The pan-cancer landscape of crosstalk between leukocyte transendothelial migration-related genes and tumor microenvironment relevant to prognosis and immunotherapy response
Highlight box
Key findings
• Leukocyte transendothelial migration-related genes (LTEMGs) are differentially expressed in various tumors and associated with prognosis and immunotherapy response. The LTEMGs score in pan-cancer is positively linked to multiple immune pathways, immune cell infiltration, and immune regulation genes. The high LTEMGs score correlates with poor immunotherapy responses, suggesting its potential as a predictive biomarker.
What is known and what is new?
• Leukocyte transendothelial migration is crucial for immune cell infiltration into tumor tissues, affecting tumor microenvironment. Dysregulation of leukocyte transendothelial migration can impact tumor progression, immune evasion, and angiogenesis, etc.
• This study provides a systematic analysis of LTEMGs expression and prognosis in pan-cancer, establishing a comprehensive LTEMGs score. The score is shown to be a significant marker for immune-related pathways and could predict immunotherapy outcomes. The study highlights potential therapeutic strategies, such as combining Kirsten rat sarcoma viral oncogene homolog (KRAS) inhibitors with LTEMGs-targeted therapies, to enhance immunotherapy effectiveness.
What is the implication, and what should change now?
• The LTEMGs score could serve as a biomarker for predicting immunotherapy efficacy, aiding in patient stratification and personalized treatments. Combining KRAS inhibitors with LTEMGs-targeted therapies may improve immunotherapy responses by mitigating immune evasion. Future research should validate the LTEMGs score through experimental methods and expand single-cell RNA sequencing to fully understand LTEMGs’ roles. Clinical trials combining KRAS inhibitors with LTEMGs-targeted therapies should be considered to enhance immunotherapy outcomes. Continuous refinement of the gene set variation analysis method is necessary to ensure robust results.
Introduction
Tumor development and metastasis involve complex interactions between cancer cells, stromal components, and the host immune system (1,2). Among these interactions, leukocytes play a vital role in shaping the tumor microenvironment. Leukocyte transendothelial migration refers to the process by which immune cells cross the endothelial barrier and infiltrate into the tumor tissue, exerting both pro- and anti-tumorigenic effects (3-5). Leukocyte subsets involved in leukocyte transendothelial migration can include neutrophils, monocytes, dendritic cells, and T lymphocytes. These immune cells are recruited from circulation and migrate through the endothelial cell layer via a multistep process involving rolling, adhesion, crawling, and transmigration. Each step is regulated by a series of adhesion molecules, chemokines, and signaling pathways (6,7).
Understanding the molecular and cellular mechanisms underlying leukocyte transendothelial migration in the tumor microenvironment is critical for unraveling its impact on tumor growth, angiogenesis, immune evasion, and therapeutic responses. Dysregulation of leukocyte infiltration can result in an immunosuppressive microenvironment conducive to tumor progression (8). Conversely, effective microenvironment remodeling and activation of anti-tumor immune cells can enhance the antitumor immune response (9,10).
In this work, we conducted a systematic analysis of leukocyte transendothelial migration-related genes (LTEMGs) in multiple cancer types. The tumor microenvironment, especially the tumor immune microenvironment (TIME), has essential influence on cancer progression (11). Thus, we further explored the association of LTEMGs score with TIME and immunotherapy response, providing the indication of score on the efficacy of immunotherapy. We present this article in accordance with the REMARK reporting checklist (available at https://tcr.amegroups.com/article/view/10.21037/tcr-24-556/rc).
Methods
Data collection
LTEMGs were obtained from the Kyoto Encyclopedia of Genes and Genomes (KEGG) database (https://www.kegg.jp/pathway/hsa04670). A total of 114 genes were enrolled. Bulk pan-cancer RNA transcriptome data and clinical information of The Cancer Genome Atlas (TCGA) and Genotype-Tissue Expression (GTEx) were obtained from the University of California, Santa Cruz Genomic Browser (UCSC) database (https://xenabrowser.net/datapages/). Infiltration level of most immune cells was downloaded from ImmuCellAI database (http://bioinfo.life.hust.edu.cn/ImmuCellAI#!/) and Tumor IMmune Estimation Resource, version 2 (TIMER2) database (http://timer.cistrome.org/). For immunotherapeutic efficacy analysis, the Bulk data of GSE135222 (non-small cell lung carcinoma) and GSE91061 (Melanoma) were downloaded from the Gene Expression Omnibus (GEO) database (https://www.ncbi.nlm.nih.gov/gds/?term=). Single-cell data of GSE115978 (SKCM_aPD1), GSE120575 (SKCM_aPD1aCTLA4), GSE123813 (BCC_aPD1), and GSE145281 (BLCA_aPDL1) were obtained from the Tumor Immune Single-Cell Hub (TISCH) database (tisch.comp-genomics.org/home/). The sample sizes of cancer and normal tissues in TCGA and GTEx databases and full names of cancer abbreviations are provided in the table available at https://cdn.amegroups.cn/static/public/TCR-24-556-1.xlsx of a previously published article (see https://www.researchsquare.com/article/rs-3340580/v1). The study was conducted in accordance with the Declaration of Helsinki (as revised in 2013).
Calculation of LTEMGs score and prognostic analysis
The LTEMGs score was quantified using the single-sample gene set enrichment analysis (ssGSEA) algorithm in R package “GSVA”. The ssGSEA algorithm allows for the estimation of gene set enrichment scores for individual samples, enabling the assessment of the activity levels of gene sets, such as LTEMGs, in a given dataset. To evaluate the prognostic significance of the LTEMGs score, we employed the “survival” and “survminer” packages for Kaplan-Meier survival analysis, which was used to estimate and visualize survival probabilities over time, providing a non-parametric approach to compare the survival experiences of different patient groups. Additionally, univariate Cox regression analysis (uniCox) was conducted to assess the relationship between LTEMGs score and patient survival outcomes, offering a method to determine the impact of LTEMGs on the hazard rate for survival. To enhance the interpretability of the Kaplan-Meier analysis, the best cut-off value for stratifying patients based on their LTEMGs score was calculated using the “survminer” package. This optimal cut-off value then applied to divide patients into high and low LTEMGs score groups, allowing for a clearer comparison of survival differences between these groups.
Enrichment analysis
To investigate the impact of LTEMGs on different types of cancer, we conducted gene set variation analysis (GSVA) enrichment analysis using the R package “GSVA”. GSVA is a non-parametric and unsupervised method that estimates variation of gene set enrichment through the samples of an expression dataset. This method allows us to evaluate the relative enrichment of predefined gene sets, across a sample population without relying on a binary classification of samples. Our aim was to assess the relationship between LTEMGs and the 50 HALLMARK pathways from the Molecular Signatures Database (MsigDB) (http://software.broadinstitute.org/gsea/msigdb/index.jsp). The HALLMARK pathways represent coherent gene sets that summarize and define specific biological states or processes. By applying GSVA, we could quantify the enrichment scores of these pathways in different cancer types and examine how LTEMGs influence various biological processes and cancer hallmarks.
Tumor microenvironment
To explore the tumor microenvironment signature, we utilized the “ESTIMATE” package. The ESTIMATE algorithm (Estimation of STromal and Immune cells in MAlignant Tumor tissues using Expression data) allows for the inference of tumor purity, stromal score, ESTIMATE score, and immune score from gene expression data. Tumor purity indicates the proportion of cancer cells in the tumor tissue, while the stromal and immune scores reflect the presence of stromal and immune cells, respectively. The ESTIMATE score combines these two scores to provide an overall assessment of the tumor microenvironment. We calculated these scores for each cancer type and performed correlation analysis to examine the relationship between the LTEMGs score and these tumor microenvironment components. Additionally, we applied a methodology curated by Zeng (12) to investigate the correlation between the LTEMGs score and other tumor microenvironment-related biological processes. This comprehensive analysis allows us to gain insights into how LTEMGs influence the composition and behavior of the tumor microenvironment across different cancers.
Statistics analysis
R software was used for all statistical analysis (https://www.r-project.org/; version:4.2.2). Correlation analysis used Pearson correlation coefficients, with a significance level of P<0.05 (two-tailed). All histograms’ statistics were calculated using the R packages “ggplot2” and “ggpubr”. A P value of *P<0.05, **P<0.01, ***P<0.001, and ****P<0.0001 was considered statistically significant.
Results
The messenger RNA (mRNA) expression and prognostic analysis of LTEMGs
We firstly assessed the aberrant expression of 114 LTEMGs in pan-cancer. We found that LTEMGs were mainly up-regulated in several tumor types, especially in acute myeloid leukemia (LAML) and pancreatic adenocarcinoma (PAAD) (Figure 1A). Figure 1B displays the correlation between LTEMGs. The protein-protein interaction network was showed in Figure 1C. We also explored the prognostic role of LTEMGs. Results suggested that most LTEMGs were risk factors in low-grade glioma (LGG), PAAD, uveal melanoma (UVM), and LAML based on uniCox (Figure 2A) and Kaplan-Meier (Figure 2B) results.
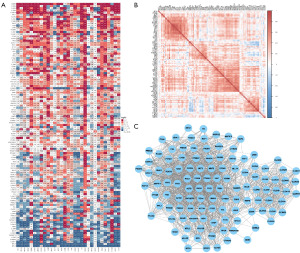
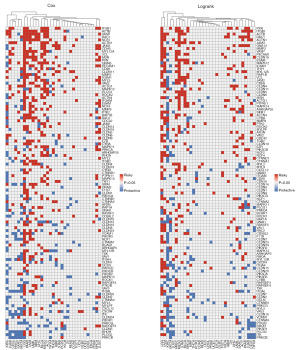
The calculation and analysis of LTEMGs score
To better understand the function of LTEMGs, we constructed and calculated LTEMGs score in pan-cancer. Results indicated that the score was highest in kidney renal clear cell carcinoma (KIRC) and lowest in UVM (Figure 3A). In addition, The score was higher in tumor tissues compared to the adjacent normal tissues in thyroid carcinoma (THCA), stomach adenocarcinoma (STAD), and esophageal carcinoma (ESCA), and lower in breast invasive carcinoma (BRCA), prostate adenocarcinoma (PRAD), bladder urothelial carcinoma (BLCA), colon adenocarcinoma (COAD), lung squamous cell carcinoma (LUSC), rectum adenocarcinoma (READ), kidney renal papillary cell carcinoma (KIRP), lung adenocarcinoma (LUAD), liver hepatocellular carcinoma (LIHC), and kidney chromophobe (KICH) (Figure 3B).
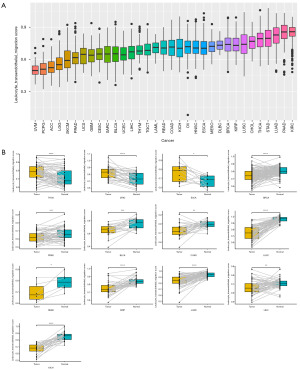
Further, we conducted the uniCox analysis to examine the LTEMGs score. (I) Regarding the overall survival (OS), the score proved to be a risk factor in LGG, UVM, LUSC, testicular germ cell tumor (TGCT), and STAD, but a protective factor in KIRC, skin cutaneous melanoma (SKCM), and adrenocortical carcinoma (ACC) (Figure 4A). (II) Concerning the disease-specific survival (DSS), the score was identified as a risk factor in LGG, UVM, and TGCT, while it acted as a protective factor in KIRC, SKCM, THCA, and ACC (Figure 4B). (III) In relation to the disease-free interval (DFI), the score showed a protective effect in LIHC (Figure 4C). (IV) Regarding the progression-free interval (PFI), the score was found to be a risk factor in LGG, glioblastoma multiforme (GBM), and UVM, but a protective factor in KIRC, head and neck squamous cell carcinoma (HNSC), LIHC, cholangiocarcinoma (CHOL), and ACC (Figure 4D).
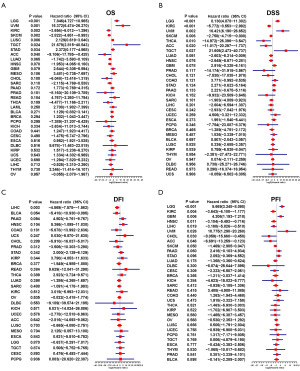
GSVA of LTEMGs score
To understand the potential function that LTEMGs may involve, we also calculated the scores of 50 HALLMARK pathways. The correlation between these scores and LTEMGs score in pan-cancer was analyzed, correlation coefficients, P values, and confidence intervals (CIs) were shown in the table available at https://cdn.amegroups.cn/static/public/TCR-24-556-1.xlsx. Results indicated that LTEMGs score was significantly positively correlated with Kirsten rat sarcoma viral oncogene homolog (KRAS) signaling up, interleukin-2 (IL-2) signal transducer and activator of transcription 5 (STAT5) signaling, apoptosis, and inflammatory response, etc. Conversely, the LTEMGs score was negatively correlated with DNA repair, MYC targets V2, E2F targets, and oxidative phosphorylation, etc. (Figure 5).
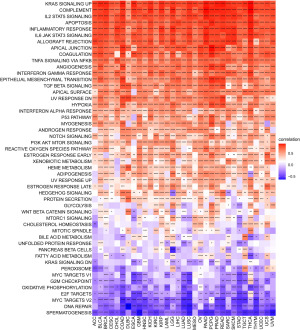
Relationship between LTEMGs score and the TIME
Following that, we examined the correlation between TIME and LTEMGs score (Figure 6A). The findings indicated a positive association between LTEMGs score and both immune and stromal scores. Moreover, we examined the connection between LTEMGs score and tumor microenvironment-related pathways. The results indicated a strong relationship of the score with immune-related pathways including immune checkpoint, cytotoxic T lymphocytes (CD8 T) effector, and antigen processing machinery, etc. (Figure 6B).
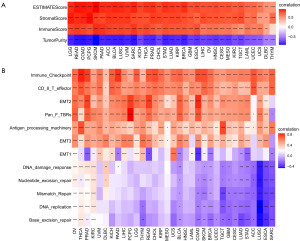
Immune infiltrating analysis
Our findings suggested that LTEMGs score had close relationship with TIME. Thus, we further assessed the connection between the score and infiltration level of most immune cells. Results based on data from ImmuCellAI database suggested that LTEMGs score was positively correlated with most immune cells, such as macrophage and induced regulatory T (iTreg) cells (Figure 7A). Results from TIMER2 data also come to same conclusion that patients with high LTEMGs score had more immune cells infiltration level (Figure 7B). Additionally, we further analyzed and found that LTEMGs score was highly associated with immune regulation-related genes (Figure 8A), chemokines (Figure 8B), and chemokine receptors (Figure 8C).
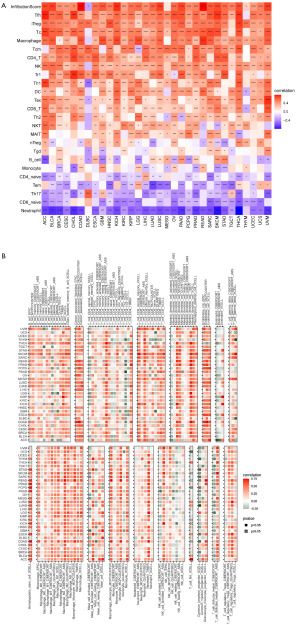
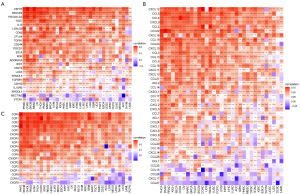
The prediction of immunotherapy efficacy
We next explored the predictive effect of LTEMGs score on immunotherapy efficacy. Multiple Kaplan-Meier survival analyses were performed using “survival” and “survimier” packages to determine the optimal classification criteria for the high- and low-risk group based on the smallest P value. We found that cancer patients undergoing immune checkpoint inhibitors treatment with higher LTEMGs score have worse OS in immunotherapy cohort, including GSE135222 (Figure 9A,9B) and GSE91061 (Figure 9C,9D) cohorts. These results indicated that patients with high LTEMGs score may be resistant to immunotherapy.
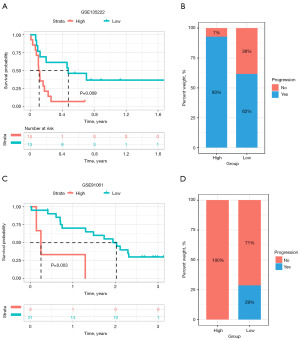
Single-cell analysis of LTEMGs
We also evaluated the function of LTEMGs in four immunotherapy-related single-cell RNA (scRNA) datasets. The expression of LTEMGs in each cell type was displayed (Figure 10A). For example, C-X-C chemokine receptor type 4 (CXCR4) was generally expressed in most cell types in all datasets, indicating an essential role in tumor microenvironment. In addition, the LTEMGs score were relative higher in endothelial and monocyte (Figure 10B). We compared the score between the immunotherapy response group and non-response (NR) group, and found that the levels of the score were higher in the NR group in all datasets (Figure 11A-11D). These findings are consistent with previous results, indicating that patients with higher LTEMGs score may be less responsive to immunotherapy.
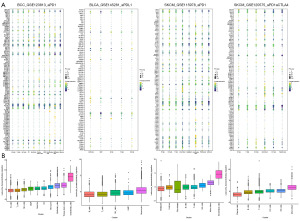
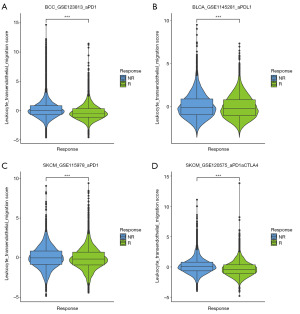
Discussion
Leukocyte transendothelial migration plays a pivotal role in the tumor microenvironment by facilitating the infiltration of immune cells into tumor tissues (13). Understanding the mechanisms and implications of LTEMGs in cancer has garnered significant attention in recent years. Numerous studies have elucidated the intricate molecular mechanisms underlying LTEMGs within the tumor microenvironment. These investigations have highlighted the involvement of various adhesion molecules, chemokines, and signaling pathways in regulating the distinct steps of leukocyte transendothelial migration, including rolling, adhesion, crawling, and transmigration. The dysregulation of these processes can significantly impact tumor progression, immune evasion, and angiogenesis (14-16).
In our work, we systematically analyzed the expression and prognosis of LTEMGs in pan-cancer. These genes were generally differentially expressed in most tumor types. To better understand the function of LTEMGs, we constructed and calculated LTEMGs score. We proved that the score was positively associated with multiple immune-related pathways in pan-cancer, such as KRAS signaling up, IL-2 STAT5 signaling, apoptosis and inflammatory response, etc. A study has shown that the activation of the KRAS signaling pathway can regulate leukocyte transendothelial migration by promoting the recruitment of immunosuppressive cells, such as regulatory T cells and myeloid-derived suppressor cells, thereby enhancing the tumor’s immune evasion capability and affecting the efficacy of immunotherapy. As an immunotherapy marker, high levels of KRAS expression may be associated with poor responses to immunotherapy, which is consistent with our findings (17). This suggests that the combination of KRAS inhibitors with LTEMGs-targeted therapies holds promise for improving the effectiveness of tumor immunotherapy. Similarly, IL-2 is a cytokine secreted by activated T cells, primarily functioning to promote the growth, differentiation, and survival of T cells. IL-2 binds to its receptor to activate JAK kinases, which in turn activate STAT5. Phosphorylated STAT5 can regulate the expression of multiple genes, promoting the proliferation and survival of T cells. Additionally, the IL-2 STAT5 signaling pathway can enhance the proliferation and persistence of chimeric antigen receptor T (CAR-T) cells, regulate their migration in vivo, and improve their antitumor effects. However, its supportive role for regulatory T cells may suppress antitumor immune responses. Based on the results of this study, further analysis of IL-2 STAT5 signaling and LTEMGs may lead to the development of new therapeutic targets, thereby improving the efficacy of therapies such as CAR-T cells therapy (18,19).
Accumulating evidence suggests that aberrant leukocyte transendothelial migration contributes to tumor progression and metastasis. Enhanced infiltration of immune cells, such as neutrophils, monocytes, dendritic cells, and T lymphocytes, into the tumor microenvironment has been associated with increased angiogenesis, immunosuppression, and promotion of tumor cell survival and proliferation. Conversely, impaired or limited leukocyte transendothelial migration can lead to compromised antitumor immune responses (20-22). Our results indicated that LTEMGs score was closely associated with tumor microenvironment. The score was positively correlated with most immune cells, such as macrophage and iTreg cells. Additionally, we further analyzed and found that the score was highly associated with immune regulation-related genes, chemokines, and chemokine receptors.
Leukocyte transendothelial migration is closely intertwined with immunotherapy responses in cancer patients (23,24). We observed that the extent of leukocyte infiltration, as measured by LTEMGs score, can serve as a predictive biomarker for immunotherapy efficacy. Patients with higher levels of the score typically demonstrate more unfavorable responses to immunotherapy.
In our study, there are some limitations that need to be noted. First, our analysis relied primarily on Bulk RNA-seq data, an approach that aggregates transcripts from multiple cell types and may mask cell-specific effects. Although we also used scRNA sequencing (scRNA-seq) data for partial validation, due to the limited coverage and depth of single-cell data, it is not yet possible to fully reveal the specific expression and function of LTEMGs in different cell types. Second, our study was mainly based on bioinformatics analysis and data integration from public databases, and lacked experimental validation to support our predicted results. Future studies should be combined with experimental methods, such as flow cytometry, immunohistochemistry, and functional tests, to further verify the specific mechanism of LTEMGs in different cancers. Finally, we used the GSVA method to calculate LTEMGs scores in our study, and although this method has high sensitivity and specificity in assessing gene set enrichment, its results may be influenced by the selected gene set and sample quality. Therefore, the results may vary from study to study.
To summarize, our study demonstrates a correlation between increased LTEMGs score and various immune-related traits of the TIME. This suggests that the score has the potential to serve as a biomarker for immunotherapy in patients with tumors.
Conclusions
LTEMGs play a crucial role in the tumor progression and tumor microenvironment, affecting immune cell infiltration and immunotherapy responsiveness. Our study found that the LTEMGs score is positively associated with multiple immune-related pathways and immune cells. This score can serve as a predictive biomarker for immunotherapy efficacy. Despite some limitations, our findings suggest that targeting LTEMGs, along with pathways like KRAS and IL-2 STAT5, could improve cancer immunotherapy outcomes. Further experimental validation is needed to confirm these results.
Acknowledgments
All authors acknowledged TCGA, GTEx, UCSC, ImmuCellAI, TIMER2, GEO, and TISCH2 databases for providing their platform and contributors for uploading their meaningful datasets.
Funding: This research was funded by grants from
Footnote
Reporting Checklist: The authors have completed the REMARK reporting checklist. Available at https://tcr.amegroups.com/article/view/10.21037/tcr-24-556/rc
Peer Review File: Available at https://tcr.amegroups.com/article/view/10.21037/tcr-24-556/prf
Conflicts of Interest: All authors have completed the ICMJE uniform disclosure form (available at https://tcr.amegroups.com/article/view/10.21037/tcr-24-556/coif). The authors have no conflicts of interest to declare.
Ethical Statement: The authors are accountable for all aspects of the work in ensuring that questions related to the accuracy or integrity of any part of the work are appropriately investigated and resolved. The study was conducted in accordance with the Declaration of Helsinki (as revised in 2013).
Open Access Statement: This is an Open Access article distributed in accordance with the Creative Commons Attribution-NonCommercial-NoDerivs 4.0 International License (CC BY-NC-ND 4.0), which permits the non-commercial replication and distribution of the article with the strict proviso that no changes or edits are made and the original work is properly cited (including links to both the formal publication through the relevant DOI and the license). See: https://creativecommons.org/licenses/by-nc-nd/4.0/.
References
- Mo J, Anastasaki C, Chen Z, et al. Humanized neurofibroma model from induced pluripotent stem cells delineates tumor pathogenesis and developmental origins. J Clin Invest 2021;131:e139807. [Crossref] [PubMed]
- Liao CP, Booker RC, Brosseau JP, et al. Contributions of inflammation and tumor microenvironment to neurofibroma tumorigenesis. J Clin Invest 2018;128:2848-61. [Crossref] [PubMed]
- Ondruššek R, Kvokačková B, Kryštofová K, et al. Prognostic value and multifaceted roles of tetraspanin CD9 in cancer. Front Oncol 2023;13:1140738. [Crossref] [PubMed]
- Haydinger CD, Ashander LM, Tan ACR, et al. Intercellular Adhesion Molecule 1: More than a Leukocyte Adhesion Molecule. Biology (Basel) 2023;12:743. [Crossref] [PubMed]
- Pleskova SN, Bezrukov NA, Gorshkova EN, et al. Exploring the Process of Neutrophil Transendothelial Migration Using Scanning Ion-Conductance Microscopy. Cells 2023;12:1806. [Crossref] [PubMed]
- Zegeye MM, Matic L, Lengquist M, et al. Interleukin-6 trans-signaling induced laminin switch contributes to reduced trans-endothelial migration of granulocytic cells. Atherosclerosis 2023;371:41-53. [Crossref] [PubMed]
- Zou Z, Fang J, Ma W, et al. Porphyromonas gingivalis Gingipains Destroy the Vascular Barrier and Reduce CD99 and CD99L2 Expression To Regulate Transendothelial Migration. Microbiol Spectr 2023;11:e0476922. [Crossref] [PubMed]
- Piao W, Li L, Saxena V, et al. PD-L1 signaling selectively regulates T cell lymphatic transendothelial migration. Nat Commun 2022;13:2176. [Crossref] [PubMed]
- van Steen ACI, Kempers L, Schoppmeyer R, et al. Transendothelial migration induces differential migration dynamics of leukocytes in tissue matrix. J Cell Sci 2021;134:jcs258690. [Crossref] [PubMed]
- Dituri F, Mancarella S, Serino G, et al. Direct and Indirect Effect of TGFβ on Treg Transendothelial Recruitment in HCC Tissue Microenvironment. Int J Mol Sci 2021;22:11765. [Crossref] [PubMed]
- Mittal S, Brown NJ, Holen I. The breast tumor microenvironment: role in cancer development, progression and response to therapy. Expert Rev Mol Diagn 2018;18:227-43. [Crossref] [PubMed]
- Zeng D, Li M, Zhou R, et al. Tumor Microenvironment Characterization in Gastric Cancer Identifies Prognostic and Immunotherapeutically Relevant Gene Signatures. Cancer Immunol Res 2019;7:737-50. [Crossref] [PubMed]
- Mannion AJ, Odell AF, Taylor A, et al. Tumour cell CD99 regulates transendothelial migration via CDC42 and actin remodelling. J Cell Sci 2021;134:jcs240135. [Crossref] [PubMed]
- Roncato F, Regev O, Yadav SK, et al. Microtubule destabilization is a critical checkpoint of chemotaxis and transendothelial migration in melanoma cells but not in T cells. Cell Adh Migr 2021;15:166-79. [Crossref] [PubMed]
- Akeus P, Szeponik L, Langenes V, et al. Regulatory T cells reduce endothelial neutral sphingomyelinase 2 to prevent T-cell migration into tumors. Eur J Immunol 2021;51:2317-29. [Crossref] [PubMed]
- de Haan L, Suijker J, van Roey R, et al. A Microfluidic 3D Endothelium-on-a-Chip Model to Study Transendothelial Migration of T Cells in Health and Disease. Int J Mol Sci 2021;22:8234. [Crossref] [PubMed]
- Xu M, Zhao X, Wen T, et al. Unveiling the role of KRAS in tumor immune microenvironment. Biomed Pharmacother 2024;171:116058. [Crossref] [PubMed]
- Lutz V, Hellmund VM, Picard FSR, et al. IL18 Receptor Signaling Regulates Tumor-Reactive CD8+ T-cell Exhaustion via Activation of the IL2/STAT5/mTOR Pathway in a Pancreatic Cancer Model. Cancer Immunol Res 2023;11:421-34. [Crossref] [PubMed]
- Zhou L, Velegraki M, Wang Y, et al. Spatial and functional targeting of intratumoral Tregs reverses CD8+ T cell exhaustion and promotes cancer immunotherapy. J Clin Invest 2024;134:e180080. [Crossref] [PubMed]
- Piao W, Xiong Y, Li L, et al. Regulatory T Cells Condition Lymphatic Endothelia for Enhanced Transendothelial Migration. Cell Rep 2020;30:1052-1062.e5. [Crossref] [PubMed]
- Kwak SY, Park S, Kim H, et al. Atorvastatin Inhibits Endothelial PAI-1-Mediated Monocyte Migration and Alleviates Radiation-Induced Enteropathy. Int J Mol Sci 2021;22:1828. [Crossref] [PubMed]
- Zhao Y, Ting KK, Coleman P, et al. The Tumour Vasculature as a Target to Modulate Leucocyte Trafficking. Cancers (Basel) 2021;13:1724. [Crossref] [PubMed]
- Roh SE, Jeong Y, Kang MH, et al. Junctional adhesion molecules mediate transendothelial migration of dendritic cell vaccine in cancer immunotherapy. Cancer Lett 2018;434:196-205. [Crossref] [PubMed]
- Ding N, Zou Z, Sha H, et al. iRGD synergizes with PD-1 knockout immunotherapy by enhancing lymphocyte infiltration in gastric cancer. Nat Commun 2019;10:1336. [Crossref] [PubMed]