Exosomal AHSG in ovarian cancer ascites inhibits malignant progression of ovarian cancer by p53/FAK/Src signaling
Highlight box
Key findings
• A common feature of patients with advanced ovarian cancer (OC) is the accumulation of ascites in the abdominal cavity, where exosomes are present and can transmit messages. Our findings indicate that the exosome alpha-2 Heremans Schmid glycoprotein (AHSG) extracted from cancer cells negatively regulates OC cell motility, migration, and metastasis. The present study suggests that AHSG is a potential candidate for the treatment of OC.
What is known and what is new?
• Exosomes have been identified in OC ascites, and these exosomes have been demonstrated to promote the metastasis of OC.
• AHSG is down-regulated in ovarian tissue and ascites and has been demonstrated to inhibit the migration of OC cells. This provides a potential candidate for OC therapy.
What is the implication, and what should change now?
• Implications: the present study demonstrates that AHSG is expressed at lower levels in OC and correlates with prognosis. Furthermore, it provides an experimental rationale for AHSG to be a potential target for OC treatment.
• Actions needed: further studies are needed to elucidate the mechanisms in more detail and to validate these findings in the clinical setting, laying the foundation for the development of targeted therapies for OC.
Introduction
Ovarian cancer (OC) is one of the deadliest gynecological malignancies of the female reproductive system (1). The World Health Organization (WHO) classifies OC into three categories based on cell type: epithelial OC, ovarian mesenchymal cell carcinoma, and ovarian germ cell carcinoma (2). Of these, epithelial OC is the most common type in more than 90% of diagnosed ovarian malignancies (2). At the tissue level, epithelial OC can be divided into five main subtypes: clear cell, high-grade plasmacytoid, low-grade plasmacytoid, endometrioid, and mucinous (3). The mainstay of treatment for patients with advanced OC is tumor cytoreduction supplemented with platinum-based chemotherapy (4). However, despite this treatment, the overall survival of these patients remains low. Disease recurrence after multidrug resistance is the most important factor affecting their survival (5). Nevertheless, the primary cause of chemoresistance in OC is the elevated antioxidant capacity of OC cells. Consequently, the urgent need to identify new biomarkers and therapeutic targets to delay chemoresistance in OC is clear (6). OC has been thought to spread as tumor cells metastasize through the peritoneal fluid to the peritoneum and omentum, unlike most of the remaining malignancies that metastasize through the bloodstream (4). It is well known that the hallmark of OC is ascites and peritoneal fluid, which contains several components that can promote tumor metastasis as well as chemo-resistance, which can lead to morbidity and mortality in patients, and for which there is still a lack of research (7).
Exosomes are 40–200 nm extracellular vesicles containing cytophilic substances such as DNA, microRNA (miRNA), proteins, and lipids, and are widely found in various body fluids such as blood, ascites urine, lymph, bile, and urine (2). Compared with that of the ascites of nontumor patients, the concentration of exosomes in the ascites of malignant ascites is increased 3–4 times (8). Ascites-derived exosomes (ADEs) are considered to be associated with tumor burden, invasion and metastasis, and poor survival (9). According to recent studies, exosomes can facilitate cancer progression by mediating communication between tumor cells and surrounding cells. Malignant ADEs can promote OC metastasis in vitro and in vivo, which is based on epithelial-mesenchymal transformation (EMT) of OC cells (10). Malignant ADEs have been shown to stimulate peritoneal metastasis through transforming growth factor beta-1 (TGF-β1) induced mesenchymal transformation (11). These results suggest that ADEs play an important role in OC progression and are associated with poorer prognosis (12). Nonetheless, how OC cell-derived exosomal proteins promote OC metastasis needs to be further investigated.
Alpha-2 Heremans Schmid glycoprotein (AHSG), a negative acute-phase protein, is mainly produced by the liver and secreted into the blood. AHSG has been demonstrated to participate in various biological functions of the body, such as insulin resistance, calcification regulation, negative stress response and cancer progression (13). Under in vitro conditions, AHSG promotes cell adhesion and diffusion and increases aggression with the help of exosomes (14). AHSG plays different roles in different tumors. Previous reports have demonstrated that AHSG can promote the occurrence and progression of breast cancer, bladder cancer, and Lewis lung carcinoma (15-17). In contrast, AHSG, as a mimic of the TGF-β receptor, shows low expression in intestinal tumors and antagonizes intestinal tumorigenesis (18). However, the role of AHSG in the progression of OC has not been reported.
P53 (cellular tumor antigen p53) is a common mutated tumor suppressor gene in cancer that encodes the p53 protein (19). The p53 protein can be activated by different signaling pathways, such as DNA damage and hypoxia pathways when cells are stimulated by internal and external factors (20). Therefore, the p53 protein plays an important role in a variety of biological processes, such as apoptosis, cell cycle arrest, senescence and cell metabolism (21). The p53 protein has also been reported to be widely involved in the regulation of cancer metastatic pathways, such as autophagy, invasion, angiogenesis, EMT, and anoikis (22). The p53 protein plays a carcinogenic role and enhances metastasis in multiple cancers, including gynecological tumors (23,24). Focal adhesion kinase (FAK) is a tyrosine kinase that is highly expressed in a variety of tumors and plays a kinase-dependent role in promoting tumor invasion and metastasis. The expression of FAK is proportional to the prognosis of patients (25). FAK can combine with Src to form a complex and participate in cancer progression (25). P53 and FAK promoters are reported to have binding sites. In prostate cancer, it has been found that interfering with p53 expression can activate the FAK/Src (proto-oncogene tyrosine-protein kinase Src) pathway and promote cancer metastasis (26).
Previous studies have reported that by promoting the metastasis and invasion of cancer cells, AHSG may serve as a regulator of many malignant tumors (15-17,27), but whether OC cell (SKOV3 and HO8910 cells)-derived AHSG can be released into the tumor microenvironment in a paracrine manner and consequently influence tumor progression have not been adequately investigated. In the current study, we demonstrated that ADEs were associated with OC ascites invasion and metastasis. In addition, AHSG protein expression was downregulated in epithelial OC tissues and exosomes, which plays an inhibitory role in the progression of OC. Critically, exosomes transferred cancer-derived AHSG to OC cells and inhibited OC migration by regulating p53/FAK/Src signaling. The study demonstrated the mechanism by which exosomes mediate communication through AHSG and confirmed the role of cancer-derived AHSG in tumor invasion and metastasis. Our study may therefore advance our understanding of OC and lead to more effective treatments for patients with OC at high risk of metastasis. We present this article in accordance with the ARRIVE and MDAR reporting checklists (available at https://tcr.amegroups.com/article/view/10.21037/tcr-24-789/rc).
Methods
OC tissue specimens and ascites specimens
A protocol was prepared before the study without registration. Twenty-six OC ascites specimens and 20 benign disease ascites specimens were collected from patients who underwent surgery at Xuzhou Central Hospital from July 2021 to October 2022. This study was conducted in accordance with the Declaration of Helsinki (as revised in 2013). The study was approved by the Ethics Committee of Xuzhou Central Hospital (Approval number: XZXY-LK-20220908-075). Informed consent was obtained from all individual participants. No patients had received radiotherapy or chemotherapy before the surgery, and all were pathologically diagnosed with OC (the included and excluded specimens are shown in Appendix 1). For removal of impurities and cellular components, the fresh ascites were centrifuged at 3,000 × g for 15 min at 4 ℃. The remaining sample was stored at −80 ℃ for subsequent experiments. Detailed information for this study population is presented in Table S1.
Cell culture and cell transfection
The human OC cell lines SKOV3 (kindly provided by Cancer Institute of Xuzhou Medical University) and HO8910 (kindly provided by Cancer Institute of Xuzhou Medical University) were cultured in RPMI 1640 medium (KeyGEN BioTECH, KGL1501-500, China) containing 1% penicillin-streptomycin (Beyotime, ST488S, China) and 10% fetal bovine serum (FBS, Biochannel, BC-SE-FBS07-500ml, China). The incubator was set at 37 ℃ with 5% CO2. At a density of approximately 80–90% for OC cells, the cells were washed twice with phosphate buffer solution (PBS, Servicebio, G4202-500ML, China), and SKOV3 and HO8910 cells were harvested. Following the exclusion of exosomes from the serum, 0 cells were co-cultured with serum-free medium and 25 µg of exosomes or 25 µL of PBS was added to the medium. The cells were then cultured for 24 hours. After 24 hours of culture, SKOV3 and HO8910 cells were harvested for subsequent experiments.
For cell transfection, SKOV3 and HO8910 cells were inoculated in 24-well plates and subsequently transfected with a serum-free medium containing either the vector or AHSG-luc (Shanghai Gene Chemistry Co., Ltd., China) for 12 hours. The volume of lentivirus we used was based on the following formula: virus (µL) = cell number × multiplicity of infection/virus (stock solution) titer. After transfection, overexpressed cell lines were further selected with puromycin and verified by Western blots and quantitative real-time polymerase chain reaction (qRT-PCR). The transfection groups were as follows: SKOV3 (blank group), SKOV3‐NC (negative control group), SKOV3-AHSG (AHSG upregulated group), HO8910 (blank group), HO8910‐NC (negative control group), and HO8910-AHSG (AHSG upregulated group).
Isolation of exosomes
The classic ultracentrifugation method was used to extract and purify exosomes. Ascites was collected and centrifuged at 3,000 ×g for 15 minutes, 11,000 ×g for 30 minutes and 12,000 ×g for 30 minutes to remove impurities and cellular components. The supernatant was collected and filtered through 0.45 µm (Merck Millipore Darmstadt, SLHUR33RB, Germany) and 0.22 µm filters (Merck Millipore Darmstadt, SLGPR33RB) and then, the exosomes were centrifuged twice by ultracentrifugation at 110,000 ×g for 70 minutes and dissolved in PBS.
Exosomes were isolated from the medium by ultracentrifugation. SKOV3 and HO8910 cells were cultured in a serum-free medium for 24 hours before the supernatant was collected. The supernatant was passed through a 0.22 mm filter (Merck Millipore, Darmstadt, Germany) to filter dead cells. Then, the supernatant was centrifuged at 10,000 ×g for 30 minutes. The subsequent procedures were the same as those used to extract exosomes from ascites.
Transmission electron microscopy
After ultracentrifugation, the exosomes were dissolved in 200 µL of PBS, and 50 µL was pipetted from the solution and dropped on copper mesh. The copper mesh was transferred to 3% pentanediol for 5 minutes. After the samples were washed 10 times with deionized water, they were exposed to 40 µL of phosphotungstic acid solution at a concentration of 4% for 30 s and then dried at room temperature (RT) for 30 minutes. The morphology and size of exosomes were observed by transmission electron microscopy (Tecnai G2 Spirit Twin, FEI Company, USA).
Dynamic light scattering analysis (DLA)
A laser nanoparticle size/potentiometer instrument (380ZLS, PSS, USA) was used to detect the size and concentration information of exosomes. First, the standard products were used to calibrate the machine, and then, the sample was loaded after passing the test.
Exosome uptake assay
For exosome uptake experiments, exosomes were labeled with the lipophilic dye Dil (Beyotime, C1990-1ml) and incubated at 37 ℃ for 20 minutes. Then, the labeled exosomes were cocultured with SKOV3 and HO8910 cells for 24 h. The nuclei were dyed with DAPI (Beyotime, P0131-5ml) after washing with PBS. Finally, the cells were imaged under a fluorescence microscope (Olympus, Kyoto, Japan).
Wound-healing assay
For the wound-healing assay, SKOV3 and HO8910 cells were placed in a 6‐well plate (5×106 cells per well) and cultured in FBS until 90% confluence. Then, the wounds were scratched with a 200 µL pipette tip. Cells were cultured in a serum-free medium with or without 25 µg exosomes after washing exfoliated cells with PBS. The wound areas were observed at 0 and 24 h with a microscope (Olympus, Kyoto, Japan), and ImageJ software was used to analyze the data of the wound healing area.
Transwell assay
SKOV3 and HO8910 cells (1×105/200 µL) in FBS-free medium were added to the upper transwell chambers, and 600 µL of 10% FBS medium was added to the bottom wells. For exosomes, a serum-free medium with exosomes (25 µg) and PBS (25 µL) was added to the upper chamber. After incubation for 24 hours, the cells were fixed in 4% paraformaldehyde for 30 minutes, washed and stained with 0.1% crystal violet (Solarbio, G1062, China). Images were taken from five random fields (200× magnification), and the number of cells in each chamber was counted under a microscope (Olympus, Kyoto, Japan).
qRT-PCR
Total RNA was extracted from ovarian cells and tissues. Then, the RNA was converted to cDNA by HiScriptII Q RT SuperMix for qPCR (+gDNA wiper) (Vazyme, Nanjing, China). Relative mRNA expression levels were calculated using the 2−ΔΔCt method. Quantification of gene expression was performed on Light Cycle 96 software version 1.1 (Roche) with specific primers: AHSG forward, CCCAGGGCTGATTTATAGACAA; AHSG reverse, GTTCAAGGTGTGTTTGTATCCC; GAPDH forward, AAGAAGGTGGTGAAGCAGGC; GAPDH reverse, TCCACCACCCAGTTGCTGTA.
Western blot analysis
Total protein was extracted with radioimmunoprecipitation assay (RIPA, Beyotime, P0013B) lysis buffer. Protein concentration was measured by the BCA protein assay kit (Beyotime, P0010) following the manufacturer’s instructions. The same amount of protein sample was loaded on sodium dodecyl sulfate polyacrylamide gel electrophoresis (SDS-PAGE, Servicebio, G2018) gels, transferred to a polyvinylidene fluoride (PVDF, Millipore, MA, USA) membrane pretreated with methanol and blocked with 3% BSA (Solarbio, A8020) at RT for 1 h. The membranes were reacted with primary antibodies at 4 ℃ overnight. The primary and secondary antibodies utilized are presented in Table S2. After reacting with the corresponding secondary antibodies at RT for 1 h, the membranes were imaged by a dual-color infrared laser imaging system (Odyssey® CLX, USA).
Immunohistochemical (IHC) staining
Paraffin-embedded sections of OC tissue and normal ovarian tissue were provided by Xuzhou Central Hospital. The anti-AHSG antibody was incubated with OC tissue and normal ovarian tissue sections overnight at 4 ℃. Then, the cells were stained with secondary antibodies for 10 minutes at RT. Finally, the cells were counterstained with 10% Mayer hematoxylin (Solarbio, G1080).
Enzyme-linked immunosorbent assay (ELISA)
The ELISA kit purchased from Wuhan Xinqidi Biotech Co. (Wuhan, China) was used to measure the level of AHSG in ascites samples. All samples were diluted with diluent reagent (1:2) before analysis. Optical density (OD) was read at 450 nm.
To measure AHSG levels in exosomes from ascites, we purchased an ELISA kit from Duoscientific Biotechnology Co., Ltd. (Hangzhou, China). The procedure was performed according to the instructions, and finally, the OD value was read at 450 nm.
Human AHSG protein data analysis
The UALCAN database (http://ualcan.path.uab.edu/) was used to detect the expression of AHSG protein in OC. To determine the prognostic value of AHSG in OC patients, we used Kaplan-Meier plotter (http://kmplot.com/analysis/) to investigate the prognostic value of AHSG in the OC dataset.
Analysis of tumorigenicity in nude mice
All animal experiments in this assay were authorized by the Ethics Committee of Xuzhou Medical University (approval number: 202208S085) in compliance with the institutional guidelines for the care and use of animals. To investigate the function of exosomal AHSG protein, we injected BALB/c nude mice with 1×107 SKOV3 cells. After tumor formation, 18 tumor-bearing mice were randomly divided into 3 groups (n=6): control, EXO-NC, and EXO-AHSG. Then, 25 µg exosomes were injected into the center of the tumor every 3 days. The tumor volume (mm3) was calculated as (length × width2)/2. After 3 weeks, all mice were sacrificed, and tumor bodies were removed and photographed.
Statistical analysis
All data were processed using SPSS 22.0 software. ImageJ software was used to process the gray value of protein bands and the wound healing area, while PicCnt software was used to count the number of migratory cells. Differences between the two groups were tested by Student’s t-tests. Comparisons among multiple sets of data were performed using ANOVA. Overall survival (OS) and progression-free survival (PFS) survival curves were assessed by the Kaplan-Meier method. P values <0.05 were considered statistically significant.
Results
Exosomes found in the ascites of OC patients promote the metastasis of OC cells
Previous studies have shown that exosomes are highly enriched in malignant ascites of OC (9,28). Thus, we extracted exosomes from 26 OC patients and 20 benign ascites samples by ultracentrifugation. Transmission electron microscopy was used to observe the morphology of the exosomes, and the results showed that the exosomes presented a typical cup-shaped appearance (Figure 1A). DLS analysis showed that the exosome diameter pellets had diameters ranging from 132 to 216 nm (Figure 1B). All the above results were consistent with the typical characteristics of exosomes. Moreover, Western blot analysis showed positive expression of the exosome marker proteins CD9, CD63 and TSG101 in the extracted exosomes from ascites (Figure 1C). These results indicated that exosomes were successfully extracted from ascites by ultracentrifugation. We used Dil to label the exosomes, and then, the Dil-labeled exosomes were cocultured with SKOV3 and HO8910 cells for 24 hours. Subsequently, the effective uptake of exosomes by SKOV3 and HO8910 cells was observed under a fluorescence microscope. The results showed that SKOV3 and HO8910 cells could take up Dil-labeled exosomes from the medium (Figure 1D,1E).
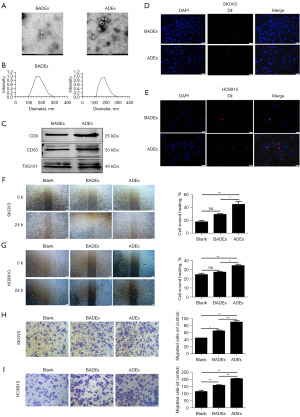
To investigate the effects of OC ADEs and benign ascites-derived exosomes (BADEs) on the biological behavior of OC cells, we cocultured SKOV3 and HO8910 cells with exosomes obtained by ultracentrifugation, and then, we detected the wound healing area at 0 and 24 h. The outcomes indicated that the migration of SKOV3 and HO8910 cells in the ADE group was significantly higher than that in the BADE group (Figure 1F,1G). Furthermore, the migration of SKOV3 and HO8910 cells treated with ADEs was significantly increased compared with that of SKOV3 and HO8910 cells treated with BADEs in migration experiments (Figure 1H,1I). The above results suggested that the ADEs had a more significant ability to promote OC cells migration than the BADEs in vitro. ADEs can promote peritoneal metastasis of OC by delivering bioactive molecules to alter the properties of recipient target cells (8). This finding is consistent with our experimental results.
AHSG expression is downregulated in OC tissues and OC ADEs
The literature has already proven that the expression of AHSG protein is downregulated in colorectal cancer and lung adenocarcinoma but upregulated in head and neck squamous cell carcinoma and pancreatic cancer (29). To investigate the expression of the AHSG protein in OC, we first detected the AHSG protein through the UALCAN database, and the results indicated that the AHSG protein was downregulated in OC tissues (Figure 2A). Moreover, we searched for the protein in the Kaplan-Meier plotter database and found that AHSG protein was positively correlated with the prognosis of OC patients (Figure 2B,2C). To verify this finding, we collected OC tissue samples and normal ovarian tissue samples for the following experiments. The results of PCR and western blotting showed that the mRNA level (Figure 2D) and protein level (Figure 2E) of AHSG in cancer tissues were significantly lower than those in noncancer tissues. IHC also showed that the AHSG protein was downregulated in OC tissues (Figure 2F). Our outcomes were consistent with the database results. All the results suggest that AHSG protein expression is downregulated in OC. Moreover, we collected the abdominal effusion of OC patients and benign disease groups for ELISA detection. The results showed that there was no significant difference in the expression of AHSG protein between malignant ascites and benign ascites (Figure 2G). We also extracted exosomes from the same batch of ascitic samples by ultracentrifugation for ELISA detection. The results showed that the AHSG protein content in ADEs was lower than that in BADEs (Figure 2H). All of these data showed that the AHSG protein was downregulated in tumor tissues and ADEs.
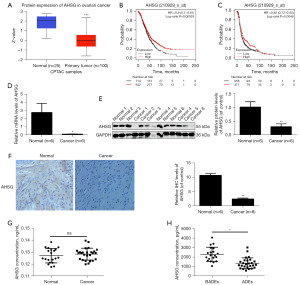
AHSG protein is transported by exosomes from OC cells
We used the AHSG overexpression lentivirus to construct AHSG-overexpressing SKOV3 cells (AHSG-SKOV3) and HO8910 cells (AHSG-HO8910) and verified them by PCR and Western blot experiments. The results showed that the mRNA and protein levels of the AHSG protein were significantly increased in the AHSG-SKOV3 group and the AHSG-HO8910 group compared with the NC-AHSG group (Figure 3A-3D). Furthermore, we extracted exosomes from the culture medium of SKOV3-NC, HO8910-NC, AHSG-SKOV3, and HO8910-AHSG cells by ultracentrifugation and then detected the expression of AHSG in exosomes by western blotting. The results showed that compared with that in the NC group, the expression level of AHSG in the exosomes extracted from the culture medium of the AHSG-SKOV3 group and the AHSG-HO8910 group was significantly increased (Figure 3E-3H). These results suggest that the AHSG protein can be secreted into exosomes by SKOV3 and HO8910 cells.
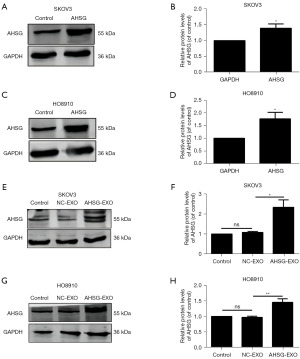
AHSG inhibits the migration of SKOV3 and HO8910 cells through the p53/FAK/Src signaling pathway
Tumor migration behavior is a crucial link in the malignant progression of OC. We first used the wound-healing assay to investigate the effect of AHSG on the migration of SKOV3 and HO8910 cells. As shown in Figure 4A,4B, the migration of the AHSG overexpression group was significantly decreased compared with that of the NC group. Furthermore, we used a migration experiment to verify the migration of SKOV3 and HO8910 cells. The results showed that the migration of the AHSG overexpression group was significantly decreased compared with that of the NC group (Figure 4C,4D).
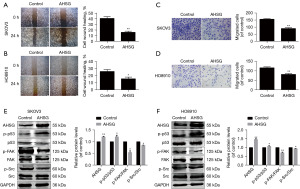
The p53/FAK/Src signaling pathway is closely related to proliferation, differentiation and metastasis (30). We used bioinformatics analysis to analyze the correlation between AHSG and p53, and the results showed that p53 and AHSG presented a positive correlation (Figure S1). Western blotting was used to detect the protein expression level of the p53/FAK/Src signaling pathway in SKOV3 and HO8910 cells when we upregulated AHSG expression. As shown in Figure 4E,4F, the expression level of p-p53 (activated p53) protein was significantly increased, while the expression levels of phosphorylated focal adhesion kinase (p-FAK) and p-Src were significantly decreased when AHSG was overexpressed. These results suggest that AHSG may inhibit the migration of SKOV3 and HO8910 cells by regulating the p53/FAK/Src signaling pathway.
Exosomal AHSG protein inhibits SKOV3 and HO8910 cell migration through the p53/FAK/Src signaling pathway
To examine the biological role of exosomal AHSG protein, we cocultured OC cells with exosomes extracted from the culture medium supernatant from the NC group (NC-EXO) and the AHSG overexpression group (AHSG-EXO). The wound healing assay showed that the migration of the AHSG-EXO group was significantly decreased compared with that of the control group and NC-EXO group (Figure 5A,5B). Furthermore, compared with that of the control group and NC-EXO group, the migration of SKOV3 and HO8910 cells treated with AHSG-EXOs was significantly reduced. All the data suggested that the exosomal AHSG protein could inhibit the migration of SKOV3 and HO8910 cells (Figure 5C,5D). These results suggest that the exosomal protein AHSG can significantly inhibit the migration of SKOV3 and HO8910 cells.
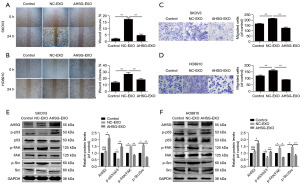
We cocultured SKOV3 and HO8910 cells with NC-EXOs and AHSG-EXOs for 24 h. Then, we detected the effects of exosomal AHSG protein on p53, FAK and Src proteins in SKOV3 and HO8910 cells by Western blotting. The expression level of p-p53 protein in the OC cells treated with AHSG-EXOs was significantly increased, while the expression levels of p-FAK and p-Src were significantly decreased. These results suggested that exosomal AHSG protein can be taken up by SKOV3 and HO8910 cells to promote the activation of p53 and inhibit the phosphorylation of FAK/Src (Figure 5E,5F). Therefore, we suggest that exosomal AHSG protein could inhibit the migration of SKOV3 and HO8910 cells through the p53/FAK/Src signaling pathway.
Exosomal AHSG protein inhibits the progression of OC cells in vivo
Next, we evaluated the effect of exosomal AHSG protein on OC development in vivo. Tumorigenicity analysis was performed in nude mice subcutaneously injected with SKOV3 cells, which were injected with PBS, NC-EXOs and AHSG-EXOs, and in the tumor center every 3 days. The results showed that the exosomal AHSG protein could significantly inhibit the growth of tumors in vivo, as demonstrated by tumor size, tumor weight, and tumor volume (Figure 6A-6D). These results indicated that exosomal AHSG protein could inhibit OC growth. In addition, we performed IHC analysis of p-p53, p-FAK and p-Src in tumor tissues and verified that exosomal AHSG significantly increased the expression of p-p53 and decreased the expression of p-FAK and p-Src in tumor tissues (Figure 6E). We also determined the expression of the metastatic markers vascular endothelial growth factor receptor (VEGFR), matrix metallopeptidase-2 (MMP2) and matrix metallopeptidase-9 (MMP9) in the tumor tissue, and the results showed that VEGFR, MMP2, and MMP9 were downregulated in the tumor tissues injected with AHSG-Exos (Figure 6F,6G). All the results suggested that exosomal AHSG could inhibit the tumor progression of OC in vivo.
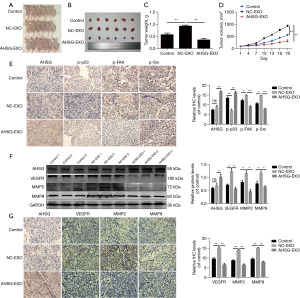
Discussion
OC is the most common cancer of the female reproductive system and has the highest mortality rate among gynecological malignancies in women (31). Metastasis is an important factor affecting the prognosis of OC patients. Fifty-eight percent of OCs have metastases at diagnosis, and the 5-year survival rate is only 30%, compared with the 5-year survival rate for locally nonspread OC, which is 93% (32). Therefore, it is important to explore the mechanism of OC metastasis to improve the survival rate and prognosis of OC patients.
Metastasis is a key link in tumor progression and the most common cause of cancer recurrence and death (33). Most women with epithelial OC present with peritoneal metastases accompanied by the accumulation of ascites at the time of diagnosis, which is a metastatic pattern specific to OC (34). Malignant ascites comprise a unique tumor microenvironment that is highly enriched in exosomes (28). Moreover, many studies have shown that exosomes secreted by tumor cells can regulate tumor growth, metastasis, angiogenesis and drug resistance (35-37). These molecules can also monitor the progression of the disease and be used as a diagnostic marker for the disease (38).
As a phosphorylated glycoprotein, AHSG participates in the normal physiological activities of the body and regulates various cancer processes (14). We found that the presence of exosomal AHSG contributes to the adhesion of cells to various extracellular matrices, and these exosomes are also responsible for premetastatic niche formation and mediate growth and motility (14). We also found that AHSG can transmit adhesion, motility, invasion, and growth signals in tumor cells via activated exosomes in astroglioblastoma (39). However, the ability of exosomal AHSG to affect OC migration has not been reported. Through ELISAs, we found that AHSG was enriched in ascitic exosomes and showed low expression in ADEs (Figure 2H). Our results show that AHSG can be transferred to receptor cells through exosomes and inhibits the migration of OC cells in vitro (Figure 5A-5D).
When we collected clinical ascites samples, we found that most of the ascites were from high-grade serous OC, a subtype of OC that often has TP53 mutations (40). This gene can encode the p53 protein, which is extensively involved in the progression of OC. We found a positive correlation between AHSG and p53 by searching the UALCAN database (Figure 4E), so we hypothesized that AHSG regulates OC progression through p53 signaling. It has been reported that the p53 protein can promote proliferation and metastasis through the FAK/Src pathway in lung adenocarcinoma. This molecule plays the same role in prostate cancer (35). FAK is a nonreceptor tyrosine kinase involved in signaling pathways related to proliferation, migration and angiogenesis (40). When activated, FAK can bind to Src family proteins to form the FAK-Src complex. The combination of the FAK-Src complex can initiate a variety of downstream pathways to promote cancer metastasis (41). In this study, we found that both exosomal AHSG and AHSG proteins inhibited the progression of OC through the p53/FAK/Src signaling pathway (Figures 4E,4F,5E,5F). This finding suggests that exosomal AHSG and AHSG proteins may act to inhibit OC migration through a similar mechanism. In vivo experiments also confirmed our conclusion (Figure 6A-6E). In addition, the expression levels of the metastatic markers VEGFR, MMP2 and MMP9 were determined, which further confirmed that exosomal AHSG could inhibit OC cell migration (Figure 6F,6G). However, these results only preliminarily suggest that exosomal AHSG can inhibit the progression of OC, which still needs to be verified by further experiments.
Conclusions
In conclusion, our findings indicate that AHSG expression levels were low in OC tissue samples. Accordingly, we postulate that it is associated with OC metastasis. Therefore, low levels of the exosomal protein AHSG were detected in ascites samples from OC patients. The exosomal AHSG protein was demonstrated to inhibit OC metastasis at the cellular and exosomal levels, respectively, through wound healing and permeabilization assays. With regard to the mechanism, it is proposed that exosomal protein AHSG exerts an inhibitory effect on OC cell metastasis via the p53/FAK/Src pathway. Furthermore, in vivo experiments demonstrated that exosomal protein AHSG impeded the metastasis of OC.
Acknowledgments
We thank all volunteers in this study for their cooperation and the support of gynecologists in Xuzhou Central Hospital.
Funding: This study was supported by
Footnote
Reporting Checklist: The authors have completed the ARRIVE and MDAR reporting checklists. Available at https://tcr.amegroups.com/article/view/10.21037/tcr-24-789/rc
Data Sharing Statement: Available at https://tcr.amegroups.com/article/view/10.21037/tcr-24-789/dss
Peer Review File: Available at https://tcr.amegroups.com/article/view/10.21037/tcr-24-789/prf
Conflicts of Interest: All authors have completed the ICMJE uniform disclosure form (available at https://tcr.amegroups.com/article/view/10.21037/tcr-24-789/coif). The authors have no conflicts of interest to declare.
Ethical Statement: The authors are accountable for all aspects of the work in ensuring that questions related to the accuracy or integrity of any part of the work are appropriately investigated and resolved. The clinical study was conducted according to the principles expressed in the Declaration of Helsinki (as revised in 2013) and was approved by the Research Ethics Committee of Xuzhou Central Hospital (Approval number: XZXY-LK-20220908-075). Informed consent was obtained from all individual participants. The animal study was approved by the Research Ethics Committee of Xuzhou Medical University (Approval number: 202208S085) in compliance with the institutional guidelines for the care and use of animals. The maximal tumor size/burden was not exceeded the permissible tolerance in institutional guidelines.
Open Access Statement: This is an Open Access article distributed in accordance with the Creative Commons Attribution-NonCommercial-NoDerivs 4.0 International License (CC BY-NC-ND 4.0), which permits the non-commercial replication and distribution of the article with the strict proviso that no changes or edits are made and the original work is properly cited (including links to both the formal publication through the relevant DOI and the license). See: https://creativecommons.org/licenses/by-nc-nd/4.0/.
References
- Shi X, Yu X, Wang J, et al. SIK2 promotes ovarian cancer cell motility and metastasis by phosphorylating MYLK. Mol Oncol 2022;16:2558-74. [Crossref] [PubMed]
- Pascual-Antón L, Cardeñes B, Sainz de la Cuesta R, et al. Mesothelial-to-Mesenchymal Transition and Exosomes in Peritoneal Metastasis of Ovarian Cancer. Int J Mol Sci 2021;22:11496. [Crossref] [PubMed]
- Prat J. Ovarian carcinomas: five distinct diseases with different origins, genetic alterations, and clinicopathological features. Virchows Arch 2012;460:237-49. [Crossref] [PubMed]
- Yousefi M, Dehghani S, Nosrati R, et al. Current insights into the metastasis of epithelial ovarian cancer - hopes and hurdles. Cell Oncol (Dordr) 2020;43:515-38. [Crossref] [PubMed]
- Fantone S, Piani F, Olivieri F, et al. Role of SLC7A11/xCT in Ovarian Cancer. Int J Mol Sci 2024;25:587. [Crossref] [PubMed]
- Tossetta G, Fantone S, Goteri G, et al. The Role of NQO1 in Ovarian Cancer. Int J Mol Sci 2023;24:7839. [Crossref] [PubMed]
- Ford CE, Werner B, Hacker NF, et al. The untapped potential of ascites in ovarian cancer research and treatment. Br J Cancer 2020;123:9-16. [Crossref] [PubMed]
- Vaksman O, Tropé C, Davidson B, et al. Exosome-derived miRNAs and ovarian carcinoma progression. Carcinogenesis 2014;35:2113-20. [Crossref] [PubMed]
- Cai J, Gong L, Li G, et al. Exosomes in ovarian cancer ascites promote epithelial-mesenchymal transition of ovarian cancer cells by delivery of miR-6780b-5p. Cell Death Dis 2021;12:210. [Crossref] [PubMed]
- Wei M, Yang T, Chen X, et al. Malignant ascites-derived exosomes promote proliferation and induce carcinoma-associated fibroblasts transition in peritoneal mesothelial cells. Oncotarget 2017;8:42262-71. [Crossref] [PubMed]
- Keller S, König AK, Marmé F, et al. Systemic presence and tumor-growth promoting effect of ovarian carcinoma released exosomes. Cancer Lett 2009;278:73-81. [Crossref] [PubMed]
- Icer MA, Yıldıran H. Effects of fetuin-A with diverse functions and multiple mechanisms on human health. Clin Biochem 2021;88:1-10. [Crossref] [PubMed]
- Watson K, Koumangoye R, Thompson P, et al. Fetuin-A triggers the secretion of a novel set of exosomes in detached tumor cells that mediate their adhesion and spreading. FEBS Lett 2012;586:3458-63. [Crossref] [PubMed]
- Nangami GN, Sakwe AM, Izban MG, et al. Fetuin-A (alpha 2HS glycoprotein) modulates growth, motility, invasion, and senescence in high-grade astrocytomas. Cancer Med 2016;5:3532-43. [Crossref] [PubMed]
- Dong Y, Ding D, Gu J, et al. Alpha-2 Heremans Schmid Glycoprotein (AHSG) promotes the proliferation of bladder cancer cells by regulating the TGF-β signalling pathway. Bioengineered 2022;13:14282-98. [Crossref] [PubMed]
- Kundranda MN, Henderson M, Carter KJ, et al. The serum glycoprotein fetuin-A promotes Lewis lung carcinoma tumorigenesis via adhesive-dependent and adhesive-independent mechanisms. Cancer Res 2005;65:499-506. [Crossref] [PubMed]
- Swallow CJ, Partridge EA, Macmillan JC, et al. alpha2HS-glycoprotein, an antagonist of transforming growth factor beta in vivo, inhibits intestinal tumor progression. Cancer Res 2004;64:6402-9. [Crossref] [PubMed]
- Ramraj SK, Elayapillai SP, Pelikan RC, et al. Novel ovarian cancer maintenance therapy targeted at mortalin and mutant p53. Int J Cancer 2020;147:1086-97. [Crossref] [PubMed]
- Nakamura M, Obata T, Daikoku T, et al. The Association and Significance of p53 in Gynecologic Cancers: The Potential of Targeted Therapy. Int J Mol Sci 2019;20:5482. [Crossref] [PubMed]
- Zhang C, Liu J, Xu D, et al. Gain-of-function mutant p53 in cancer progression and therapy. J Mol Cell Biol 2020;12:674-87. [Crossref] [PubMed]
- Babaei G, Aliarab A, Asghari Vostakolaei M, et al. Crosslink between p53 and metastasis: focus on epithelial-mesenchymal transition, cancer stem cell, angiogenesis, autophagy, and anoikis. Mol Biol Rep 2021;48:7545-57. [Crossref] [PubMed]
- Tang Q, Su Z, Gu W, et al. Mutant p53 on the Path to Metastasis. Trends Cancer 2020;6:62-73. [Crossref] [PubMed]
- Roszkowska KA, Gizinski S, Sady M, et al. Gain-of-Function Mutations in p53 in Cancer Invasiveness and Metastasis. Int J Mol Sci 2020;21:1334. [Crossref] [PubMed]
- Zhou J, Yi Q, Tang L. The roles of nuclear focal adhesion kinase (FAK) on Cancer: a focused review. J Exp Clin Cancer Res 2019;38:250. [Crossref] [PubMed]
- Wan J, Zhang J, Zhang J. Expression of p53 and its mechanism in prostate cancer. Oncol Lett 2018;16:378-82. [Crossref] [PubMed]
- Ochieng J, Nangami G, Sakwe A, et al. Impact of Fetuin-A (AHSG) on Tumor Progression and Type 2 Diabetes. Int J Mol Sci 2018;19:2211. [Crossref] [PubMed]
- Guillory B, Sakwe AM, Saria M, et al. Lack of fetuin-A (alpha2-HS-glycoprotein) reduces mammary tumor incidence and prolongs tumor latency via the transforming growth factor-beta signaling pathway in a mouse model of breast cancer. Am J Pathol 2010;177:2635-44. [Crossref] [PubMed]
- Nafar S, Nouri N, Alipour M, et al. Exosome as a target for cancer treatment. J Investig Med 2022;70:1212-8. [Crossref] [PubMed]
- Golubovskaya VM, Cance WG. Focal adhesion kinase and p53 signaling in cancer cells. Int Rev Cytol 2007;263:103-53. [Crossref] [PubMed]
- Tian W, Lei N, Zhou J, et al. Extracellular vesicles in ovarian cancer chemoresistance, metastasis, and immune evasion. Cell Death Dis 2022;13:64. [Crossref] [PubMed]
- Siegel RL, Miller KD, Fuchs HE, et al. Cancer Statistics, 2021. CA Cancer J Clin 2021;71:7-33. [Crossref] [PubMed]
- Jafari A, Babajani A, Abdollahpour-Alitappeh M, et al. Exosomes and cancer: from molecular mechanisms to clinical applications. Med Oncol 2021;38:45. [Crossref] [PubMed]
- Rakina M, Kazakova A, Villert A, et al. Spheroid Formation and Peritoneal Metastasis in Ovarian Cancer: The Role of Stromal and Immune Components. Int J Mol Sci 2022;23:6215. [Crossref] [PubMed]
- Uno K, Iyoshi S, Yoshihara M, et al. Metastatic Voyage of Ovarian Cancer Cells in Ascites with the Assistance of Various Cellular Components. Int J Mol Sci 2022;23:4383. [Crossref] [PubMed]
- Zhang Y, Bi J, Huang J, et al. Exosome: A Review of Its Classification, Isolation Techniques, Storage, Diagnostic and Targeted Therapy Applications. Int J Nanomedicine 2020;15:6917-34. [Crossref] [PubMed]
- Hyung S, Ko J, Heo YJ, et al. Patient-derived exosomes facilitate therapeutic targeting of oncogenic MET in advanced gastric cancer. Sci Adv 2023;9:eadk1098. [Crossref] [PubMed]
- Liu H, Deng S, Yao X, et al. Ascites exosomal lncRNA PLADE enhances platinum sensitivity by inducing R-loops in ovarian cancer. Oncogene 2024;43:714-28. [Crossref] [PubMed]
- Dabrowska AM, Tarach JS, Wojtysiak-Duma B, et al. Fetuin-A (AHSG) and its usefulness in clinical practice. Review of the literature. Biomed Pap Med Fac Univ Palacky Olomouc Czech Repub 2015;159:352-9. [Crossref] [PubMed]
- Silwal-Pandit L, Langerød A, Børresen-Dale AL. TP53 Mutations in Breast and Ovarian Cancer. Cold Spring Harb Perspect Med 2017;7:a026252. [Crossref] [PubMed]
- Juárez-Cruz JC, Zuñiga-Eulogio MD, Olea-Flores M, et al. Leptin induces cell migration and invasion in a FAK-Src-dependent manner in breast cancer cells. Endocr Connect 2019;8:1539-52. [Crossref] [PubMed]
- Dong C, Li X, Yang J, et al. PPFIBP1 induces glioma cell migration and invasion through FAK/Src/JNK signaling pathway. Cell Death Dis 2021;12:827. [Crossref] [PubMed]