Research progress on the correlation between obesity and the occurrence and development of kidney cancer: a narrative review
Introduction
In recent years, the incidence of kidney cancer has been increasing year by year. The global incidence of kidney cancer increased by 155% in 2019 compared to 1990 (1). According to the Global Cancer Statistics 2020, urinary cancers account for 13.1% of new cancer cases and 7.9% of total cancer mortality, of which kidney cancer is a common malignant tumor of the urinary system, accounting for 2.2% of the total cancer incidence and 1.8% of the total cancer mortality (2). Common carcinogenic factors such as smoking, obesity, hypertension, type II diabetes, alcohol, and occupational exposure are considered to be the main causes of kidney cancer (1,3). Obesity is a worldwide health problem, and over the past decade, new evidence has emerged for the association between obesity and cancer risk and prognosis, which has been shown to be associated with 13 cancer types and is a recognized risk factor for the development of renal cell carcinoma (RCC) (4-6). At the same time, the underlying mechanism of obesity on the occurrence and progression of kidney cancer has also been newly understood. We present this article in accordance with the Narrative Review reporting checklist (available at https://tcr.amegroups.com/article/view/10.21037/tcr-24-744/rc).
Methods
A non-systematic review of the latest literature to investigate the progress of the correlation between obesity and kidney cancer was carried out. Relevant articles in English available in the PubMed, Web of Science and Embase as at 15 July, 2024 were included. Search terms included (“Obesity”) AND (“kidney cancer” OR “Epidemiology” OR “Perirenal fat” OR “Mechanism” OR “Prognosis”). The secondary references cited in articles obtained from the PubMed search were also retrieved (Table 1).
Table 1
Items | Specification |
---|---|
Date of search | March 4, 2024 and July 15, 2024 |
Databases and other sources searched | PubMed, Web of Science and Embase |
Search terms used | (“Obesity”) AND (“kidney cancer” OR “Epidemiology” OR “Perirenal fat” OR “Mechanism” OR “Prognosis”) |
Timeframe | 2000–2024 |
Inclusion and exclusion criteria | Inclusion criteria: original articles, review articles; written in English only |
Exclusion criteria: case reports, letters to the editor; non-English language | |
Selection process | L.K. independently conducted the search; all the authors contributed to final version of the paper |
Epidemiological association of obesity with kidney cancer
RCC is the most common type of kidney cancer, accounting for the vast majority (90%) of cases, mainly including clear cell renal cell carcinoma (ccRCC; 70%), papillary renal cell carcinoma (pRCC; 10–15%) and chromophobe renal cell carcinoma (cRCC; 5%) (7). The most common histological subtype, ccRCC, arises from proximal tubular epithelial cells and causes most cancer-related deaths (8), characterized by the presence of lipid droplets in the cells (9). This intracellular accumulation of large amounts of lipids and glycogen may indicate that metabolic alterations play a role in the biology and pathogenesis of ccRCC, particularly lipid metabolism (10). According to the World Health Organization (WHO), RCC is the 6th most commonly diagnosed malignancy in men and the 10th most common in women (11). Studies of different countries and age groups have shown that the incidence of kidney cancer in men is about twice that of women (12,13). The incidence of kidney cancer increases steadily with age, and globally, the median age at diagnosis is around 75 years (14). Geographically, the regions with the highest incidence of kidney cancer are North America and Europe, and the higher the median income, the higher the incidence of kidney cancer (13,14). Of course, this may be related to the reason that abdominal imaging examinations are more common in high-income areas and there is a stronger awareness of health check-ups. In the reported incidence by race in the United States, blacks (age-standardized rate 17.0/100,000) have a higher incidence of kidney cancer than whites (age-standardized rate 13.2/100,000), with African Americans, Native Americans, and Alaska Natives having the highest susceptibility, and Asian Americans and people of Pacific Island descent having the lowest susceptibility (15-17).
Obesity is characterized by excess body fat, which the WHO defines as “abnormal or excessive fat accumulation that may harm health” (18), and it is caused by a complex interplay among genetic, environmental, socioeconomic and behavioural factors (19). Body mass index (BMI) is a good proxy for assessing overall body fat, and in adults, overweight is defined as a BMI of 25–29.9 kg/m2 and obesity is BMI ≥30 kg/m2. BMI greater than 30 kg/m2 is further classified as follows: grade I: BMI of 30.0–34.9 kg/m2, grade II: BMI of 35.0–39.9 kg/m2, and grade III: BMI of greater than 40 kg/m2 (20). Global obesity rates have almost tripled since 1975 (18), with 2.7 billion adults expected to be overweight by 2025, more than 1 billion obese and 177 million extremely obese, while by 2030, about 38% of the global adult population will be overweight and another 20% obese (21). Many epidemiological studies have shown that obesity is associated with an increased risk of cancer in multiple parts of the body (4,22,23). Based on data from observational studies in International Agency for Research on Cancer (IARC) Working Group 2016 (4) and World Cancer Research Fund and American Institute for Cancer Research under Continuous Update Project (WCRF/AICR CUP) 2018 (24), there is strong or probable evidence that being overweight or obese increases the risk of esophageal (adenocarcinoma), stomach (cardia), colorectal, liver, gallbladder, pancreas, kidney (RCC), endometrial, ovarian, and breast (postmenopausal women) cancer. Approximately 20% of kidney cancer worldwide is attributable to obesity, primarily due to higher levels of central obesity [visceral adipose tissue (VAT)] (14). In the study conducted by the WCRF CUP group, a 5 kg/m2 increase in BMI was associated with a 1.30 increase in the associated risk of kidney cancer. A British cohort study noted that for every 1-point increase in BMI, the risk of kidney cancer increased by 4% (25). Another pooled analysis of three America cohort studies also found that a higher BMI was associated with a significantly increased risk of kidney cancer (RCC), with an overall risk ratio of 2.16 [95% confidence interval (CI): 1.77–2.63] for a BMI of ≥30 kg/m2 versus a BMI of 18–25 kg/m2 (26). A Sweden study of associations between BMI in youth and site-specific cancer in men showed a linear association between increased BMI and the risk of kidney cancer (27). In the two most recent meta-analyses, Liu et al. (28) included 24 cohort studies that concluded that overweight/obesity increases the risk of kidney cancer in both men and women, and Shi et al. (29) also showed that the risk of kidney cancer increases with higher BMI. Globally, obesity prevalence varies by region, ethnicity, and gender. Europe and North America have the highest prevalence of obesity (30). An American study showed an overall prevalence of obesity of about 35 percent, with higher rates among Hispanics (43 percent) and African Americans (48 percent) (31,32). Interestingly, this coincides with areas with a high incidence of kidney cancer and susceptible populations. The population attributable fraction (PAF) of obesity-related cancers is 11.9% for men and 13.1% for women among all obesity-related malignancies, with 16.6% for men and 25.9% for women with kidney cancer (33). This contradicts the difference in the incidence of kidney cancer between the sexes, which is derived from BMI statistics. However, BMI is an indirect indicator and does not reflect the difference between fat and non-fat tissue, nor does it reflect the location of fat (centrally, periphery, or in organs) (34). Females and males have different fat distributions, with females being more susceptible to obesity as classified by BMI (32), while males are more likely to develop visceral obesity (35). This may explain the high incidence of kidney cancer in men and the high PAF in obesity in women.
In the latest meta-analysis on the impact of obesity on RCC, Shi et al. (29) included studies prior to July 2023. We systematically searched PubMed, Web of Science and Embase from July 2023 to July 2024 and included six articles, all of which supported the idea that obesity increases the incidence or mortality of kidney cancer (Table 2).
Table 2
Study | Year | Country | Sex | Remark |
---|---|---|---|---|
Maimaitiyiming et al. (36) | 2023 | UK | M/F | Obesity-related dietary pattern |
Deng et al. (37) | 2023 | US | M/F | Lifetime body weight trajectories |
Wang et al. (38) | 2024 | UK | M/F | Metabolic syndrome |
Onerup et al. (39) | 2023 | Sweden | M | BMI and mortality |
Suarez Arbelaez et al. (40) | 2023 | US | M/F | BMI and morbidity, a cross-sectional study |
Onerup et al. (27) | 2024 | Sweden | M | BMI and morbidity in young men, a cohort study |
RCC, renal cell carcinoma; M, male; F, female; BMI, body mass index.
Potential mechanisms of obesity leading to the development of kidney cancer
Although the mechanism by which obesity leads to the development of kidney cancer has not been fully elucidated, the latest research highlights the role of obesity-related factors in cancer (35). Excess body fat is associated with metabolic and endocrine abnormalities and it can stimulate the occurrence and progression of obesity-related cancers (41-43). Excess body fat is associated with metabolic and endocrine abnormalities and can stimulate the occurrence and progression of obesity-related cancers. At present, little research is known on the pathways and potential mechanisms related to the occurrence and development of obesity and ccRCC, mainly including the following aspects (Figure 1).
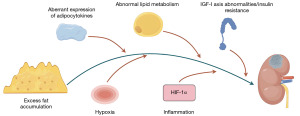
Abnormal expression of adipose tissue and adipocytokines
Adipose tissue is the most prevalent tissue in the human body. It is commonly found in the subcutaneous connective tissues and also surrounds various organs, including the kidneys. The development of obesity leads not only to an increase in the fat depot in the classical anatomical position, but also to the deposition of lipids in and around other tissues and organs, a phenomenon known as ectopic adipose deposition (44). Adipose tissue is not only a storage site for lipids, but also a major endocrine gland that secretes a variety of adipokines, including adiponectin, leptin, resistin, plasminogen activator inhibitor-1 (PAI 1), tumor necrosis factor α (TNF-α), vascular endothelial growth factor (VEGF), and interleukin 6 (IL-6) (45,46). With the continuous weight gain, the secretion function of adipose tissue is dysregulated, and the physiological state of perirenal fat may have carcinogenic effects and affect the progression of kidney tumors (47,48). Adiponectin is currently the focus of research on adipocytokines.
Adiponectin
Adiponectin, which is mainly secreted by adipocytes and was first reported in 1995 as the adipocyte complement-related protein 30 (Acrp30), was thought to be specifically expressed only in adipose tissue and differentiated adipocytes (49), and has now been found to be produced by bone marrow, osteoblasts, etc. (50,51). Adiponectin has been shown to have a variety of functions in the human body, balancing glucose and lipid metabolism, insulin-sensitizing, anti-apoptosis, and immunomodulatory effects (52). Under the regulation of transcription, translation, and post-translational level feedback loops, adiponectin was negatively correlated with BMI and central obesity, and negatively correlated with waist-to-hip ratio (53,54). Multiple evidence suggests that low levels of adiponectin are associated with a risk of several cancers, including breast, endometrial, non-small cell lung, renal cell, pancreatic, liver, prostate, stomach, and colon (55-58). Several case-control studies have shown a relationship between adiponectin and kidney cancer (59,60), two of which have been linked to distant metastasis and vascular invasion of kidney cancer (61,62). In a study that measured the predictability of preoperative serum adiponectin in predicting cancer-specific survival in patients with RCC, patients with high adiponectin levels had an 83% lower risk of death due to RCC (63). Another study showed a significant reduction in serum levels of total adiponectin and polymer adiponectin in patients with metastatic kidney cancer compared with non-metastatic RCC (64). Numerous studies have shown that adiponectin exerts anti-tumor effects on cancer mainly through two mechanisms. First, it can affect tumor growth by acting directly on cancer cells through a receptor-mediated pathway (65). It is mainly for the activation of adenosine monophosphate-activated protein kinase (AMPK). Other avenues are mitogen-activated protein kinase (MAPK) and phosphatidylinositol 3 kinase (PI3K)/protein kinase B (Akt). AMPK inhibits the induction of tumor formation by influencing cell growth through mammalian target of rapamycin (mTOR) signaling pathway targets (66). As a key regulator of energy balance, AMPK addresses metabolic abnormalities by shutting down anabolic and proliferative pathways in response to inflammation and oxidative stress, while increasing adenosine triphosphate (ATP) production (67). Adiponectin induces growth arrest and apoptosis by activating AMPK in various cell lines in a p53- and p21-dependent manner and by various cellular mediators including the adaptor protein containing pleckstrin homology (PH) domain, phosphotyrosine binding (PTB) domain and leucine zipper motif 1 (APPL-1), calcium-dependent kinase, and liver kinase B1 (LKB1) (68). For example, adiponectin inhibits colon cancer cell proliferation by inducing p27 and p21 and impairing the cell cycle during the G1/S transition (69), induces the tumor suppressor gene LKB1, which leads to AMPK activation and inhibits cell adhesion, invasion and migration in breast cancer cell lines (70,71). The role of adiponectin in MAPK signaling remains controversial (66). In addition, some studies have shown that PI3K/Akt and cyclin D1 signaling are also associated with adiponectin-mediated growth arrest and apoptosis (72). Second, adiponectin may indirectly affect tumor invasion and metastasis by modulating insulin sensitivity, inflammation, and tumor angiogenesis (65). Data have shown that insulin can promote the proliferation of tumor cells (73). Serum adiponectin levels appear to be inversely correlated with fasting insulin concentrations and decrease under conditions of insulin resistance (74). Adiponectin has been shown to have anti-inflammatory effects and interfere with cancer progression by inhibiting TNF-α. Adiponectin-deficient mice do exhibit increased levels of TNF-α-encoding mRNA in adipose tissue and higher concentrations of TNF-α in plasma compared to wild-type mice. In addition, adiponectin exhibits a negative effect on macrophage phagocytic activity (75). In terms of antiangiogenesis, adiponectin hinders angiogenesis and reduces macrophage infiltration by inhibiting the mTOR and Stat3 pathways and stimulating AMPK and caspase activity (76). However, the results reported in the literature appear to be contradictory, and there are also convincing studies that suggest that adiponectin may have a potent pro-angiogenic effect and may promote cancer development (77). This contradictory result suggests that the regulation of angiogenesis by adiponectin warrants further investigation.
Abnormal lipid metabolism
Both RCC and obesity are considered metabolic diseases, and lipid metabolism plays an important role in the occurrence and development of both diseases. ccRCC is a highly metabolically functional tumor characterized by a “clear cell” appearance due to excessive accumulation of lipid droplets and glycogen. The lipid droplets formed by this large amount of lipid deposition can provide sufficient energy for cell membrane formation and signaling of tumor cells in the form of fatty acids, and promote cancer cell proliferation and metastasis (10). Recent studies have focused on the molecular mechanisms of lipid metabolism in RCC and the search for effective targets for the treatment of RCC with abnormal lipid metabolism. Ancient ubiquitous protein 1 (AUP1; a lipid droplet-regulated very low-density lipoprotein assembly factor) can promote the synthesis of intracellular fatty acids and the formation of cholesteryl esters by targeting the sterol O-acyltransferase 1 (SOAT1) pathway, and induce lipid accumulation in ccRCC tissues by inhibiting β-oxidation of fatty acids, regulating lipolysis and cholesterol transport, thereby promoting the proliferation and metastasis of ccRCC cells (78). The Hippo pathway is able to alter tumor metabolism and is associated with lipid metabolism. Miao et al. demonstrated that Hippo signaling regulated by the dihydrolipoamide branched-chain transacylase (DBT) E2/Recombinant Annexin A2 (ANXA2)/Yes-associated protein (YAP) axis has a tumor suppressive effect. They believe that DBT is a tumor suppressor that inhibits tumor progression and corrects lipid metabolism disorders in ccRCC, and suggests that DBT can be used as a potential target for pharmacological intervention in ccRCC (79). In view of the fact that abnormal lipid metabolism is a common pathogenesis of RCC and obesity, and obesity is an important risk factor for RCC, it is worth exploring whether there is a mechanism of obesity reverse to promote the occurrence and development of RCC by aggravating abnormal lipid metabolism.
Abnormalities in the insulin-like growth factor-I (IGF-I) axis and hyperinsulinemia/insulin resistance
In obese individuals, changes in adiponectin levels and other reasons lead to reduced insulin sensitivity, insulin resistance, insulin cannot bind to receptors, glucose cannot enter cells and remains in the blood in large quantities. The continuous increase in blood glucose causes the pancreatic β cells to compensate for the secretion of more insulin, manifesting as elevated levels of circulating insulin and insulin-like growth factor (IGF) (80). The data showed that every 1 kg/m2 increase in BMI increased fasting insulin levels by 8.5% (81). Johansson et al. demonstrated a significant association between fasting insulin-related variables and the risk of RCC, with an increase of one standard deviation (SD) in fasting insulin increasing the risk of RCC by 82% (82). High serum insulin concentrations may inhibit autophagy, proteasome activity, and apoptosis through the insulin action of its receptors, exert anti-apoptotic and mitotic effects (83), and secondly, enhance the growth and proliferation of cancer cells by activating the IGF pathway. The interaction between insulin and the IGF system, which includes IGF-1, IGF-2 and its receptors (IGF-1R and IGF-2R), and six types of IGF-binding proteins (IGFBPs), appears to be critical to the development and progression of RCC. Insulin directly or indirectly regulates hepatic IGF-1 production through upregulation of growth hormone (GH) receptors and increases IGF-1 bioavailability by downregulating IGFBP-1 and IGFBP-2 (hindering IGF-1 action) (84). IGF-1 is thought to stimulate tumor angiogenesis, and inhibition of IGF-1R signaling is a fundamental mechanism in RCC biology (85). Binding of IGF-1 to IGF-1R activates tyrosine kinase signaling and phosphorylation of insulin receptor substrate (IRS) protein, thereby activating the PI-3K/Akt/mTOR (mammalian target of rapamycin) and Ras/MAPK pathways (86). These pathways have been shown to regulate apoptosis and cell proliferation and may be involved in the development of cancer. Takahashi found that the expression of insulin receptor (IR) on RCC cells was inversely correlated with cancer stage and the occurrence of distant metastases, and this value was significantly reduced in patients with tumor-stage pT2–4 and distant metastases. In addition, enhanced IR expression is strongly associated with better disease-free survival and overall survival after nephrectomy (87). However, they did not clearly observe the pro-tumor effect of hyperinsulinemia in clinical and mouse experimental model studies, and the decrease in IR expression in high-stage RCC tumors with poor prognosis may be the result of host hyperinsulinemia-induced downregulation. Another study showed that the lack of IR on RCC cell lines was not associated with inhibition of insulin activity in RCC cells, which remained susceptible to insulin stimulation through IGF-1R (86). These results seem to suggest that insulin is more likely to play a role in the growth and proliferation of cancer cells through interaction with the IGF system, or that the IGF system appears to be a more effective stimulator of cancer cell proliferation.
Hypoxia
Hypoxia is a hallmark of solid tumors, and the effects of obesity on adipose tissue vascular function and oxygen supply have been well studied. Hypertrophy and expansion of adipose tissue affects the tumor microenvironment to stimulate hypoxia, and the limited supply of oxygen triggers compensatory angiogenesis mechanisms in response to oxygen and nutrient deficiencies (88). There are two main hypoxia-inducing factors (HIFs) that mediate hypoxia responses: HIF-1α and HIF-2α. Decreased partial pressure of oxygen and elevated HIF-1α expression in adipose tissue can trigger adipose tissue inflammation and dysfunction in obese patients (89). Von Hippel-Lindau (VHL) mutation inactivation is a genetic event in most ccRCC whose encoded amino acid protein (pVHL) leads to aberrant activation of HIFs, which induces gene expression programs by altering cellular metabolism, inducing angiogenesis, and promoting epithelial-mesenchymal transition, invasion, and metastatic diffusion, which play a key role in the pathogenesis of ccRCC (90). Previous studies have suggested that HIF-2α is the main pro-tumor factor in ccRCC, and HIF-1α appears to inhibit tumor invasion. ccRCC expressing only HIF-2α has a higher rate of proliferation than tumors expressing HIF-1α and HIF-2α (91), and knockdown of HIF-1α enhances xenograft tumor formation in cell lines expressing both HIF-1α and HIF-2α (92). Recent studies have demonstrated that HIF-1α is also essential for tumor formation, and that both HIF-1α and HIF-2α are required for ccRCC, and that alterations in the balance of activity of both can affect different aspects of ccRCC biology and disease aggressiveness (90). HIF-1α and HIF-2α may play different roles at different stages of tumor formation and progression, and these effects may be regulated by the spectrum of mutations present in each individual ccRCC.
Chronic inflammation
Chronic inflammation has been shown to promote cancer progression. Obesity is associated with the secretion of a large number of pro-inflammatory cytokines that produce a low-grade chronic inflammatory state. This chronic inflammatory state maintained by adipocytes may modulate host immune surveillance, resulting in direct effects on the local tumor microenvironment and distant tumor cells (through systemic effects of endocrine signaling) (93). Systemic inflammation is often observed in the late stages of RCC. Studies have shown that levels of IL-10 protein are higher in more advanced tumor node metastasis (TNM) classification tumors (pT3). IL-6, IL-10, and heat shock protein 90 (HSP-90) have been identified as markers of RCC development and progression (94). In addition, the expression of inflammation-related genes and neutrophil infiltration in tumor tissues are prognostic markers for metastatic RCC (95). A recent study showed that the pro-inflammatory factor angiopoietin-like protein 8 (ANGPTL8) can induce the expression of CXC motif chemokine ligand 1 (CXCL1) and CXC motif chemokine ligand 2 (CXCL2) chemokines by activating the nuclear factor kappa-B (NF-κB) signaling pathway, maintain cancer cells in an undifferentiated state, and play an important role in ccRCC tumor progression by establishing a tumor microenvironment (96).
Effect of perirenal adipose tissue (PAT) on renal cancer progression
Adipose tissue is divided into subcutaneous adipose tissue (SAT) and VAT, which are significantly different in terms of adipokine secretion, lipolytic activity and endocrine. VAT is an endocrine organ that secretes a variety of hormones and cytokines associated with carcinogenesis and tumor progression (97). PAT is a type of VAT between the renal fascia and the renal fibrous sac that surrounds the kidneys and supports their function.
PAT is a complex microenvironment consisting of a mixture of white adipocytes and dormant, active brown adipocytes. Because of its anatomically adjacent relationship to the kidney, PAT is able to regulate renal activity through paracrine or endocrine mechanisms such as adiponectin, leptin, resistin, TNF-α, visceral lipids, IL-6, and IL-1β (98). In addition, there has been recent evidence that PAT is involved in local tumor invasion, and during tumorigenesis, ccRCC cells can infiltrate through the renal fibrous sac and enter PAT, a process that is associated with poor prognosis of renal cancer (99). BMI, as a widely used indicator in clinical practice to assess obesity, has limitations in measuring body fat distribution. Computed tomography (CT) and magnetic resonance imaging have been used to assess the distribution of fat in the body. Includes measurements of SAT, VAT, perirenal fat thickness (PFT), perirenal fat area (PFA), and perirenal fat volume (PFV). In a study of PFT measurements in 174 patients, high PFT predicted poor progression-free survival in patients with localized ccRCC (100). A higher percentage of PFA in surgically treated patients with ccRCC also suggests an independent association with an increased risk of death, and perirenal fat is thought to be a new independent prognostic factor for surgical treatment of patients with ccRCC (101). Controversially, the findings of Preza-Fernandes et al. (102) are consistent with the obesity paradox, in which obese patients were observed to have greater PFA and prevent ccRCC disease progression and death. This may be due to a decrease in fat density in larger PFAs, which reduces metabolic activity and produces less secretory signaling. Wei et al. (103) found that there is bidirectional communication between ccRCC cells and PAT, and that ccRCC cells secrete parathyroid hormone-related protein (PTHrP) and promote PAT browning through protein kinase A (PKA) activation. PAT-mediated thermogenesis where browning occurs leads to the release of excess lactate, which provides ample energy to ccRCC to enhance growth, invasion, and metastasis. Current studies have shown that perirenal fat infiltration is closely related to the development of ccRCC disease, but the effect of perirenal fat accumulation on survival outcomes remains controversial. The extent of radical nephrectomy requires free excision of the kidney and perirenal fat outside the perirenal fascia, so the completeness of perirenal fascial resection and the amount of residual perirenal fat may also have an important impact on the prognosis of patients with renal cancer. The measurement of perirenal fat distribution using imaging technology may be a new method to assess the prognosis of patients with renal cancer. New perspectives on PAT as an endocrine organ, and more recent knowledge that dormant brown adipocytes may be activated, are now considered a new area of research on the relationship between obesity and kidney cancer. Future in-depth research on PAT may provide new ideas for the treatment of kidney cancer (Figure 2).
Effect of perirenal fat on surgical treatment of RCC
Approximately 25% of clinical T1b/T2 kidney cancers involve perirenal fat, which means that if the Gerota fascia and perirenal fat are not completely removed by surgery, the prognosis of about 25% of patients with T1b/T2 disease will be affected in addition to T3a kidney cancer. Liu et al. (104) developed a tool to assess the integrity of surgical specimens for nephrectomy. Their study showed that retroperitoneal laparoscopic radical nephrectomy (RLRN) had poor integrity in removing the Gerota fascia and perirenal fat compared to transperitoneal laparoscopic radical nephrectomy (TLRN). This may be due to the transabdominal approach, which has better operating space and surgical visibility. It is hypothesized that the use of TLRN for large tumors can improve prognosis and improve the time to recurrence and survival of patients, but no survival data are provided. Perirenal fat adhesions limit the mobility of the kidney and make it more difficult for the kidney to be free. Mayo Adhesive Probability (MAP) score can quantitatively assess the possibility of perirenal fat adhesions, so as to effectively predict the difficulty of surgery. Studies have shown that the MAP score can have a significant impact on the clamp time of robotic-assisted partial nephrectomy (PN) (105). Trifecta was the primary criterion for evaluating the success of PN, and Campi et al. (106,107) found that renal characteristics were reported based on the preoperative aspects and dimensions used for an anatomical (PADUA) score or RENAL (radius, exophytic/endophytic properties, nearness to hilum, anterior/posterior location, and location relative to polar lines) score, tumor complexity and surgical methods were independent predictors of Trifecta failure after PN. At the same time, the 3D virtual model is reported to be available for reliable evaluation of surgical planning (108). Therefore, perirenal fat has an important impact on the surgical treatment of RCC, and the combination of MAP score with renal tumor scoring systems, such as RENAL score, PADUA score, 3D virtual model, etc., may more comprehensively and objectively evaluate the complexity of surgery such as laparoscopic/robotic PN, so as to guide clinical decision-making.
Conclusions
There is growing evidence that obesity plays an important role in the development and progression of kidney cancer. Although larger studies are needed to uncover the pathological mechanisms involved in cancer growth to conclusively determine the impact of obesity on the occurrence, progression, and prognosis of kidney cancer, the current epidemiological evidence appears to be sufficient to demonstrate that obesity is an important risk factor for kidney cancer. The emergence of the “obesity paradox” makes the relationship between obesity and the prognostic outcome of kidney cancer still inconclusive. The quality and quantity of perirenal fat may replace BMI as a new indicator to study obesity and the prognosis of kidney cancer. Existing studies have shown that the underlying mechanisms of obesity and the occurrence and development of kidney cancer mainly include abnormal expression of adipocytokines, abnormal lipid metabolism, insulin resistance, abnormal IGF-I system and signaling, hypoxia and inflammation (Table 3). In addition, sex hormones, oxidative stress damage, and inactivation of tumor suppressor genes may also play a role in the mechanism of obesity-inducing kidney cancer. Despite current significant advances in the treatment of kidney cancer, disease recurrence and metastasis remain the leading cause of kidney cancer-related deaths. Further research on obesity and kidney cancer can expand to the treatment of kidney cancer. For example, pharmacological inhibition of adipocyte browning can enhance the anti-renal cancer efficacy of the targeted drug sunitinib (103). It also reminds us that monitoring health conditions, such as diabetes, high BMI, and high blood pressure, is equally important in the prevention and treatment of kidney cancer.
Table 3
ID | Correlation |
---|---|
1 | Obesity increases the risk of kidney cancer |
2 | Areas and ethnicities with high prevalence of obesity are highly consistent with areas with high incidence of kidney cancer and susceptible populations |
3 | Females are more susceptible to obesity by BMI classification, while males are more likely to develop visceral obesity, which may explain the high incidence of kidney cancer in males and the high PAF of kidney cancer due to obesity in females |
4 | The underlying mechanisms of obesity and the occurrence and development of kidney cancer mainly include abnormal expression of adipocytokines, abnormal lipid metabolism, insulin resistance, abnormal IGF-I system and signaling, hypoxia and inflammation |
5 | Perirenal adipose tissue can promote the occurrence of renal cancer through metabolic activity and secretion of adipokines |
6 | Perirenal fat has an important impact on the surgical treatment of RCC |
ID, identifier; BMI, body mass index; PAF, population attributable fraction; IGF-I, insulin-like growth factor-I; RCC, renal cell carcinoma.
After reading a large number of epidemiological literature on obesity and kidney cancer, this article summarizes the interaction between the two and adds our own interpretation and reflection on the epidemiological results. In the TNM stage of renal cancer, the invasion of tumor into PAT is defined as T3a. The authors believe that PAT, as an adipose tissue adjacent to the kidney, is inextricably related to the occurrence and development of kidney cancer. In this article, we summarize the clinical and basic research between perirenal fat and kidney cancer, hoping to provide reference for subsequent related studies.
This article has the following limitations. First of all, in this article, although the potential mechanism of obesity and kidney cancer is summarized, the specific mechanism is not described in depth and completely, such as the fact that leptin in adipocytokines is also closely related to kidney cancer, but it is not described in detail due to space considerations. Second, the article mentions the association between the “obesity paradox” and kidney cancer, but does not explain the mechanism that supports the “obesity paradox” view.
Acknowledgments
Funding: This study was supported by
Footnote
Reporting Checklist: The authors have completed the Narrative Review reporting checklist. Available at https://tcr.amegroups.com/article/view/10.21037/tcr-24-744/rc
Peer Review File: Available at https://tcr.amegroups.com/article/view/10.21037/tcr-24-744/prf
Conflicts of Interest: All authors have completed the ICMJE uniform disclosure form (available at https://tcr.amegroups.com/article/view/10.21037/tcr-24-744/coif). The authors have no conflicts of interest to declare.
Ethical Statement: The authors are accountable for all aspects of the work in ensuring that questions related to the accuracy or integrity of any part of the work are appropriately investigated and resolved.
Open Access Statement: This is an Open Access article distributed in accordance with the Creative Commons Attribution-NonCommercial-NoDerivs 4.0 International License (CC BY-NC-ND 4.0), which permits the non-commercial replication and distribution of the article with the strict proviso that no changes or edits are made and the original work is properly cited (including links to both the formal publication through the relevant DOI and the license). See: https://creativecommons.org/licenses/by-nc-nd/4.0/.
References
- Zi H, He SH, Leng XY, et al. Global, regional, and national burden of kidney, bladder, and prostate cancers and their attributable risk factors, 1990-2019. Mil Med Res 2021;8:60. [Crossref] [PubMed]
- Sung H, Ferlay J, Siegel RL, et al. Global Cancer Statistics 2020: GLOBOCAN Estimates of Incidence and Mortality Worldwide for 36 Cancers in 185 Countries. CA Cancer J Clin 2021;71:209-49. [Crossref] [PubMed]
- Al-Bayati O, Hasan A, Pruthi D, et al. Systematic review of modifiable risk factors for kidney cancer. Urol Oncol 2019;37:359-71. [Crossref] [PubMed]
- Lauby-Secretan B, Scoccianti C, Loomis D, et al. Body Fatness and Cancer--Viewpoint of the IARC Working Group. N Engl J Med 2016;375:794-8. [Crossref] [PubMed]
- Sung H, Siegel RL, Torre LA, et al. Global patterns in excess body weight and the associated cancer burden. CA Cancer J Clin 2019;69:88-112. [Crossref] [PubMed]
- Islami F, Goding Sauer A, et al. Proportion of Cancer Cases Attributable to Excess Body Weight by US State, 2011-2015. JAMA Oncol 2019;5:384-92. [Crossref] [PubMed]
- Motzer RJ, Jonasch E, Agarwal N, et al. Kidney Cancer, Version 3.2022, NCCN Clinical Practice Guidelines in Oncology. J Natl Compr Canc Netw 2022;20:71-90. [Crossref] [PubMed]
- Hsieh JJ, Purdue MP, Signoretti S, et al. Renal cell carcinoma. Nat Rev Dis Primers 2017;3:17009. [Crossref] [PubMed]
- Reuter VE, Tickoo SK. Differential diagnosis of renal tumours with clear cell histology. Pathology 2010;42:374-83. [Crossref] [PubMed]
- Klasson TD, LaGory EL, Zhao H, et al. ACSL3 regulates lipid droplet biogenesis and ferroptosis sensitivity in clear cell renal cell carcinoma. Cancer Metab 2022;10:14. [Crossref] [PubMed]
- Znaor A, Lortet-Tieulent J, Laversanne M, et al. International variations and trends in renal cell carcinoma incidence and mortality. Eur Urol 2015;67:519-30. [Crossref] [PubMed]
- Scelo G, Li P, Chanudet E, et al. Variability of Sex Disparities in Cancer Incidence over 30 Years: The Striking Case of Kidney Cancer. Eur Urol Focus 2018;4:586-90. [Crossref] [PubMed]
- Scelo G, Larose TL. Epidemiology and Risk Factors for Kidney Cancer. J Clin Oncol 2018; Epub ahead of print. [Crossref] [PubMed]
- Bukavina L, Bensalah K, Bray F, et al. Epidemiology of Renal Cell Carcinoma: 2022 Update. Eur Urol 2022;82:529-42. [Crossref] [PubMed]
- Giaquinto AN, Miller KD, Tossas KY, et al. Cancer statistics for African American/Black People 2022. CA Cancer J Clin 2022;72:202-29. [Crossref] [PubMed]
- Miller KD, Ortiz AP, Pinheiro PS, et al. Cancer statistics for the US Hispanic/Latino population, 2021. CA Cancer J Clin 2021;71:466-87. [Crossref] [PubMed]
- Siegel RL, Miller KD, Fuchs HE, et al. Cancer statistics, 2022. CA Cancer J Clin 2022;72:7-33. [Crossref] [PubMed]
- World Health Organization. Obesity and overweight [cited 2023 Jul 6]. Available online: https://www.who.int/news-room/fact-sheets/detail/obesity-and-overweight
- Jukaku SA, Williams SRP. The cause of obesity is multifactorial but GPs can do more. BMJ 2021;373: [Crossref] [PubMed]
- Sweatt K, Garvey WT, Martins C. Strengths and Limitations of BMI in the Diagnosis of Obesity: What is the Path Forward? Curr Obes Rep 2024;13:584-95. Erratum in: Curr Obes Rep 2024 [Epub ahead of print]. doi: 10.1007/s13679-024-00584-x. [Crossref] [PubMed]
- Kelly T, Yang W, Chen CS, et al. Global burden of obesity in 2005 and projections to 2030. Int J Obes (Lond) 2008;32:1431-7. [Crossref] [PubMed]
- Lee DH, Giovannucci EL. The Obesity Paradox in Cancer: Epidemiologic Insights and Perspectives. Curr Nutr Rep 2019;8:175-81. [Crossref] [PubMed]
- Choi EK, Park HB, Lee KH, et al. Body mass index and 20 specific cancers: re-analyses of dose-response meta-analyses of observational studies. Ann Oncol 2018;29:749-57. [Crossref] [PubMed]
- WCRF International. Diet, activity and cancer [cited 2023 Jul 6]. Available online: https://www.wcrf.org/diet-activity-and-cancer/
- Bhaskaran K, Douglas I, Forbes H, et al. Body-mass index and risk of 22 specific cancers: a population-based cohort study of 5·24 million UK adults. Lancet 2014;384:755-65. [Crossref] [PubMed]
- Graff RE, Wilson KM, Sanchez A, et al. Obesity in Relation to Renal Cell Carcinoma Incidence and Survival in Three Prospective Studies. Eur Urol 2022;82:247-51. [Crossref] [PubMed]
- Onerup A, Mehlig K, Af Geijerstam A, et al. Associations between BMI in youth and site-specific cancer in men-A cohort study with register linkage. Obesity (Silver Spring) 2024;32:376-89. [Crossref] [PubMed]
- Liu X, Sun Q, Hou H, et al. The association between BMI and kidney cancer risk: An updated dose-response meta-analysis in accordance with PRISMA guideline. Medicine (Baltimore) 2018;97:e12860. [Crossref] [PubMed]
- Shi X, Deng G, Wen H, et al. Role of body mass index and weight change in the risk of cancer: A systematic review and meta-analysis of 66 cohort studies. J Glob Health 2024;14:04067. [Crossref] [PubMed]
- Stevens GA, Singh GM, Lu Y, et al. National, regional, and global trends in adult overweight and obesity prevalences. Popul Health Metr 2012;10:22. [Crossref] [PubMed]
- Rawla P, Thandra KC, Sunkara T. Pancreatic cancer and obesity: epidemiology, mechanism, and preventive strategies. Clin J Gastroenterol 2019;12:285-91. [Crossref] [PubMed]
- Hruby A, Hu FB. The Epidemiology of Obesity: A Big Picture. Pharmacoeconomics 2015;33:673-89. [Crossref] [PubMed]
- Arnold M, Pandeya N, Byrnes G, et al. Global burden of cancer attributable to high body-mass index in 2012: a population-based study. Lancet Oncol 2015;16:36-46. [Crossref] [PubMed]
- Lu Y, Tang H, Huang P, et al. Assessment of causal effects of visceral adipose tissue on risk of cancers: a Mendelian randomization study. Int J Epidemiol 2022;51:1204-18. [Crossref] [PubMed]
- Avgerinos KI, Spyrou N, Mantzoros CS, et al. Obesity and cancer risk: Emerging biological mechanisms and perspectives. Metabolism 2019;92:121-35. [Crossref] [PubMed]
- Maimaitiyiming M, Yang H, Zhou L, et al. Associations between an obesity-related dietary pattern and incidence of overall and site-specific cancers: a prospective cohort study. BMC Med 2023;21:251. [Crossref] [PubMed]
- Deng Z, Hajihosseini M, Moore JX, et al. Lifetime Body Weight Trajectories and Risk of Renal Cell Cancer: A Large U.S. Prospective Cohort Study. Cancer Epidemiol Biomarkers Prev 2023;32:1651-9. [Crossref] [PubMed]
- Wang L, Du H, Sheng C, et al. Association between metabolic syndrome and kidney cancer risk: a prospective cohort study. Lipids Health Dis 2024;23:142. [Crossref] [PubMed]
- Onerup A, Mehlig K, Ekblom-Bak E, et al. Cardiorespiratory fitness and BMI measured in youth and 5-year mortality after site-specific cancer diagnoses in men-A population-based cohort study with register linkage. Cancer Med 2023;12:20000-14. [Crossref] [PubMed]
- Suarez Arbelaez MC, Nackeeran S, Shah K, et al. Association between body mass index, metabolic syndrome and common urologic conditions: a cross-sectional study using a large multi-institutional database from the United States. Ann Med 2023;55:2197293. [Crossref] [PubMed]
- Aurilio G, Piva F, Santoni M, et al. The Role of Obesity in Renal Cell Carcinoma Patients: Clinical-Pathological Implications. Int J Mol Sci 2019;20:5683. [Crossref] [PubMed]
- Quail DF, Dannenberg AJ. The obese adipose tissue microenvironment in cancer development and progression. Nat Rev Endocrinol 2019;15:139-54. [Crossref] [PubMed]
- Larsson SC, Spyrou N, Mantzoros CS. Body fatness associations with cancer: evidence from recent epidemiological studies and future directions. Metabolism 2022;137:155326. [Crossref] [PubMed]
- Qiu Y, Gan M, Wang X, et al. The global perspective on peroxisome proliferator-activated receptor γ (PPARγ) in ectopic fat deposition: A review. Int J Biol Macromol 2023;253:127042. [Crossref] [PubMed]
- Gu X, Wang L, Liu S, et al. Adipose tissue adipokines and lipokines: Functions and regulatory mechanism in skeletal muscle development and homeostasis. Metabolism 2023;139:155379. [Crossref] [PubMed]
- Lopez-Yus M, Hörndler C, Borlan S, et al. Unraveling Adipose Tissue Dysfunction: Molecular Mechanisms, Novel Biomarkers, and Therapeutic Targets for Liver Fat Deposition. Cells 2024;13:380. [Crossref] [PubMed]
- Gati A, Kouidhi S, Marrakchi R, et al. Obesity and renal cancer: Role of adipokines in the tumor-immune system conflict. Oncoimmunology 2014;3:e27810. [Crossref] [PubMed]
- Blee AM, Huang H. Fat lure: adipocytes attract cancer cells out of the prostate. Transl Cancer Res 2016;5:S123-5. [Crossref] [PubMed]
- Scherer PE, Williams S, Fogliano M, et al. A novel serum protein similar to C1q, produced exclusively in adipocytes. J Biol Chem 1995;270:26746-9. [Crossref] [PubMed]
- Wang GX, Zhao XY, Lin JD. The brown fat secretome: metabolic functions beyond thermogenesis. Trends Endocrinol Metab 2015;26:231-7. [Crossref] [PubMed]
- Brochu-Gaudreau K, Rehfeldt C, Blouin R, et al. Adiponectin action from head to toe. Endocrine 2010;37:11-32. [Crossref] [PubMed]
- Scherer PE. The Multifaceted Roles of Adipose Tissue-Therapeutic Targets for Diabetes and Beyond: The 2015 Banting Lecture. Diabetes 2016;65:1452-61. [Crossref] [PubMed]
- Cnop M, Havel PJ, Utzschneider KM, et al. Relationship of adiponectin to body fat distribution, insulin sensitivity and plasma lipoproteins: evidence for independent roles of age and sex. Diabetologia 2003;46:459-69. [Crossref] [PubMed]
- Rasmussen MS, Lihn AS, Pedersen SB, et al. Adiponectin receptors in human adipose tissue: effects of obesity, weight loss, and fat depots. Obesity (Silver Spring) 2006;14:28-35. [Crossref] [PubMed]
- Ohbuchi Y, Suzuki Y, Hatakeyama I, et al. A lower serum level of middle-molecular-weight adiponectin is a risk factor for endometrial cancer. Int J Clin Oncol 2014;19:667-73. [Crossref] [PubMed]
- Kosova F, Coskun T, Kaya Y, et al. Adipocytokine levels of colon cancer patients before and after treatment. Bratisl Lek Listy 2013;114:394-7. [Crossref] [PubMed]
- Kerenidi T, Lada M, Tsaroucha A, et al. Clinical significance of serum adipokines levels in lung cancer. Med Oncol 2013;30:507. [Crossref] [PubMed]
- Liao LM, Schwartz K, Pollak M, et al. Serum leptin and adiponectin levels and risk of renal cell carcinoma. Obesity (Silver Spring) 2013;21:1478-85. [Crossref] [PubMed]
- Spyridopoulos TN, Petridou ET, Skalkidou A, et al. Low adiponectin levels are associated with renal cell carcinoma: a case-control study. Int J Cancer 2007;120:1573-8. [Crossref] [PubMed]
- Liao LM, Weinstein SJ, Pollak M, et al. Prediagnostic circulating adipokine concentrations and risk of renal cell carcinoma in male smokers. Carcinogenesis 2013;34:109-12. [Crossref] [PubMed]
- Horiguchi A, Sumitomo M, Asakuma J, et al. Increased serum leptin levels and over expression of leptin receptors are associated with the invasion and progression of renal cell carcinoma. J Urol 2006;176:1631-5. [Crossref] [PubMed]
- Pinthus JH, Kleinmann N, Tisdale B, et al. Lower plasma adiponectin levels are associated with larger tumor size and metastasis in clear-cell carcinoma of the kidney. Eur Urol 2008;54:866-73. [Crossref] [PubMed]
- de Martino M, Leitner CV, Hofbauer SL, et al. Serum Adiponectin Predicts Cancer-specific Survival of Patients with Renal Cell Carcinoma. Eur Urol Focus 2016;2:197-203. [Crossref] [PubMed]
- Horiguchi A, Ito K, Sumitomo M, et al. Decreased serum adiponectin levels in patients with metastatic renal cell carcinoma. Jpn J Clin Oncol 2008;38:106-11. [Crossref] [PubMed]
- Tumminia A, Vinciguerra F, Parisi M, et al. Adipose Tissue, Obesity and Adiponectin: Role in Endocrine Cancer Risk. Int J Mol Sci 2019;20:2863. [Crossref] [PubMed]
- Parida S, Siddharth S, Sharma D. Adiponectin, Obesity, and Cancer: Clash of the Bigwigs in Health and Disease. Int J Mol Sci 2019;20:2519. [Crossref] [PubMed]
- Steinberg GR, Hardie DG. New insights into activation and function of the AMPK. Nat Rev Mol Cell Biol 2023;24:255-72. [Crossref] [PubMed]
- Tahergorabi Z, Lotfi H, Rezaei M, et al. Crosstalk between obesity and cancer: a role for adipokines. Arch Physiol Biochem 2024;130:155-68. [Crossref] [PubMed]
- Kim AY, Lee YS, Kim KH, et al. Adiponectin represses colon cancer cell proliferation via AdipoR1- and -R2-mediated AMPK activation. Mol Endocrinol 2010;24:1441-52. [Crossref] [PubMed]
- Taliaferro-Smith L, Nagalingam A, Zhong D, et al. LKB1 is required for adiponectin-mediated modulation of AMPK-S6K axis and inhibition of migration and invasion of breast cancer cells. Oncogene 2009;28:2621-33. [Crossref] [PubMed]
- Saxena NK, Sharma D. Metastasis suppression by adiponectin: LKB1 rises up to the challenge. Cell Adh Migr 2010;4:358-62. [Crossref] [PubMed]
- Di Zazzo E, Polito R, Bartollino S, et al. Adiponectin as Link Factor between Adipose Tissue and Cancer. Int J Mol Sci 2019;20:839. [Crossref] [PubMed]
- Sciacca L, Vella V, Frittitta L, et al. Long-acting insulin analogs and cancer. Nutr Metab Cardiovasc Dis 2018;28:436-43. [Crossref] [PubMed]
- Tadiotto MC, Corazza PRP, Menezes FJ Junior, et al. Lower adiponectin is associated with higher anthropometry and insulin resistance but not with low cardiorespiratory fitness in adolescents. J Endocrinol Invest 2024;47:307-14. [Crossref] [PubMed]
- Maeda N, Shimomura I, Kishida K, et al. Diet-induced insulin resistance in mice lacking adiponectin/ACRP30. Nat Med 2002;8:731-7. [Crossref] [PubMed]
- Park J, Morley TS, Kim M, et al. Obesity and cancer--mechanisms underlying tumour progression and recurrence. Nat Rev Endocrinol 2014;10:455-65. [Crossref] [PubMed]
- Kim JW, Kim JH, Lee YJ. The Role of Adipokines in Tumor Progression and Its Association with Obesity. Biomedicines 2024;12:97. [Crossref] [PubMed]
- Chen C, Zhao W, Lu X, et al. AUP1 regulates lipid metabolism and induces lipid accumulation to accelerate the progression of renal clear cell carcinoma. Cancer Sci 2022;113:2600-15. [Crossref] [PubMed]
- Miao D, Wang Q, Shi J, et al. N6-methyladenosine-modified DBT alleviates lipid accumulation and inhibits tumor progression in clear cell renal cell carcinoma through the ANXA2/YAP axis-regulated Hippo pathway. Cancer Commun (Lond) 2023;43:480-502. [Crossref] [PubMed]
- Xu H, Jin C, Guan Q. Causal Effects of Overall and Abdominal Obesity on Insulin Resistance and the Risk of Type 2 Diabetes Mellitus: A Two-Sample Mendelian Randomization Study. Front Genet 2020;11:603. [Crossref] [PubMed]
- Holmes MV, Lange LA, Palmer T, et al. Causal effects of body mass index on cardiometabolic traits and events: a Mendelian randomization analysis. Am J Hum Genet 2014;94:198-208. [Crossref] [PubMed]
- Johansson M, Carreras-Torres R, Scelo G, et al. The influence of obesity-related factors in the etiology of renal cell carcinoma-A mendelian randomization study. PLoS Med 2019;16:e1002724. [Crossref] [PubMed]
- Matyszewski A, Czarnecka A, Kawecki M, et al. Impaired glucose metabolism treatment and carcinogenesis. Oncol Lett 2015;10:589-94. [Crossref] [PubMed]
- Frystyk J. Free insulin-like growth factors -- measurements and relationships to growth hormone secretion and glucose homeostasis. Growth Horm IGF Res 2004;14:337-75. [Crossref] [PubMed]
- Zhang T, Niu X, Liao L, et al. The contributions of HIF-target genes to tumor growth in RCC. PLoS One 2013;8:e80544. [Crossref] [PubMed]
- Solarek W, Koper M, Lewicki S, et al. Insulin and insulin-like growth factors act as renal cell cancer intratumoral regulators. J Cell Commun Signal 2019;13:381-94. [Crossref] [PubMed]
- Takahashi M, Inoue T, Huang M, et al. Inverse relationship between insulin receptor expression and progression in renal cell carcinoma. Oncol Rep 2017;37:2929-41. [Crossref] [PubMed]
- Lin S, Chai Y, Zheng X, et al. The role of HIF in angiogenesis, lymphangiogenesis, and tumor microenvironment in urological cancers. Mol Biol Rep 2023;51:14. [Crossref] [PubMed]
- Seo JB, Riopel M, Cabrales P, et al. Knockdown of Ant2 Reduces Adipocyte Hypoxia And Improves Insulin Resistance in Obesity. Nat Metab 2019;1:86-97. [Crossref] [PubMed]
- Hoefflin R, Harlander S, Schäfer S, et al. HIF-1α and HIF-2α differently regulate tumour development and inflammation of clear cell renal cell carcinoma in mice. Nat Commun 2020;11:4111. [Crossref] [PubMed]
- Golijanin B, Malshy K, Khaleel S, et al. Evolution of the HIF targeted therapy in clear cell renal cell carcinoma. Cancer Treat Rev 2023;121:102645. [Crossref] [PubMed]
- Shen C, Beroukhim R, Schumacher SE, et al. Genetic and functional studies implicate HIF1α as a 14q kidney cancer suppressor gene. Cancer Discov 2011;1:222-35. [Crossref] [PubMed]
- Fernandes Q, Inchakalody VP, Bedhiafi T, et al. Chronic inflammation and cancer; the two sides of a coin. Life Sci 2024;338:122390. [Crossref] [PubMed]
- Cardillo MR, Ippoliti F. Interleukin-6, interleukin-10 and heat shock protein-90 expression in renal epithelial neoplasias and surrounding normal-appearing renal parenchyma. Int J Immunopathol Pharmacol 2007;20:37-46. [Crossref] [PubMed]
- Wang T, Lu R, Kapur P, et al. An Empirical Approach Leveraging Tumorgrafts to Dissect the Tumor Microenvironment in Renal Cell Carcinoma Identifies Missing Link to Prognostic Inflammatory Factors. Cancer Discov 2018;8:1142-55. [Crossref] [PubMed]
- Matsukawa T, Doi T, Obayashi K, et al. ANGPTL8 links inflammation and poor differentiation, which are characteristics of malignant renal cell carcinoma. Cancer Sci 2023;114:1410-22. [Crossref] [PubMed]
- Jin X, Qiu T, Li L, et al. Pathophysiology of obesity and its associated diseases. Acta Pharm Sin B 2023;13:2403-24. [Crossref] [PubMed]
- Liu BX, Sun W, Kong XQ. Perirenal Fat: A Unique Fat Pad and Potential Target for Cardiovascular Disease. Angiology 2019;70:584-93. [Crossref] [PubMed]
- Shah PH, Lyon TD, Lohse CM, et al. Prognostic evaluation of perinephric fat, renal sinus fat, and renal vein invasion for patients with pathological stage T3a clear-cell renal cell carcinoma. BJU Int 2019;123:270-6. [Crossref] [PubMed]
- Huang H, Chen S, Li W, et al. High perirenal fat thickness predicts a poor progression-free survival in patients with localized clear cell renal cell carcinoma. Urol Oncol 2018;36:157.e1-157.e6. [Crossref] [PubMed]
- Du Y, Yang W, Liu H, et al. Perirenal Fat as a New Independent Prognostic Factor in Patients With Surgically Treated Clear Cell Renal Cell Carcinoma. Clin Genitourin Cancer 2022;20:e75-80. [Crossref] [PubMed]
- Preza-Fernandes J, Passos P, Mendes-Ferreira M, et al. A hint for the obesity paradox and the link between obesity, perirenal adipose tissue and Renal Cell Carcinoma progression. Sci Rep 2022;12:19956. [Crossref] [PubMed]
- Wei G, Sun H, Dong K, et al. The thermogenic activity of adjacent adipocytes fuels the progression of ccRCC and compromises anti-tumor therapeutic efficacy. Cell Metab 2021;33:2021-2039.e8. [Crossref] [PubMed]
- Liu J, Zheng D, Qi P, et al. Retroperitoneal laparoscopic radical nephrectomy (RLRN) is associated with poor integrity of Gerota's fascia and perirenal fat: A prospective comparative study. Front Surg 2023;10:1114065. [Crossref] [PubMed]
- Nakanishi Y, Hirose K, Yasujima R, et al. Impact of perinephric fat volume and the Mayo Adhesive Probability score on time to clamping in robot-assisted partial nephrectomy. J Robot Surg 2023;17:1485-91. [Crossref] [PubMed]
- Campi R, Di Maida F, Lane BR, et al. Impact of surgical approach and resection technique on the risk of Trifecta Failure after partial nephrectomy for highly complex renal masses. Eur J Surg Oncol 2022;48:687-93. [Crossref] [PubMed]
- Campi R, Grosso AA, Lane BR, et al. Impact of Trifecta definition on rates and predictors of "successful" robotic partial nephrectomy for localized renal masses: results from the Surface-Intermediate-Base Margin Score International Consortium. Minerva Urol Nephrol 2022;74:186-93. [Crossref] [PubMed]
- Grosso AA, Di Maida F, Tellini R, et al. Robot-assisted partial nephrectomy with 3D preoperative surgical planning: video presentation of the florentine experience. Int Braz J Urol 2021;47:1272-3. [Crossref] [PubMed]