol 2023;14:798-805. [Crossref] [PubMed]
- Yuan S, Chen J, Ruan X, et al. Smoking, alcohol consumption, and 24 gastrointestinal diseases: Mendelian randomization analysis. Elife 2023;12:e84051. [Crossref] [PubMed]
- Yin L, Yan H, Chen K, et al. Diet-Derived Circulating Antioxidants and Risk of Digestive System Tumors: A Mendelian Randomization Study. Nutrients 2022;14:3274. [Crossref] [PubMed]
- Tan Y, Wei Z, Liu K, et al. Lifestyle habits and gastric cancer in an East Asian population: a Mendelian randomization study. Front Oncol 2023;13:1224753. [Crossref] [PubMed]
- Yang Z, Guo L, Sun Y, et al. Investigation of the causal relationship between Interleukin-6 signaling and gastrointestinal tract cancers: A Mendelian randomization study. Dig Liver Dis 2024;56:679-86. [Crossref] [PubMed]
- Niu W, Pang Q, Lin T, et al. A Causal Role of Genetically Elevated Circulating Interleukin-10 in the Development of Digestive Cancers: Evidence from Mendelian Randomization Analysis Based on 29,307 Subjects. Medicine (Baltimore) 2016;95:e2799. [Crossref] [PubMed]
- Zhu M, Ma Z, Zhang X, et al. C-reactive protein and cancer risk: a pan-cancer study of prospective cohort and Mendelian randomization analysis. BMC Med 2022;20:301. [Crossref] [PubMed]
- Gubin MM, Vesely MD. Cancer Immunoediting in the Era of Immuno-oncology. Clin Cancer Res 2022;28:3917-28. [Crossref] [PubMed]
- Zhou C, Guo L, Cai Q, et al. Circulating neutrophils activated by cancer cells and M2 macrophages promote gastric cancer progression during PD-1 antibody-based immunotherapy. Front Mol Biosci 2023;10:1081762. [Crossref] [PubMed]
- Sanderson E, Glymour MM, Holmes MV, et al. Mendelian randomization. Nat Rev Methods Primers 2022;2:6. [Crossref] [PubMed]
- Orrù V, Steri M, Sidore C, et al. Complex genetic signatures in immune cells underlie autoimmunity and inform therapy. Nat Genet 2020;52:1036-45. [Crossref] [PubMed]
- Sidore C, Busonero F, Maschio A, et al. Genome sequencing elucidates Sardinian genetic architecture and augments association analyses for lipid and blood inflammatory markers. Nat Genet 2015;47:1272-81. [Crossref] [PubMed]
- Sakaue S, Kanai M, Tanigawa Y, et al. A cross-population atlas of genetic associations for 220 human phenotypes. Nat Genet 2021;53:1415-24. [Crossref] [PubMed]
- Marley J, Nicholl BI, Macdonald S, et al. Associations between long-term conditions and upper gastrointestinal cancer incidence: A prospective population-based cohort of UK Biobank participants. J Multimorb Comorb 2021;11:26335565211056136. [Crossref] [PubMed]
- Kurki MI, Karjalainen J, Palta P, et al. FinnGen provides genetic insights from a well-phenotyped isolated population. Nature 2023;613:508-18. [Crossref] [PubMed]
- Wang C, Zhu D, Zhang D, et al. Causal role of immune cells in schizophrenia: Mendelian randomization (MR) study. BMC Psychiatry 2023;23:590. [Crossref] [PubMed]
- Gu J, Yan GM, Kong XL, et al. Assessing the causal relationship between immune traits and systemic lupus erythematosus by bi-directional Mendelian randomization analysis. Mol Genet Genomics 2023;298:1493-503. [Crossref] [PubMed]
- 1000 Genomes Project Consortium; Auton A, Brooks LD, et al. A global reference for human genetic variation. Nature 2015;526:68-74.
- Tan Z, Zhu S, Liu C, et al. Causal Link between Inflammatory Bowel Disease and Fistula: Evidence from Mendelian Randomization Study. J Clin Med 2023;12:2482. [Crossref] [PubMed]
- Kamat MA, Blackshaw JA, Young R, et al. PhenoScanner V2: an expanded tool for searching human genotype-phenotype associations. Bioinformatics 2019;35:4851-3. [Crossref] [PubMed]
- Meng Y, Tan Z, Liu C, et al. Association between Inflammatory Bowel Disease and Iridocyclitis: A Mendelian Randomization Study. J Clin Med 2023;12:1282. [Crossref] [PubMed]
- Burgess S, Small DS, Thompson SG. A review of instrumental variable estimators for Mendelian randomization. Stat Methods Med Res 2017;26:2333-55. [Crossref] [PubMed]
- Slob EAW, Burgess S. A comparison of robust Mendelian randomization methods using summary data. Genet Epidemiol 2020;44:313-29. [Crossref] [PubMed]
- Bowden J, Davey Smith G, Burgess S. Mendelian randomization with invalid instruments: effect estimation and bias detection through Egger regression. Int J Epidemiol 2015;44:512-25. [Crossref] [PubMed]
- Bowden J, Davey Smith G, Haycock PC, et al. Consistent Estimation in Mendelian Randomization with Some Invalid Instruments Using a Weighted Median Estimator. Genet Epidemiol 2016;40:304-14. [Crossref] [PubMed]
- Hartwig FP, Davey Smith G, Bowden J. Robust inference in summary data Mendelian randomization via the zero modal pleiotropy assumption. Int J Epidemiol 2017;46:1985-98. [Crossref] [PubMed]
- Qi G, Chatterjee N. Mendelian randomization analysis using mixture models for robust and efficient estimation of causal effects. Nat Commun 2019;10:1941. [Crossref] [PubMed]
- Ran B, Qin J, Wu Y, et al. Causal role of immune cells in chronic obstructive pulmonary disease: Mendelian randomization study. Expert Rev Clin Immunol 2024;20:413-21. [Crossref] [PubMed]
- Burgess S, Thompson SG. Interpreting findings from Mendelian randomization using the MR-Egger method. Eur J Epidemiol 2017;32:377-89. [Crossref] [PubMed]
- Wu Z, Zheng Y, Sheng J, et al. CD3(+)CD4(-)CD8(-) (Double-Negative) T Cells in Inflammation, Immune Disorders and Cancer. Front Immunol 2022;13:816005. [Crossref] [PubMed]
- Fischer K, Voelkl S, Heymann J, et al. Isolation and characterization of human antigen-specific TCR alpha beta+ CD4(-)CD8- double-negative regulatory T cells. Blood 2005;105:2828-35. [Crossref] [PubMed]
- Lu Y, Hu P, Zhou H, et al. Double-negative T Cells Inhibit Proliferation and Invasion of Human Pancreatic Cancer Cells in Co-culture. Anticancer Res 2019;39:5911-8. [Crossref] [PubMed]
- Fang L, Ly D, Wang SS, et al. Targeting late-stage non-small cell lung cancer with a combination of DNT cellular therapy and PD-1 checkpoint blockade. J Exp Clin Cancer Res 2019;38:123. [Crossref] [PubMed]
- Lee J, Minden MD, Chen WC, et al. Allogeneic Human Double Negative T Cells as a Novel Immunotherapy for Acute Myeloid Leukemia and Its Underlying Mechanisms. Clin Cancer Res 2018;24:370-82. [Crossref] [PubMed]
- Velikkakam T, Gollob KJ, Dutra WO. Double-negative T cells: Setting the stage for disease control or progression. Immunology 2022;165:371-85. [Crossref] [PubMed]
- Prins RM, Incardona F, Lau R, et al. Characterization of defective CD4-CD8- T cells in murine tumors generated independent of antigen specificity. J Immunol 2004;172:1602-11. [Crossref] [PubMed]
- Greenplate AR, McClanahan DD, Oberholtzer BK, et al. Computational Immune Monitoring Reveals Abnormal Double-Negative T Cells Present across Human Tumor Types. Cancer Immunol Res 2019;7:86-99. [Crossref] [PubMed]
- Vallacchi V, Vergani E, Camisaschi C, et al. Transcriptional profiling of melanoma sentinel nodes identify patients with poor outcome and reveal an association of CD30(+) T lymphocytes with progression. Cancer Res 2014;74:130-40. [Crossref] [PubMed]
- Li Y, Li Z, Hu F. Double-negative (DN) B cells: an under-recognized effector memory B cell subset in autoimmunity. Clin Exp Immunol 2021;205:119-27. [Crossref] [PubMed]
- Jenks SA, Cashman KS, Zumaquero E, et al. Distinct Effector B Cells Induced by Unregulated Toll-like Receptor 7 Contribute to Pathogenic Responses in Systemic Lupus Erythematosus. Immunity 2018;49:725-739.e6. [Crossref] [PubMed]
- Woodruff MC, Ramonell RP, Nguyen DC, et al. Extrafollicular B cell responses correlate with neutralizing antibodies and morbidity in COVID-19. Nat Immunol 2020;21:1506-16. [Crossref] [PubMed]
- Hopp CS, Skinner J, Anzick SL, et al. Atypical B cells up-regulate costimulatory molecules during malaria and secrete antibodies with T follicular helper cell support. Sci Immunol 2022;7:eabn1250. [Crossref] [PubMed]
- Chung MKY, Gong L, Kwong DL, et al. Functions of double-negative B cells in autoimmune diseases, infections, and cancers. EMBO Mol Med 2023;15:e17341. [Crossref] [PubMed]
- Centuori SM, Gomes CJ, Kim SS, et al. Double-negative (CD27(-)IgD(-)) B cells are expanded in NSCLC and inversely correlate with affinity-matured B cell populations. J Transl Med 2018;16:30. [Crossref] [PubMed]
- Gong L, Kwong DL, Dai W, et al. Comprehensive single-cell sequencing reveals the stromal dynamics and tumor-specific characteristics in the microenvironment of nasopharyngeal carcinoma. Nat Commun 2021;12:1540. [Crossref] [PubMed]
- Yang J, Li W, Luo F, et al. Low percentage of CD24hiCD27+CD19+ B cells decelerates gastric cancer progression in XELOX-treated patients. Int Immunopharmacol 2015;26:322-7. [Crossref] [PubMed]
- Murakami Y, Saito H, Shimizu S, et al. Increased regulatory B cells are involved in immune evasion in patients with gastric cancer. Sci Rep 2019;9:13083. [Crossref] [PubMed]
- Noviski M, Zikherman J. Control of autoreactive B cells by IgM and IgD B cell receptors: maintaining a fine balance. Curr Opin Immunol 2018;55:67-74. [Crossref] [PubMed]
- Hobeika E, Maity PC, Jumaa H. Control of B Cell Responsiveness by Isotype and Structural Elements of the Antigen Receptor. Trends Immunol 2016;37:310-20. [Crossref] [PubMed]
- Dirks J, Andres O, Paul L, et al. IgD shapes the pre-immune naïve B cell compartment in humans. Front Immunol 2023;14:1096019. [Crossref] [PubMed]