Preparation and anticancer activity of telomerase inhibitor TAT-LPTS39 polypeptide
Highlight box
Key findings
• The polypeptide TAT-LPTS39 is prepared and delivered into the cells, where it inhibits the cell growth of telomerase-positive tumour cells.
What is known and what is new?
• Although the C-terminal fragment 290-328 of LPTS/PinX1 has been reported to inhibit telomerase activity, its effect on tumour growth remains uncertain.
• TAT-LPTS39 inhibits telomerase activity and the growth of human telomerase-positive cancer cells in vitro and in vivo.
What is the implication, and what should change now?
• TAT-LPTS39 with the effect of inhibiting cancer cell growth, has a prospect in anticancer drug development.
Introduction
Telomeres are located at the ends of linear chromosomes in eukaryotic cells and consist of a series of simple tandem and repeated 5'-TTAGGG-3' nucleotide sequences and related proteins, which play a role in stabilizing chromosomes (1). Due to linear DNA end replication problems, every division of normal cells is accompanied by shortening of telomeres. Critically short telomeres lead to genomic instability, causing cellular senescence and organismal ageing (2,3). Telomerase, which is composed of the catalytic subunit telomerase reverse transcriptase (TERT) and the telomerase RNA subunit telomerase RNA component (TERC), can synthesize telomeric DNA sequences using its own RNA as a template, compensating for the loss of telomeres during cell division to maintain telomere length and genomic stability (4,5). Telomerase is not expressed or expressed at a low level in normal cells except stem cells and male/female germline cells (6). However, it is highly expressed in most tumour cells (7). The telomere length of tumour cells is usually shorter than that of normal cells, but the growth rate is much higher than that of normal cells (8). Therefore, in theory, once telomerase activity is inhibited, tumour cells die faster than normal cells (9). Based on this, telomerase is considered a potential antitumour target.
LPTS, a liver-related candidate tumour suppressor gene, is located in the human 8p23.1 region, with a high frequency of chromosome complex deletion (10). LPTS expression is observed in all human normal tissues but is reduced or absent in many types of tumour cells (11,12). LPTS is associated with the occurrence of various cancers, such as liver, gastric, colorectal, lung, cervical cancers (10,13-16). Overexpression of LPTS can significantly inhibit telomerase activity, shorten telomeres, and inhibit the growth of tumour cells, indicating that LPTS has therapeutic potential for cancer (17-19).
Sequence analysis showed that LPTS and the telomeric repeat DNA binding factor Pin2/TRF1 interaction protein PinX1 are actually derived from the same gene (20). With the forced exogenous expression of the LPTS/PinX1 gene in cancer cells, such as hepatocellular carcinoma (HCC) and urothelial carcinoma of the bladder cells, cellular telomerase activity and cells proliferation were inhibited in vitro and in vivo (10,21). These experimental results show that the LPTS/PinX1 gene encodes an inhibitory protein of telomerase activity. The telomere length of tumour cells cannot be maintained; thus, cells enter the crisis period and eventually die. More importantly, more than 94% of PinX1 heterozygous deletion mice (PinX1 +/−) developed tumours in vivo (22), so LPTS may be a tumour suppressor and used as an anticancer drug.
Chen et al. reported that the C-terminal 290-328 amino acid (aa) was the core region that inhibited telomerase activity, and the N-terminal 1-289 aa had no obvious inhibitory effect (23). In this study, the recombinant protein GST-TAT-LPTS39 was constructed to fuse the C-terminal 290-328 aa of LPTS and the cell-penetrating peptide TAT (11 aa peptide of HIV transcription activator of transcription protein), and a GST tag was attached at the N-terminal of TAT-LPTS39 for purification. Purified GST-TAT-LPTS39 entered cells rapidly, inhibited tumour cell growth and induced cell death. Then we removed the GST tag to obtain the polypeptide TAT-LPTS39, which could also inhibit tumour cell growth in vitro and in vivo. These results indicate that TAT-LPTS39 is a promising anticancer protein drug. We present this article in accordance with the ARRIVE and MDAR reporting checklists (available at https://tcr.amegroups.com/article/view/10.21037/tcr-24-792/rc).
Methods
Cell culture and reagents
Three human telomerase-positive liver cancer cell lines BEL-7404, QGY-7701 and PLC-PRF-5, and one human telomerase-positive cervical cancer cell line Hela, were purchased from the Chinese Academy of Sciences Cell Bank (Shanghai, China). All cells were cultured in Dulbecco’s modified medium (DMEM) containing 10% foetal bovine serum (Gibco, Waltham, MA, USA) and 1% streptomycin and penicillin with a humidified atmosphere at 37 ℃ in 5% CO2.
Plasmid construction
The TAT sequence was inserted into pGEX-6p-1 (Addgene, Watertown, MA, USA) to generate the recombinant plasmid pGEX-6p-1-TAT, and then the cDNA encoding LPTS290-328 was cloned and inserted into the pGEX-6p-1-TAT vector to generate the plasmid GST-TAT-LPTS290-328 by the primers (F: 5'-CGGGATCCACCCTGAAGCCCAAAAAG-3'; R: 5'-CCCTCGAGTTTGGAATCTTTCTTCTTCTTC-3'). Green fluorescent protein (GFP) was cloned and inserted into pTAT to generate pTAT-GFP (a kind gift from M.Z.) (24).
Protein expression and purification
The recombinant plasmid was transferred into E. coli BL21(DE3) (Beyotime Biotech, Inc., Shanghai, China), and the culture was expanded with LB liquid medium (37 ℃, 200 rpm). When the bacterial concentration reached OD600=0.8, IPTG at a final concentration of 0.5 mM was added to induce protein expression (37 ℃, 200 rpm, 3 h). After 3 h of incubation, the cells were harvested (8,000 rpm, 15 min). The harvested cells were lysed by ultrasonication in buffer (50 mM Tris-HCl, 150 mM NaCl, pH 7.5). The supernatant was centrifuged (15,000 rpm, 30 min), and the supernatant containing GST-TAT-LPTS39 protein was purified by GST affinity chromatography columns (Beyotime Biotech, Inc.), further purified using size-exclusion chromatography Superdex200 (GE Healthcare, Shanghai, China), and tested for protein purity using sodium dodecyl sulfate-polyacrylamide gel electrophoresis (SDS-PAGE).
Delivery of fusion proteins into cells
Cells were seeded in 6-well plates, and when the cell density reached 90%, the medium for cultured cells was replaced every two days with fresh medium supplemented with GST-TAT-LPTS39 or phosphate buffered saline (PBS, 137 mM NaCl, 2.7 mM KCl, 10 mM Na2HPO4, and 1.8 mM KH2PO4, pH 7.4), as described in reference (24).
Western blot and immunofluorescence
Cells were lysed in SDS-PAGE electrophoresis sample buffer and separated by SDS-PAGE followed by Western blot using anti-LPTS/PinX1 antibody (Santa Cruz Biotechnology, Inc., Santa Cruz, CA, USA, sc-376588) and anti-mouse tubulin antibody (Santa Cruz Biotechnology, Inc., sc-73242). For the immunofluorescence assay, all treated cells were extensively washed with PBS, immobilized in cold methanol for 3 min, permeated with PBS for 10 min, and then sealed with new goat serum in PBS for 10 min. The cells were then incubated with primary anti-LPTS/PinX1 antibodies for 60 min, rinsed three times with PBS, and incubated with secondary fluorescein isothiocyanate-labelled goat anti-rabbit antibodies (Santa Cruz Biotechnology, sc-2356). The cells were then cleaned and incubated with 4',6-diamidino-2-phenylindole (DAPI) for 10 min to stain the nucleus. After cleaning, the lid was sealed, and the cells were observed under a fluorescence microscope (Olympus BX51, Shanghai, China).
Telomeric repeat amplification protocol (TRAP) assay
Cell lysates containing the GST-TAT-LPTS39 protein were quantified by total protein quantification and diluted to 1 mg/mL. Then, the samples were diluted to 5–7 concentration gradients, and TRAP experiments were performed to analyse their telomerase activity as follows: the reaction was performed in a polymerase chain reaction (PCR) tube. The total volume was 50 µL, containing the reaction buffer, cell lysate, dNTPs, Taq enzyme and Ts primers (5'-AATCCGTCGAGCAGAGTT-3'), at 25 ℃ extension for 30 min. Then, an Acx primer [5'-(CCCTTA) 3CCCTAA-3'] was added. PCR was performed (95 ℃ for 5 min; 30 cycles: 94 ℃ for 30 s, 50 ℃ for 30 s, 72 ℃ for 30 s; 72 ℃ for 2 min). The products were separated by SDS-PAGE with TBE buffer in the electrophoresis system with silver stain (25).
Cell growth assay
The cells were continuously cultured with PBS, T-GFP (TAT-GFP) or GST-TAT-LPTS39 for 3 to 6 weeks, then the cells (1–2 ×103/100 µL) were seeded on 96-well culture plates and treated with the corresponding protein or PBS solution. The cells were incubated for 7 days at 37 ℃ in 5% CO2 and saturated humidity. Every day for 1 week, 20 µL of MTT solution [3-(4,5-dimethylthiazol-2-yl)-2,5-diphenyltetrazolium bromide] at a concentration of 5 mg/mL was added to each well. After 4 h incubation, the culture supernatant was carefully discarded. Dimethyl sulfoxide (DMSO; 100 µL) was added to each well, and then the cells were transferred to another new 96-well culture plate. A wavelength of 490 nm was selected to measure the light absorption value of each well. The results were recorded, and the cell growth curves were drawn with time as the abscissa and absorbance value as the ordinate.
Flow cytometry analysis
Cells were seeded at 1×103 in 96-well culture plates and treated with PBS, TAT-GFP (T-GFP, 40 µg/mL), or GST-TAT-LPTS39 (10, 40, 100, 200 or 300 µg/mL) for 72 h, 1 to 2 weeks, or 6 weeks. After incubation, the cells were fixed with prechilled 70% ethanol at 4 ℃ for 30 min. After washing with PBS, the cells were treated with 100 µg/mL RNaseA and 50 µg/mL propidium iodide, incubated at room temperature for 30 min, and the cells were counted using a FACSCalibur flow cytometer (BD, New Jersey, USA).
β-galactosidase staining (β-gal)
The cells were treated with PBS or 200 µg/mL GST-TAT-LPTS39 for 6 weeks, then detected by a senescence β-Galactosidase Staining Kit (Beyotime) according to the manufacturer’s protocol. Briefly, cells were fixed for 15 min at room temperature and then washed three times and stained with β-gal dye for 12 h. The stained cells were viewed under a microscope, and counted by ImageJ software (version 1.8.0).
Cell culture doubling time
Cells were continuously cultured for five to 6 weeks with PBS or 2 µM TAT-LPTS39 according to previous methods (26). Cells were passaged every three to seven days with the same number of cells cultured in each passage. The cell number was counted per passage, and the cell growth was curved. The doubling time (Dt) and the different Dt (ΔDt) are calculated.
Measurement of the relative telomere length (RTL)
The RTL was measured as described in the reference (26). The genomic DNA was extracted from cell by using FFPE DNA kits (Jiangsu Cowin Biotech Co., Ltd., Beijing, China), before diluting to 10 ng/µL. Then two pairs of primers (GC-Telc and GC-Telg, MRef1F and MRef1R) were added to each reaction system. The RTL was measured through quantitative real-time polymerase chain reaction (qPCR; TB Green® Premix Ex Taq™ II, Tli RNase H Plus, TaKaRa, Beijing, China) and Ct and ΔCt were calculated.
Animal studies
All animal procedures were performed under a project license (No. 20210601) granted by institutional committee of the Huzhou University, in compliance with all national or institutional guidelines for the care and use of animals. A protocol was prepared before the study without registration. Male BALB/c nude mice (4- to 5-week-old, 13–17 g, GemPharmatech LLC, Nanjing, China) were raised in standard environment, 20–26 ℃, humidity 40–70%, air changes 15–20 times/h, 12 h light/dark cycle with free access to food and water. In cell derived xenograft (CDX) experiments, the liver cancer cell line BEL-7404 were injected (2×106 cells per site) subcutaneously into the right flank of mice. One day after cell implantation, the mice were randomized into three groups (five mice per group) and then injected with PBS, 20 mg/kg T-GFP or TAT-LPTS39 via the tail vein. The injections were performed 15 times every three days. Tumour size was measured weekly with calipers, and tumour volume was calculated as follows: volume = (length × width2) × 0.5. The tumours were then collected and weighed 7 weeks after inoculation.
Statistical analysis
The cell experiments were run in triplicate, and the animal experiments were run in quintuplicate, all these n-numbers were just technical replicates. All data were presented as the mean ± standard deviation (SD). Differences between two groups were evaluated using Student’s t-test. and P values <0.05 were considered statistically significant. All the data were processed using GraphPad Prism software (version 10.1.2).
Results
Expression and purification of the recombinant protein GST-TAT-LPTS39
Since the constructed TAT-LPTS39 recombinant protein clone was expressed with a GST tag, we initially purified the protein using a GST affinity chromatography column and verified the purified samples by SDS-PAGE. After purification on the GST column, the protein was concentrated and further purified by size-exclusion chromatography Superdex200, as shown in Figure 1A. The molecular weight of GST-TAT-LPTS39 was approximately 31 kDa, and the protein purity was high, with almost no impurity protein (Figure 1B). We then used the TRAP assay to examine the telomerase inhibitory activity of the purified protein GST-TAT-LPTS39 for four cell lines (BEL-7404, QGY-7701, Hela and PLC-PRF-5), with a total of 50 µL of the reaction system. Different amounts of GST-TAT-LPTS39 protein were added to the reaction system. The results showed that the protein had telomerase inhibition, and with increasing concentration, the inhibitory strength of the protein on telomerase activity increased gradually. When the amount of protein reached 100 ng, telomerase activity was almost completely inhibited (Figure 1C,1D). In the control group with 1,000 ng GST protein, telomerase activity was unaffected, and the telomere extended normally (Figure 1D).
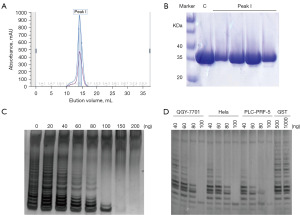
Delivery of the GST-TAT-LPTS39 protein into cells
In a previous study, the C-terminal of LPTS was fused to the TAT—an 11-aa peptide that translocates across the cell membrane. TAT-LPTS-LC was efficiently delivered into the cells (24). BEL-7404 cells were incubated with GST-TAT-LPTS39 to test the ability of TAT to carry GST-TAT-LPTS39 into cells. The Western blot results indicated that the GST-TAT-LPTS39 fusion protein was rapidly introduced into the cell and could be detected in the cell after two hours of incubation and remained stable for at least 24 hours (Figure 2A). Immunofluorescence experiments also showed that GST-TAT-LPTS39 protein fluorescence was observed in the cells after two hours of incubation and that the fluorescence gradually accumulated in the nucleus over time, whereas the background expression of LPTS/PinX1 protein was barely observed in the PBS group (Figure 2B). The above experimental results showed that TAT could carry LPTS39 into cells.
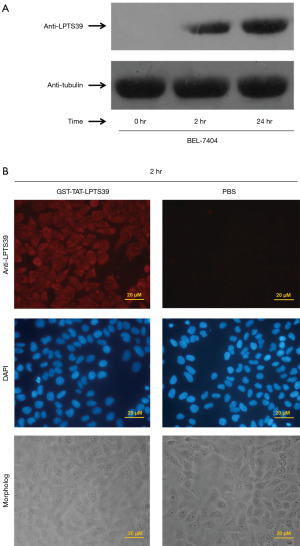
GST-TAT-LPTS39 inhibited telomerase-positive tumour cell growth
When conducting cell culture, we observed that cells expressing the GST-TAT-LPTS39 protein grew slowly compared to the untreated cells. To further verify this phenomenon, we examined the cell growth rate using the MTT assay. The results showed that the GST-TAT-LPTS39 protein could significantly inhibit the growth of telomerase-positive liver cancer cells BEL-7404 and QGY-7701 compared with PBS and T-GFP control. Compared with the PBS group, the growth rate of BEL-7404 cells treated with GST-TAT-LPTS39 decreased to 68.8% (10 µg/mL), 60.8% (40 µg/mL), 40.9% (100 µg/mL), and 18.1% (200 µg/mL) (Figure 3A). The growth rate of QGY-7701 cells treated with GST-TAT-LPTS39 decreased to 62.7% (10 µg/mL), 53.7% (40 µg/mL), 38.1% (100 µg/mL), and 8.9% (200 µg/mL) (Figure 3B). However, GST-TAT-LPTS39 protein did not influence the growth of telomerase-negative osteosarcoma cell Saos-2 (Figure 3C). In conclusion, 10 µg/mL GST-TAT-LPTS39 protein treatment affected the growth of BEL-7404 and QGY-7701 cells, the growth inhibition of cells was more obvious with increasing protein concentration and 200 µg/mL almost completely inhibited the two types of cell growth (Figure 3D). These results indicated that GST-TAT-LPTS39 inhibited telomerase-positive tumour cell growth.
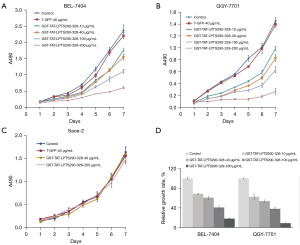
GST-TAT-LPTS39 induced cell senescence and apoptosis
To assess whether the reduction in GST-TAT-LPTS39-associated cell growth was induced by cell death, the cell death rate of BEL-7404 and Hela cells was analysed. BEL-7404 cells were treated with GST-TAT-LPTS39 protein at final concentrations of 40, 100 and 200 µg/mL. After 72 h treatment, a single-cell suspension was collected and prepared for FACS by PI staining. The results showed that the percentage of dead cells increased with the final concentration of 200 µg/mL GST-TAT-LPTS39 protein for 72 h, and the death rate reached 18.45% (Figure 4A). Hela cells were treated with GST-TAT-LPTS39 protein at final concentrations of 100 and 300 µg/mL for different times. Most of the Hela cells became round but remained adherent after 24 h treatment with the GST-TAT-LPTS39 protein. GST-TAT-LPTS39 protein at final concentrations of 100 and 300 µg/mL caused Hela cell death, with death rates of 14.02% and 17.21%, respectively, after 24 h (Figure 4B). The above results indicated that the GST-TAT-LPTS39 protein specifically induces cell death of BEL-7404 and Hela cells, and LPTS39, not the TAT peptide, is responsible for this effect. Moreover, the high concentration of TAT-LPTS39 protein could induce death in a short time.
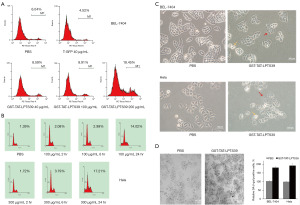
We also checked the morphology of BEL-7404 and Hela cells after treatment with PBS and GST-TAT-LPTS39 protein for 6 weeks. The cells were placed under an inverted microscope to observe the morphology. Compared with the PBS group, cells treated with the GST-TAT-LPTS39 protein showed significant changes in morphology, with cells becoming larger, flat and with blurred margins, which are all changes typically associated with cellular senescence (Figure 4C, red arrows). In addition, apoptotic cells were observed, which became small and round with fragmented nuclei (Figure 4C, yellow arrows). The β-galactosidase staining result showed that more than half of the cells became senescent at the sixth week. The percentage of SA-β-gal-positive cells treated with the GST-TAT-LPTS39 protein was almost double that of cells treated with PBS (Figure 4D). These data indicated that the GST-TAT-LPTS39 protein could induce BEL-7404 and Hela cells senescence.
GST-TAT-LPTS39 inhibited the growth of QGY-7701 human telomerase-positive cancer cells in a time-dependent manner
In this study, we found that GST-TAT-LPTS39 did not significantly induce apoptosis of QGY-7701 cells within 24 h (data not shown). Therefore, QGY-7701 cells were treated with GST-TAT-LPTS39 protein at final concentrations of 10 and 40 µg/mL every other day for a long time. After 1–2 weeks of treatment, when the protein concentration was 10 µg/mL, there was no significant change in mortality compared with the control group. However, the cell death rate of the 40 µg/mL treatment group reached 15.73%, approximately three times that of the control group, and the effect of low-concentration protein accumulation. Extending the cell treatment time to 6 weeks increased the death rate of cells. The death rate of 10 µg/mL GST-TAT-LPTS39 treatment reached 19.91%, while that of 40 µg/mL reached 34.75% (Figure 5). These results suggested that GST-TAT-LPTS39 inhibited the growth of the human telomerase-positive cancer cell line QGY-7701 in a time-dependent manner.
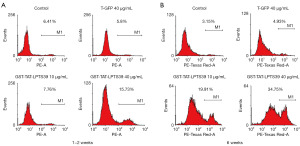
TAT-LPTS39 suppressed the growth of human telomerase-positive cancer cells
The N-terminal of the recombinant protein GST-TAT-LPTS39 contains a GST tag, approximately 26 KD, which may cause immunogenicity and interfere with the function of therapeutic peptide drugs. Therefore, we removed the GST tag and obtained the TAT-LPTS39 polypeptide without the GST tag. In our previous study we found liver cancer cell PLC-PRF-5 with short telomere was more sensitive to LPTS/PinX1 protein. We selected two types of telomerase-positive cancer cells Hela and PLC-PRF-5 to test the inhibitory effect of the TAT-LPTS39 polypeptide on cell growth. Cells were treated with TAT-LPTS39 polypeptide for 6–7 weeks to calculate the cell growth curve and Dt, respectively. The results showed that Hela and PLC-PRF-5 cells treated with TAT-LPTS39 grew more slowly than cells treated with PBS (Figure 6A). The Dt of Hela cells treated with 2 µM TAT-LPTS39 increased from 27.9 to 31.1 h, with an 11% rise compared to PBS-treated cells. The Dt of PLC-PRF-5 cells treated with 2 µM TAT-LPTS39 increased from 41.7 to 52.5 h, a 26% rise compared to PBS-treated cells (Figure 6B). Then we analysed the RTL by qPCR. Compared with PBS and T-GFP group, the RTLs of Hela and PLC-PRF-5 cells treated with the TAT-LPTS39 polypeptide were reduced (Figure 6C). These data indicated that TAT-LPTS39 could inhibit the growth and increase the Dt of telomerase-positive cancer cells by shortening telomere length.
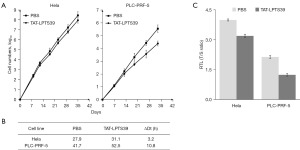
TAT-LPTS39 suppressed tumour growth in xenografted mice
We then examined the inhibitory effect of the TAT-LPTS39 polypeptide on tumour growth in vivo through the CDX model. In the BEL-7404 CDX models, a significant cessation of tumour growth was observed in the TAT-LPTS39 group compared to the PBS group, and the weight inhibition rates were 66.7%, but the T-GFP group had no obvious effect on tumour growth compared to the PBS group (Figure 7). These results indicated that the TAT-LPTS39 polypeptide could inhibit the growth of BEL-7404 cancer cell CDX.
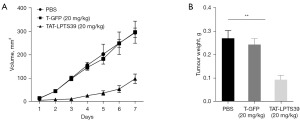
Discussion
Telomerase is an excellent target for cancer therapy, and candidate drugs targeting telomerase have market prospects. As an effective therapeutic method, peptide drugs are characterized by high activity, strong specificity, low toxicity, clear biological function and benefit for clinical application (27). Therefore, protein inhibitor therapy targeting telomerase may provide a new safe and effective cancer therapy approach. LPTS/PinX1 is the only human protein reported to inhibit telomerase activity, and overexpression of LPTS/PinX1 could inhibit the tumour cell growth in lung, breast, stomach, etc. (11,17,28). Early candidate peptides had high molecular weights and poor stability (26). Screening new LPTS functional peptides with good stability, high inhibitory activity and easy preparation will help to improve the feasibility of their application for the clinical treatment of cancer.
The 39 aa peptide of LPTS/PinX1, a 290-328 aa fragment, can bind to hTERT. Importantly, LPTS/PinX1 290-328 aa exhibited stronger telomerase inhibitory activity than the full-length LPTS/PinX1 protein (23). In this study, we successfully expressed and purified the recombinant protein GST-TAT-LPTS39 in vitro. The recombinant GST-TAT-LPTS39 could quickly enter the cell and be present for at least 24 h, indicating that it was relatively stable, which was an important property of protein drugs. Our previous study found that the LPTS/PinX1133-328 could inhibit the growth of eight types of liver cancer cell lines, indicating its potential as a broad-spectrum drug candidate for liver cancer treatment (26). Our novel GST-TAT-LPTS39 protein also could affect the growth of telomerase-positive liver cancer cells BEL-7404 and QGY-7701, but was ineffective to telomerase-negative cell Saos-2, indicating that the anticancer ability of GST-TAT-LPTS39 is specific by targeting telomerase. The telomerase inhibitory activity of GST-TAT-LPTS39 protein was detected in vitro, while the inhibition strengthened with increasing protein concentration. Therefore, GST-TAT-LPTS39 protein, based on the telomerase inhibitory activity, has good research and development prospects for cancer therapy.
Our further study revealed that the GST-TAT-LPTS39 protein could induce senescence of telomerase-positive cancer BEL-7404 and Hela cells. Some senescent cells could continue to divide and undergo severe telomere shortening, which eventually results in crisis, including chromosome fusion and cell death (29). The GST-TAT-LPTS39 protein had no obvious effect on the cell apoptosis of QGY-7701 liver cancer cells in a short period of time, and the death rate gradually increased with increasing time. After 6 weeks, even at a low concentration of 10 µg/mL, there was a strong cell death rate. This demonstrated that the effect of the GST-TAT-LPTS39 protein on liver cancer cell QGY-7701 was time dependent.
For the subsequent development of peptide drugs, we removed the GST tag from the protein GST-TAT-LPTS39 and obtained a highly pure TAT-LPTS39 polypeptide containing 53 aa. The TAT-LPTS39 polypeptide could inhibit the growth of telomerase-positive cancer cells in vitro and in vivo. Although the difference of Hela cell growth in division time was only 11%, we found the inhibitory effect of TAT-LPTS39 polypeptide on Hela cell growth gradually increased over time. Moreover, after treatment with TAT-LPTS39 polypeptide, the RTLs of telomerase-positive cancer cells were shortened, which further showed that the ability of TAT-LPTS39 to inhibit tumor cell growth was achieved by inhibiting telomerase activity and then shortening telomere length. Compared with our previously reported TAT-LPTS133-328 (TLC) recombinant protein, its size is only 1/4 of TLC and showed good ability to inhibit the growth of cancer cells (26). Therefore, TAT-LPTS39 has a better prospect in the development of protein drugs.
Although the TAT-LPTS39 polypeptide could effectively inhibit the growth of tumour cells, there is still a lot of work to be done to enter clinical anticancer drug development. Firstly, our study only showed that TAT-LPTS39 polypeptide inhibited the growth of liver cancer and cervical cancer cells, and subsequent broad-spectrum studies are necessary. Secondly, TAT-LPTS39 polypeptide worked slowly on inhibiting tumour cell growth. Therefore, combining TAT-LPTS39 polypeptide with other drugs can be considered to improving anticancer activity. The telomerase inhibitor BIBR1532 was used in combination with paclitaxel to inhibit the telomerase activity in breast cancer cells (30). However, telomerase inhibitors have not been achieved successful application in clinical trials as anticancer drugs due to their toxic and side effects in other cell types such as stem cells which also have telomerase activity (31). The development of anticancer drugs specific for telomerase with low toxicity is demanding.
Conclusions
We constructed the recombinant protein GST-TAT-LPTS39 by fusing the C-terminal 290-328 of LPTS and the cell-penetrating peptide TAT. Purified GST-TAT-LPTS39 could quickly enter cells and inhibit telomerase-positive cell growth by inducing cell crisis and death. Moreover, to reduce the effect of the GST tag, TAT-LPTS39 was prepared. The study found that TAT-LPTS39 still has a good ability to inhibit the growth of telomerase-positive cancer cells in vitro and in vivo, which laid a good foundation for subsequent anticancer drug development.
Acknowledgments
Funding: This work was supported by grants from
Footnote
Reporting Checklist: The authors have completed the ARRIVE and MDAR reporting checklists. Available at https://tcr.amegroups.com/article/view/10.21037/tcr-24-792/rc
Data Sharing Statement: Available at https://tcr.amegroups.com/article/view/10.21037/tcr-24-792/dss
Peer Review File: Available at https://tcr.amegroups.com/article/view/10.21037/tcr-24-792/prf
Conflicts of Interest: All authors have completed the ICMJE uniform disclosure form (available at https://tcr.amegroups.com/article/view/10.21037/tcr-24-792/coif). The authors have no conflicts of interest to declare.
Ethical Statement: The authors are accountable for all aspects of the work in ensuring that questions related to the accuracy or integrity of any part of the work are appropriately investigated and resolved.
Open Access Statement: This is an Open Access article distributed in accordance with the Creative Commons Attribution-NonCommercial-NoDerivs 4.0 International License (CC BY-NC-ND 4.0), which permits the non-commercial replication and distribution of the article with the strict proviso that no changes or edits are made and the original work is properly cited (including links to both the formal publication through the relevant DOI and the license). See: https://creativecommons.org/licenses/by-nc-nd/4.0/.
References
- de Lange T. How telomeres solve the end-protection problem. Science 2009;326:948-52. [Crossref] [PubMed]
- Shay JW. Role of Telomeres and Telomerase in Aging and Cancer. Cancer Discov 2016;6:584-93. [Crossref] [PubMed]
- Saretzki G. Role of Telomeres and Telomerase in Cancer and Aging. Int J Mol Sci 2023;24:9932. [Crossref] [PubMed]
- Maciejowski J, de Lange T. Telomeres in cancer: tumour suppression and genome instability. Nat Rev Mol Cell Biol 2017;18:175-86. [Crossref] [PubMed]
- Shay JW, Wright WE. Telomeres and telomerase: three decades of progress. Nat Rev Genet 2019;20:299-309. [Crossref] [PubMed]
- Liu M, Zhang Y, Jian Y, et al. The regulations of telomerase reverse transcriptase (TERT) in cancer. Cell Death Dis 2024;15:90. [Crossref] [PubMed]
- Tornesello ML, Cerasuolo A, Starita N, et al. Reactivation of telomerase reverse transcriptase expression in cancer: the role of TERT promoter mutations. Front Cell Dev Biol 2023;11:1286683. [Crossref] [PubMed]
- Lansdorp PM. Telomeres, Telomerase and Cancer. Arch Med Res 2022;53:741-6. [Crossref] [PubMed]
- Ali JH, Walter M. Combining old and new concepts in targeting telomerase for cancer therapy: transient, immediate, complete and combinatory attack (TICCA). Cancer Cell Int 2023;23:197. [Crossref] [PubMed]
- Liao C, Zhao M, Song H, et al. Identification of the gene for a novel liver-related putative tumor suppressor at a high-frequency loss of heterozygosity region of chromosome 8p23 in human hepatocellular carcinoma. Hepatology 2000;32:721-7. [Crossref] [PubMed]
- Yao Y, Lin M, Liu Z, et al. Hesperidin Inhibits Lung Cancer In Vitro and In Vivo Through PinX1. Front Pharmacol 2022;13:918665. [Crossref] [PubMed]
- Majercikova Z, Dibdiakova K, Gala M, et al. Different Approaches for the Profiling of Cancer Pathway-Related Genes in Glioblastoma Cells. Int J Mol Sci 2022;23:10883. [Crossref] [PubMed]
- Kondo T, Oue N, Mitani Y, et al. Loss of heterozygosity and histone hypoacetylation of the PINX1 gene are associated with reduced expression in gastric carcinoma. Oncogene 2005;24:157-64. [Crossref] [PubMed]
- Deng W, Jiao N, Li N, et al. Decreased expression of PinX1 protein predicts poor prognosis of colorectal cancer patients receiving 5-FU adjuvant chemotherapy. Biomed Pharmacother 2015;73:1-5. [Crossref] [PubMed]
- Wang S, Zhang H, Zhu J, et al. PinX1 Is a Potential Prognostic Factor for Non-Small-Cell Lung Cancer and Inhibits Cell Proliferation and Migration. Biomed Res Int 2017;2017:7956437. [Crossref] [PubMed]
- Wu G, Liu D, Jiang K, et al. PinX1, a novel target gene of p53, is suppressed by HPV16 E6 in cervical cancer cells. Biochim Biophys Acta 2014;1839:88-96. [Crossref] [PubMed]
- Shi R, Zhou JY, Zhou H, et al. The role of PinX1 in growth control of breast cancer cells and its potential molecular mechanism by mRNA and lncRNA expression profiles screening. Biomed Res Int 2014;2014:978984. [Crossref] [PubMed]
- Hou P, Li H, Yong H, et al. PinX1 represses renal cancer angiogenesis via the mir-125a-3p/VEGF signaling pathway. Angiogenesis 2019;22:507-19. [Crossref] [PubMed]
- Li HL, Song J, Yong HM, et al. PinX1: structure, regulation and its functions in cancer. Oncotarget 2016;7:66267-75. [Crossref] [PubMed]
- Zhou XZ, Lu KP. The Pin2/TRF1-interacting protein PinX1 is a potent telomerase inhibitor. Cell 2001;107:347-59. [Crossref] [PubMed]
- Liu JY, Qian D, He LR, et al. PinX1 suppresses bladder urothelial carcinoma cell proliferation via the inhibition of telomerase activity and p16/cyclin D1 pathway. Mol Cancer 2013;12:148. [Crossref] [PubMed]
- Zhou XZ, Huang P, Shi R, et al. The telomerase inhibitor PinX1 is a major haploinsufficient tumor suppressor essential for chromosome stability in mice. J Clin Invest 2011;121:1266-82. [Crossref] [PubMed]
- Chen G, Da L, Xu Y, et al. C-terminal amino acids 290-328 of LPTS/PinX1 confer telomerase inhibition. Biochem Biophys Res Commun 2010;398:683-9. [Crossref] [PubMed]
- Chen G, Da L, Wang H, et al. HIV-Tat-mediated delivery of an LPTS functional fragment inhibits telomerase activity and tumorigenicity of hepatoma cells. Gastroenterology 2011;140:332-43. [Crossref] [PubMed]
- Kim NW, Wu F. Advances in quantification and characterization of telomerase activity by the telomeric repeat amplification protocol (TRAP). Nucleic Acids Res 1997;25:2595-7. [Crossref] [PubMed]
- Chen G, Zhang X, He W, et al. A scalable and reproducible preparation for the antitumor protein TLC, a human-derived telomerase inhibitor. Protein Expr Purif 2021;187:105942. [Crossref] [PubMed]
- Sarfaraz M, Anjum F, Zahra D, et al. Recent Updates on Peptide Molecules in Drug and Vaccine Development. Curr Pharm Des 2023;29:1564-78. [Crossref] [PubMed]
- Wang HB, Wang XW, Zhou G, et al. PinX1 inhibits telomerase activity in gastric cancer cells through Mad1/c-Myc pathway. J Gastrointest Surg 2010;14:1227-34. [Crossref] [PubMed]
- Murnane JP. Telomere dysfunction and chromosome instability. Mutat Res 2012;730:28-36. [Crossref] [PubMed]
- Shi Y, Sun L, Chen G, et al. A combination of the telomerase inhibitor, BIBR1532, and paclitaxel synergistically inhibit cell proliferation in breast cancer cell lines. Target Oncol 2015;10:565-73. [Crossref] [PubMed]
- Chen X, Tang WJ, Shi JB, et al. Therapeutic strategies for targeting telomerase in cancer. Med Res Rev 2020;40:532-85. [Crossref] [PubMed]