Exploring the key pathogenic mechanisms and potential intervention targets for Sophorae Flavescentis radix in managing bone metastasis of lung cancer based on network pharmacology and molecular docking techniques
Highlight box
Key findings
• MMP1 was identified as a significant differentially expressed gene that is inversely correlated with survival outcomes in bone metastasis of lung cancer.
• Molecular docking studies confirmed a strong binding affinity between MMP1 and luteolin.
• Sophorae Flavescentis radix may exert therapeutic effects on lung cancer bone metastasis by inhibiting MMP1 expression and modulating the abnormal activation of the Wingless/Integrated (Wnt) signaling pathway.
What is known, and what is new?
• The use of traditional Chinese medicine (TCM) in the treatment of cancer has been recognized, but the specific mechanisms and effective compounds often remain unidentified.
• This study revealed the active compounds of Sophorae Flavescentis radix and their target genes and highlighted that MMP1 could serve as a predictive biomarker and therapeutic target in lung cancer bone metastasis. Our findings showed the molecular basis for the efficacy of Sophorae Flavescentis radix in modulating key pathways such as the Wnt signaling pathway.
What is the implication, and what should change now?
• This study established that Sophorae Flavescentis radix is a viable option that can be incorporated as a complement to the therapeutic regimens for the treatment of lung cancer bone metastasis, as it appears to modulate MMP1 and its associated pathways.
• However, further clinical studies need to be conducted to validate the therapeutic potential of Sophorae Flavescentis radix and its components with a focus on creating targeted therapies that could improve the survival outcomes and quality of life of patients with lung cancer bone metastasis.
Introduction
Lung cancer is characterized by its insidious onset, rapid progression, and poor prognosis. The average five-year survival rate of lung cancer patients is nearly 16% in the United States, and <10% in England; thus, it is one of the deadliest malignancies worldwide (1,2). In China, lung cancer is the most common cancer (21.9%) and the leading cause of cancer-related death (26.4%) among male cancer patients, while it is the second most common cancer (13.3%) (following breast cancer) and the leading cause of cancer-related death (11.1%) among female cancer patients (3). Thus, it negatively affects Chinese society.
Lung cancer frequently metastasizes to distant sites such as the brain, bones, and lymph nodes (4). The bones are a common site of distant metastasis (4). Bone metastasis, which often affects weight-bearing bones like the axial skeleton, is a primary cause of pain in advanced lung cancer. If it spreads to the bones, patients only live for 6–10 months, and even after treatment only 40–50% of patients survive (5). Currently, there is no curative treatment for bone metastasis in lung cancer, and research primarily focuses on the clinical efficacy of chemotherapy or targeted therapies to control the disease and alleviate symptoms (6). Traditional Chinese medicine (TCM) is used broadly in the treatment of cancer, as it can enhance drug sensitivity, regulate immune function, improve the efficacy of chemotherapy, and improve patient prognosis (7-10). The advantages of TCM are mainly reflected in its multi-target, multi-pathway actions, broad biological activity, and low toxicity, making it an alternative therapy for various cancers, including lung cancer.
Sophorae Flavescentis radix is a bitter, cold herb that was first recorded in the Shennong Ben Cao Jing. This herb has a clearing heat, drying dampness, kills insects, and encourages diuresis. The main chemical components of Sophorae Flavescentis radix are alkaloids, flavonoids, phenylpropanoids, terpenes, steroids, organic acids, and volatile oils. Among these, matrine and oxymatrine are the two characteristic components, accounting for 3% and 1.5% of Sophorae Flavescentis radix, respectively. These components have been shown to exert anti-inflammatory and anti-tumor pharmacological effects, demonstrating significant anti-tumor activity against many cancers (11). Previous research on the anti-lung cancer effects of Sophorae Flavescentis radix has mostly explored the mechanisms of specific active ingredients acting on particular targets or pathways and has not examined the overall mechanism of action (12). Thus, using network pharmacology, this study explored the effects of Sophorae Flavescentis radix on lung cancer bone metastasis from the perspective of the holistic theory of TCM and the modernization of TCM research. Additionally, it employed bioinformatics methods to conduct a preliminary investigation of the network of action mechanisms, and to provide a reference for the development of new anti-lung cancer bone metastasis drugs and clinical medication based on Sophorae Flavescentis radix. We present this article in accordance with the STREGA reporting checklist (available at https://tcr.amegroups.com/article/view/10.21037/tcr-24-1947/rc).
Methods
Active-part finding and focus factors (genetic)
TCM Systems Pharmacology (TCMSP) (https://www.tcmsp-e.com/#/home) was used to screen the active components of the Sophorae Flavescentis radix, including the drug-likeness property (DLP) ≥0.18 and oral bioavailability (OB) ≥30%. The predicted targets of the compounds were screened from the DrugBank (https://www.drugbank.ca/) database. Additionally, UniProt (https://www.uniprot.org) was used to compared the target information.
Screening of differentially expressed genes (DEGs)
The DEGs of the primary and bone metastatic lung cancer tissue samples were obtained from the GSE175601 dataset in the Gene Expression Omnibus (GEO) database (https://www.ncbi.nlm.nih.gov/geo/). The data from GSE175601 was processed and analyzed for differential expression using CHDTEPDB (13). The target genes of Sophorae Flavescentis radix were intersected with the DEGs from the GSE175601 dataset to identify the final candidate target genes. The study was conducted in accordance with the Declaration of Helsinki (as revised in 2013).
Data examination using The Cancer Genome Atlas (TCGA) data
In the TCGA database (https://www.cancer.gov/ccg/research/genome-sequencing/tcga), the expression of key genes in lung cancer tumor tissues was explored, and their correlation with the prognosis of lung cancer patients was analyzed.
Protein-protein interaction network analysis
Various tools, such as the GeneMANIA (http://www.genemania.org) database, and Search Tool for Recurring Instances of Neighbouring Genes (STRING) (https://string-db.org/) online database were used to study the MMP1 interaction.
Docking of molecules
We sourced the 3D structures of the active chemicals from Pubchem (https://pubchem.ncbi.nlm.nih.gov/). The key targets’ receptor proteins were obtained using the Protein Data Bank (PDB) database (http://www.rcsb.org/pdb/home/home.do). For the molecular docking, the receptor proteins of the important targets and the 3D structures of the active ingredients were submitted to the DockThor (https://dockthor.lncc.br/v2/) online analysis platform. According to Dockthor calculations, the lower the binding energy required for the ligand to combine with the receptor, the more stable the binding and the better the effect. Finally, the CB-DOCK2 (https://cadd.labshare.cn/cb-dock2/) database was used to visualize the results.
Gene Set Enrichment Analysis (GSEA)
A functional analysis was conducted by uploading the DEGs to Metascape for investigation. This tool helped us to determine whether entire gene sets showed significant increases or decreases in biological processes. The identification of common signaling pathways and regulatory network modules extends understanding of the underlying mechanisms of biological differences between different samples.
Statistical analysis
R software (version 3.6.3) was used for the statistical analysis. The MMP1 mechanism was studied by a GSEA. The Kaplan-Meier method and log-rank test were used to examine patient survival and conduct significance testing, respectively. A P value or log-rank P value of <0.05 was considered statistically significant.
Results
Dynamic Sophorae Flavescentis radix’ genetic factors and parts
In the TCMSP database, 28 active compounds were identified from Sophorae Flavescentis radix as per the DLP and OB criteria (Table 1). Additionally, the target genes for these active compounds were screened from the DrugBank database. Among these compounds, (2R)-5,7-dihydroxy-2-(4-hydroxyphenyl)chroman-4-one, 5,7-dihydroxy-2-(3-hydroxy-4-methoxyphenyl)chroman-4-one, and matrine each had 10 target genes; inermine had 17 target genes; 8-isopentenyl-kaempferol had 29 target genes; sophocarpine and sophoridine each had two target genes; inermin had 18 target genes; wighteone had 19 target genes; formononetin had 39 target genes; cis-Dihydroquercetin had six target genes; (2R)-7-hydroxy-2-(4-hydroxyphenyl)chroman-4-one had 15 target genes; and luteolin had 50 target genes (Table S1).
Table 1
ID | Compound | OB (%) | DLP |
---|---|---|---|
MOL001040 | (2R)-5,7-dihydroxy-2-(4-hydroxyphenyl)chroman-4-one | 42.36332114 | 0.21141 |
MOL001484 | Inermine | 75.18306038 | 0.53754 |
MOL003542 | 8-Isopentenyl-kaempferol | 38.04433524 | 0.3948 |
MOL003627 | Sophocarpine | 64.26433236 | 0.25078 |
MOL003648 | Inermin | 65.83093145 | 0.53754 |
MOL003673 | Wighteone | 42.79667217 | 0.3622 |
MOL003676 | Sophoramine | 42.16188312 | 0.25182 |
MOL003680 | Sophoridine | 60.07032754 | 0.24941 |
MOL000392 | Formononetin | 69.67388061 | 0.21202 |
MOL004580 | cis-Dihydroquercetin | 66.43699795 | 0.27344 |
MOL004941 | (2R)-7-hydroxy-2-(4-hydroxyphenyl)chroman-4-one | 71.12298901 | 0.18303 |
MOL005100 | 5,7-dihydroxy-2-(3-hydroxy-4-methoxyphenyl)chroman-4-one | 47.73643694 | 0.27226 |
MOL005944 | Matrine | 63.77493138 | 0.24931 |
MOL000006 | Luteolin | 36.16262934 | 0.24552 |
MOL006561 | (+)-14alpha-hydroxymatrine | 35.72646117 | 0.28667 |
MOL006562 | (+)-7,11-dehydromatrine,(leontalbinine) | 62.08261476 | 0.2502 |
MOL006563 | (+)-9alpha-hydroxymatrine | 32.04497964 | 0.28641 |
MOL006564 | (+)-allomatrine | 58.87487526 | 0.24941 |
MOL006565 | AIDS211310 | 68.67679194 | 0.24936 |
MOL006566 | (+)-lehmannine | 58.33510716 | 0.25065 |
MOL006568 | isosophocarpine | 61.57153772 | 0.25081 |
MOL006569 | (–)-14beta-hydroxymatrine | 37.2580396 | 0.28667 |
MOL006570 | (–)-9alpha-hydroxysophoramine | 35.22654095 | 0.2882 |
MOL006571 | Anagyrine | 62.01260851 | 0.24338 |
MOL006572 | 1,4-diazaindan-type,alkaloid,flavascensine | 34.6417641 | 0.24095 |
MOL006573 | 13,14-dehydrosophoridine | 65.34313997 | 0.25078 |
MOL006582 | 5α,9α-dihydroxymatrine | 40.93360553 | 0.32076 |
MOL006583 | 7,11-dehydromatrine | 44.42628517 | 0.2503 |
OB, oral bioavailability; DLP, drug-likeness property.
DEG screening
The DEGs were obtained from the GSE175601 dataset in the GEO database as per the set criteria, and visualized in volcano plots, principal component analysis (PCA) plots, sample normalization box plots, and heatmaps (Figure 1A-1D). The (genetic) factors for the responsible constituents in Sophorae Flavescentis radix were intersected with the DEGs from the GSE175601 dataset, and a Venn diagram was generated, and the following five candidate target genes were identified: F10, JUN, AKR1B1, MMP1, and CCND1 (Figure 1E).
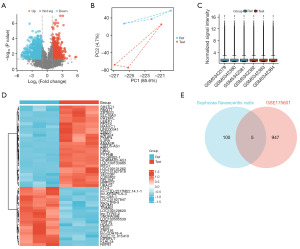
Expression of DEG in lung cancer tissues in the TCGA database
The five target genes (i.e., F10, JUN, AKR1B1, MMP1, and CCND1) were analyzed in lung cancer tumor tissues using TCGA database. In both the non-paired and paired lung cancer tumor tissues, MMP1 expression was significantly upregulated, while F10 and JUN expression was significantly downregulated (Figure 2A,2B).
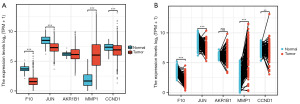
Correlation between the expression of five DEGs and the prognosis of lung cancer patients in the TCGA database
The prognostic value of the expression of the five DEGs was evaluated in lung cancer patients using TCGA data. The areas under the curve for F10, JUN, and MMP1 were all >0.7, indicating good predictive and discriminative value for these genes. Further, the low expression of AKR1B1, MMP1, and CCND1 indicated good overall survival (OS) in lung cancer patients (Figure 3A,3B). Based on these findings and the expression patterns in lung cancer tissues, MMP1 was identified as the key gene of interest.
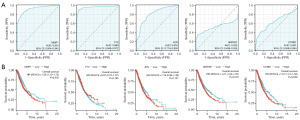
Molecular docking studies
In the process of screening the DEGs, MMP1 was identified as a key gene found in the compound luteolin from Sophorae Flavescentis radix. The 3D structure of luteolin was obtained from Pubchem, and the receptor protein structure of the key target MMP1 was retrieved from the PDB. The 3D structures of the active compound and the receptor protein structure of the key target were uploaded to DockThor for the online molecular docking analysis. The results revealed that the binding affinity between MMP1 and luteolin was −8.9. Further visualization was performed using the CB-DOCK2 database (Figure 4). Based on the strength of the binding affinity, it was concluded that luteolin was indeed an effective compound exerting an action on MMP1.
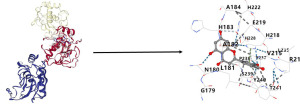
Identification of MMP1 interaction genes and proteins
The GeneMania data were used to build the gene-gene interaction network of MMP1 and its altered neighboring genes (Figure 5A). The STRING data were used to generate the protein-protein interaction network for MMP1 (Figure 5B).
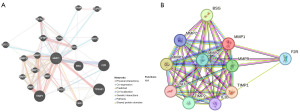
Pathway prediction by GSEA
A functional GSEA was conducted using the Metascape online tool. The analysis indicated that MMP1 might influence lung cancer biological processes through its involvement in several signaling pathways, including the chemokine signaling, apoptosis, Wingless/Integrated (Wnt) signaling, tumor protein p53 (TP53)-regulated cell cycle arrest, Hedgehog signaling, and mitogen-activated protein kinase (MAPK) signaling pathways. These pathways may contribute to the different prognoses observed in lung cancer patients (Figure 6).
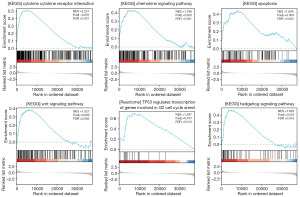
Discussion
In recent years, network pharmacology has become an essential tool in Chinese medicine research, particularly in exploring the multi-target and multi-component nature of TCM. The application of network pharmacology allows for the identification of active compounds and the prediction of potential molecular targets, offering a more comprehensive understanding of TCM’s therapeutic effects. For instance, network pharmacology has been successfully applied to reveal the potential pharmacological mechanisms of Astragalus in the treatment of glioma (14). Moreover, advancements in bioinformatics and database technology have improved the predictive power of network pharmacology, enabling more accurate identification of drug-target interactions. However, there are several challenges that need to be addressed when applying network pharmacology to drug research. One of the main difficulties lies in the accuracy of predictive models, which depend heavily on the comprehensiveness and quality of existing databases. These databases may not fully capture all possible drug-target interactions or account for off-target effects. Additionally, while network pharmacology can reveal potential molecular targets and pathways, translating these findings into clinically effective treatments requires rigorous experimental validation and clinical trials, which can be time-consuming and costly. Addressing these challenges will be crucial for advancing drug innovation and realizing the full potential of network pharmacology in future research.
In TCM, bone metastasis can be classified under the categories of “bone paralysis”, “bone tumor”, “bone carbuncle”, and “bone erosion”. Patients with chronic illness and physical weakness may experience bone metastasis due to the accumulation of pathological factors in the bones. The primary pathogenesis is centered around “pain due to lack of nourishment” and “pain due to obstruction”. Tumors and sores on the body are often the result of pathogenic factors invading a body weakened by deficient vital energy, disrupting the flow of vital energy, and causing cold and heat to congeal within the body. Supporting the vital energy (Fu Zheng) and eliminating the pathogenic factors (Qu Xie) are two critical aspects of the comprehensive treatment of tumors by TCM. The herbs used to eliminate pathogenic factors primarily cleanse, soften, and remove hard masses, and promote blood circulation to remove blood stasis. They play various roles, including inducing cell differentiation, promoting apoptosis, reversing multidrug resistance, and inhibiting invasion and metastasis. Therefore, clearing heat and detoxifying, as part of eliminating pathogenic factors, is one of the primary treatment methods for lung cancer bone metastasis.
In TCM, Sophorae Flavescentis radix is mainly used to treat damp-heat jaundice, diarrhea, dysentery, and skin itching. Modern pharmacological studies have shown that Sophorae Flavescentis radix possesses various pharmacological activities, including antioxidant, anti-inflammatory, anti-arrhythmic, anti-asthmatic, anti-ulcer, and anti-tumor effects (15). Research on its anti-tumor effects indicates that Sophorae Flavescentis radix and its preparations play significant roles in anti-tumor activity, reducing adverse reactions to chemotherapy, and reversing tumor drug resistance.
Using network pharmacology, this study identified 28 active compounds in Sophorae Flavescentis radix with a total of 227 target genes. By intersecting these target genes with the 952 DEGs related to lung cancer bone metastasis obtained from the GSE175601 dataset in the GEO database, we identified five common DEGs (i.e., F10, JUN, AKR1B1, MMP1, and CCND1). Based on the DEGs in lung cancer tumor tissues and their correlation with the prognosis of lung cancer patients, we ultimately identified MMP1 as the candidate gene for this study.
Matrix metalloproteinases (MMPs) are a family of proteases containing metal ions (zinc ions) that degrade various proteins in the extracellular matrix, including collagen, gelatin, elastin, and fibronectin (16). MMP1 was the first gene discovered in the MMP family. It encodes a collagenase with relatively high affinity for type III collagen, and it is located on the long arm of chromosome 11. Due to its fundamental role in collagen degradation, the aberrant expression of MMP1 is often associated with the local invasion and metastasis of tumor cells (17). Zhou et al. (18) conducted a bioinformatics analysis and found that MMP1 was related to non-small cell lung cancer (NSCLC). Gabasa et al. (19) confirmed that MMP1 can drive tumor progression in large cell lung carcinoma through fibroblast senescence. Additionally, MMP1 has been implicated in tumor bone metastasis; for example, Lu et al. (20) found that ADAMTS1 and MMP1 proteolysis are involved in the osteolytic signaling cascade of breast cancer bone metastasis. In molecular docking, the primary components of Sophorae Flavescentis radix showed strong binding interactions with the core target MMP1, which also validated the effective relationship between Sophorae Flavescentis radix and the treatment of lung cancer bone metastasis, demonstrating its potential research value.
Pathway prediction based on a GSEA suggested that MMP1 may influence lung cancer biological processes by participating in several signaling pathways, including the chemokine signaling, apoptosis, Wnt signaling, TP53-regulated cell cycle arrest, Hedgehog signaling, and MAPK signaling pathways. These mechanisms are also supported by related research in lung cancer bone metastasis. For instance, Wang et al. (21) established a bone metastasis xenograft model by implanting NCI-H460-luc2 lung cancer cells and found that the TCM Qilian formula inhibited tumor bone metastasis by suppressing osteoclastogenesis and promoting apoptosis. Xu et al. (22) detected blood samples from NSCLC patients and found that single nucleotide polymorphisms in the Wnt signaling pathway are associated with a reduced risk of bone metastasis and may serve as valuable biomarkers for bone metastasis in NSCLC patients. Xi et al. (23) also showed the role of anomalous activation of the Wnt signaling in lung cancer bone metastasis in malignant tumors, as well as the potential therapeutic application of targeting the Wnt pathway for the treatment of lung cancer and cancer-induced bone metastasis.
Conclusions
Sophorae Flavescentis radix may exert its therapeutic effects on lung cancer bone metastasis by inhibiting MMP1 expression and regulating the anomalous instigation of the Wnt signaling pathway. This hypothesis aligns with the observed molecular interactions and signaling pathways identified by our network pharmacology and bioinformatics analyses, providing a plausible mechanism for the anti-cancer activity of lung cancer bone metastasis. However, this study had certain limitations, including a lack of relevant preclinical experiments and the absence of large-sample, multicenter clinical data validation. Thus, future studies need to be conducted.
Acknowledgments
Funding: None.
Footnote
Reporting Checklist: The authors have completed the STREGA reporting checklist. Available at https://tcr.amegroups.com/article/view/10.21037/tcr-24-1947/rc
Peer Review File: Available at https://tcr.amegroups.com/article/view/10.21037/tcr-24-1947/prf
Conflicts of Interest: All authors have completed the ICMJE uniform disclosure form (available at https://tcr.amegroups.com/article/view/10.21037/tcr-24-1947/coif). The authors have no conflicts of interest to declare.
Ethical Statement:
Open Access Statement: This is an Open Access article distributed in accordance with the Creative Commons Attribution-NonCommercial-NoDerivs 4.0 International License (CC BY-NC-ND 4.0), which permits the non-commercial replication and distribution of the article with the strict proviso that no changes or edits are made and the original work is properly cited (including links to both the formal publication through the relevant DOI and the license). See: https://creativecommons.org/licenses/by-nc-nd/4.0/.
References
- Liu X, Yu Y, Wang M, et al. The mortality of lung cancer attributable to smoking among adults in China and the United States during 1990-2017. Cancer Commun (Lond) 2020;40:611-9. [Crossref] [PubMed]
- Kim D, Lee JW. Current Status of Lung Cancer and Surgery Based on Studies Using a Nationwide Database. J Chest Surg 2022;55:1-9. [Crossref] [PubMed]
- Feng RM, Zong YN, Cao SM, et al. Current cancer situation in China: good or bad news from the 2018 Global Cancer Statistics? Cancer Commun (Lond) 2019;39:22. [Crossref] [PubMed]
- Hong S, Youk T, Lee SJ, et al. Bone metastasis and skeletal-related events in patients with solid cancer: A Korean nationwide health insurance database study. PLoS One 2020;15:e0234927. [Crossref] [PubMed]
- Wang L, Yan X, Zhao J, et al. Expert consensus on resection of chest wall tumors and chest wall reconstruction. Transl Lung Cancer Res 2021;10:4057-83. [Crossref] [PubMed]
- Asano Y, Hayashi K, Takeuchi A, et al. Combining dynamics of serum inflammatory and nutritional indicators as novel biomarkers in immune checkpoint inhibitor treatment of non-small-cell lung cancer with bone metastases. Int Immunopharmacol 2024;136:112276. [Crossref] [PubMed]
- Zhang X, Qiu H, Li C, et al. The positive role of traditional Chinese medicine as an adjunctive therapy for cancer. Biosci Trends 2021;15:283-98. [Crossref] [PubMed]
- Rizvi SAA, Einstein GP, Tulp OL, et al. Introduction to Traditional Medicine and Their Role in Prevention and Treatment of Emerging and Re-Emerging Diseases. Biomolecules 2022; [Crossref] [PubMed]
- Xin Y, Sang Y, Ji X, et al. Explore the Constituents and Mechanism of Traditional Chinese Medicine Preparation Zhachong Shisan Pills Based on HPLC-QTOF-MS and Network Pharmacology. Curr Pharm Anal 2023;19:712-34. [Crossref]
- Zhou W, Wu C, Zhao C, et al. An Advanced Systems Pharmacology Strategy Reveals AKR1B1, MMP2, PTGER3 as Key Genes in the Competing Endogenous RNA Network of Compound Kushen Injection Treating Gastric Carcinoma by Integrated Bioinformatics and Experimental Verification. Front Cell Dev Biol 2021;9:742421. [Crossref] [PubMed]
- Chen MH, Gu YY, Zhang AL, et al. Biological effects and mechanisms of matrine and other constituents of Sophora flavescens in colorectal cancer. Pharmacol Res 2021;171:105778. [Crossref] [PubMed]
- Luo D, Dai X, Tian H, et al. Sophflarine A, a novel matrine-derived alkaloid from Sophora flavescens with therapeutic potential for non-small cell lung cancer through ROS-mediated pyroptosis and autophagy. Phytomedicine 2023;116:154909. [Crossref] [PubMed]
- Song Z, Yu J, Wang M, et al. CHDTEPDB: Transcriptome Expression Profile Database and Interactive Analysis Platform for Congenital Heart Disease. Congenit Heart Dis 2023;18:693-701. [Crossref]
- Feng Y, Zhu P, Wu D, et al. A Network Pharmacology Prediction and Molecular Docking-Based Strategy to Explore the Potential Pharmacological Mechanism of Astragalus membranaceus for Glioma. Int J Mol Sci 2023; [Crossref] [PubMed]
- Li JJ, Zhang X, Shen XC, et al. Phytochemistry and biological properties of isoprenoid flavonoids from Sophora flavescens Ait. Fitoterapia 2020;143:104556. [Crossref] [PubMed]
- Raeeszadeh-Sarmazdeh M, Do LD, Hritz BG. Metalloproteinases and Their Inhibitors: Potential for the Development of New Therapeutics. Cells 2020; [Crossref] [PubMed]
- Ng L, Wong SK, Huang Z, et al. CD26 Induces Colorectal Cancer Angiogenesis and Metastasis through CAV1/MMP1 Signaling. Int J Mol Sci 2022; [Crossref] [PubMed]
- Zhou H, Xiang Q, Hu C, et al. Identification of MMP1 as a potential gene conferring erlotinib resistance in non-small cell lung cancer based on bioinformatics analyses. Hereditas 2020;157:32. [Crossref] [PubMed]
- Gabasa M, Radisky ES, Ikemori R, et al. MMP1 drives tumor progression in large cell carcinoma of the lung through fibroblast senescence. Cancer Lett 2021;507:1-12. [Crossref] [PubMed]
- Lu X, Wang Q, Hu G, et al. ADAMTS1 and MMP1 proteolytically engage EGF-like ligands in an osteolytic signaling cascade for bone metastasis. Genes Dev 2009;23:1882-94. [Crossref] [PubMed]
- Wang Q, Zhao B, Li J, et al. Qilian Formula Inhibits Tumor Cell Growth in a Bone Metastasis Model of Lung Cancer. Integr Cancer Ther 2023;22:15347354231217274. [Crossref] [PubMed]
- Xu Y, Li H, Weng L, et al. Single nucleotide polymorphisms within the Wnt pathway predict the risk of bone metastasis in patients with non-small cell lung cancer. Aging (Albany NY) 2020;12:9311-27. [Crossref] [PubMed]
- Xi Y, Chen Y. Wnt signaling pathway: implications for therapy in lung cancer and bone metastasis. Cancer Lett 2014;353:8-16. [Crossref] [PubMed]
(English Language Editor: L. Huleatt)